ABSTRACT
Background
This study aimed to develop and characterize quatsomes as a novel nanocarrier system for the delivery of retinoic acid in keloid treatment.
Materials and Methods
Three quatsomes formulations (F1, F2 and F3) with varying surfactant/cholesterol ratios were prepared and evaluated for their physicochemical properties. The formulations are characterized for morphology, particle size, zeta potential, polydispersity index and entrapment efficiency.
Results
Based on the results, F3 was selected as the optimal formulation for further investigation. F3 exhibited spherical morphology, mean particle size of 104.5 nm, zeta potential of -41.9 mV, polydispersity index of 0.02 and entrapment efficiency of 85.2%. In vitro release studies of F3 were conducted over 600 hr, revealing a sustained release profile of retinoic acid. The release kinetics best fit in Korsmeyer-Peppas, indicating the mechanism of diffusion-controlled release. The developed quatsomes formulation demonstrated promising characteristics for retinoic acid delivery, including high drug loading capacity, uniform size distribution and sustained release properties.
Conclusion
These findings suggest that quatsomes may serve as an effective nanocarrier system for retinoic acid in keloid treatment, potentially improving drug stability, enhancing skin penetration and reducing side effects associated with conventional formulations.
INTRODUCTION
Quatsomes, also known as Quaternary ammonium compound-based vesicles, are nanovesicles that are self-assembled and are produced by mixing a cationic surfactant, a fatty acid and cholesterol using a combination of these three components.1 The self-assembly of quaternary ammonium surfactants with sterols like cholesterol results in the formation of quatsomes, which are hydrodynamically stable vesicular structures. They are able to maintain their morphologies despite changes in temperature or dilution, making these colloidal structures extraordinarily durable over extended periods of time. In terms of size, lamellarity and membrane supramolecular architecture, quatsomes exhibit a remarkable degree of consistent homogeneity. What gives quatsomes their characteristic structure is the synergy that exists between sterol and quaternary ammonium surfactant. In quatsomes, the sterol is situated within the bilayer itself, in contrast to the traditional vesicle-forming systems, in which both types of molecules have head groups at the interface between the water and the hydrocarbon. They exhibit high stability; biocompatibility and can encapsulate both hydrophilic and hydrophobic molecules, making them ideal for drug delivery, bioimaging and nanomedicine applications. Their advantages include enhanced drug solubility, controlled and sustained release, improved stability of sensitive drugs and targeted drug delivery that reduces toxicity.2 Retinoic acid, an active form of vitamin A, is commonly used in skincare and dermatology to treat conditions like acne, psoriasis and keloid scars. In the treatment of keloids, retinoic acid helps reduce chronic inflammation and excess collagen production, shrinking the size of keloids.3 By activating nuclear receptors (RAR and RXR), retinoic acid regulates cell differentiation, proliferation and apoptosis. Clinically, a 0.05% tretinoin cream has shown to reduce keloid size by around 20%. Additionally, retinoic acid improves skin texture, reduces UV-induced damage and is effective in preventing hypertrophic scars.4 Retinoic acid is well-known for its therapeutic effectiveness in skin disorders including acne, hyperpigmentation and photoaging. The integration of lipid-based nanocarriers, such as quatsomes (lipid vesicles stabilized by surfactants, present significant opportunities for improved skin penetration, regulated release and diminished irritation. Recent research has investigated lipid-based vesicular systems such as liposomes, niosomes and transferosomes for the administration of retinoic acid. Nonetheless, the potential of quatsomes for this application remains comparatively under investigated. Therefore, this research aims to develop most stable lipid carrier for topical delivery of Retinoic acid.
MATERIALS AND METHODS
Retinoic acid was purchased from Sigma-Aldrich Chemicals Private Limited, Bangalore, India. Cetyl Trimethyl Ammonium Bromide (CTAB) and Polyvinyl Alcohol (PVA) were purchased from LOBA Chemie Pvt. Ltd., Maharashtra, India. Cholesterol was purchased from AVRA synthesis Pvt. Ltd., Hyderabad, India. All the solvent and reagents were used in this research was analytical grade.
Solubility Testing
To test the solubility of retinoic acid in various solvents, a tiny amount was weighed and added to separate test tubes, each holding a fixed volume of one of the solvents (water, ethanol, acetone, ethyl acetate, or benzene). Surfactants and stabilizers such as CTAB, PVA and cholesterol were also tested for their impact on solubility. Every mixture was thoroughly mixed for 30 min to an hour at room temperature and observations were taken to see the solubility of different compounds in various solvents.5
Compatibility Studies
The compatibility of retinoic acid and cholesterol was studied using FTIR spectroscopy. The dry powder sample was combined with 100 mg of potassium bromide powder and it was compacted into a disc by applying a pressure of 5 tons for 2 min in a hydraulic press. The pellet was positioned in the sample cell and the spectra were studied in the range of 4000-400 cm-1. The spectral peaks were identified using integrated FTIR software.
Preparation of Retinoic Acid-Loaded Quatsomes
Dissolved 100 mg of Cetyl-Trimethyl Ammonium Bromide (CTAB) in 5 mL of ethanol. To this solution, added 0.1 mg of retinoic acid and mixed thoroughly to ensure complete dissolution. Dissolved 1 mg of Polyvinyl Alcohol (PVA) in 100 mL of deionized water. Ensure that the PVA is completely dissolved by stirring the solution gently. Using a homogenizer, gradually added the organic solution to the PVA aqueous solution dropwise. Stirred continuously during this process to promote the formation of the quatsomes. Allowed the solution to rest until the foam has completely dissipated. This resting period was essential for stabilizing the emulsion.6
Evaluation of Retinoic Acid Loaded Quatsomes
Vesicular Morphology
The surface morphology of quatsomes was scanned by using Transmission Electron Microscopy (TEM). A droplet of quatsome was carbon-coated to form a thin film on the grid and it was stained with 1% Phosphotungstic Acid (PTA) followed by air dry and samples were examined using a TEM (Hitachi 3000 N, JEOL, Japan) chamber. The quatsome picture was captured at 20 kV with a chamber pressure of 0.6 mmHg.
Vesicle size and zeta potential
The size, zeta potential and Polydispersity Index (PDI) were assessed using Zetasizer (Malvern Instruments, UK). A sufficient sample was dispersed in distilled water and measured all the parameters at 25°C. The parameters were measured based on the intensity of light scattered and conductivity of quatsome. All parameters were assessed in triplicate (n=3).
Entrapment Efficiency (EE %)
The quatsomes loaded drug was assessed to evaluate the entrapment efficiency. About 2 mL of quatsome was solubilized in 5 mL of DCM and centrifugation the suspension at 10,000 rpm for 15 min. The filter the supernatant liquid using a membrane filter and the drug concentration was analyzed using a UV-vis spectrophotometer at 350 nm.7 The EE was computed utilizing the subsequent formula.
W1 represents the weight of loaded drug, while W2 denotes the overall weight of drugs utilized.
In vitro Drug Release
The retinoic acid-loaded quatsomes was taken in a dialysis tube and donor chamber was safely clamped using strings. About 2.0 mL of sample was collected at predefined intervals upto 24 hr and the fresh solvent replaced to maintain sink conditions.7 The amount drug released was measured by UV spectroscopy at a wavelength of 350 nm. Various kinetic models were employed to examine the in vitro release data.
RESULTS
Solubility Testing
Retinoic Acid showed poor solubility in water but had high solubility in ethanol and benzene, indicating its preference for less polar solvents. The moderate solubility in acetone and ethyl acetate suggests some degree of compatibility with these solvents, likely due to their intermediate polarity. Cholesterol exhibited similar solubility characteristics as Retinoic Acid, with poor solubility in water and high solubility in ethanol and ethyl acetate.
Compatibility Studies
The Retinoic acid spectrum was reveals important functional groups that are crucial to explain its compatibility with Cholesterol and CTAB (Figure 1). The distinct peaks and its functional groups observed in the spectrum are as follows: 1690 cm-1 (-COOH group): This peak shows the stretching vibration of the carbonyl moiety in the carboxylic acid of Retinoic Acid. 1600 cm-1 (C=C, Alkenes): This peak corresponds to the stretching vibrations of the carbon-carbon double bond (C=C) in alkenes. At 3049 cm-1 (C-H, Alkenes), C-H stretching vibration associated with alkenes is observed at this peak. The similarity in functional groups between Retinoic Acid and Cholesterol (which also contains alkenes) could promote favorable intermolecular interactions. However, the effect on compatibility with CTAB, a surfactant, would depend more on the interplay of hydrophilic and hydrophobic domains rather than direct interaction with alkenes. At 963 cm-1 (Unsubstituted trans Double Bonds): This peak indicates the presence of unsubstituted trans double bonds, a feature that is characteristic of certain unsaturated lipids and acids. At 1254 cm-1 (Epoxy Rings) the presence of epoxy rings is indicated by this peak, which can be involved in ring-opening reactions or interactions with nucleophilic sites on other molecules. At 1175 cm-1 (Furan Structure Presence) the peak at 1175 cm-1 suggests the presence of a furan structure within Retinoic Acid.
Preparation of Retinoic Acid Loaded Quatsomes
By adjusting the amounts of CTAB, cholesterol and retinoic acid in the quatsome formulations, three distinct variants (F1, F2 and F3) were developed. The primary goal was to optimize the formulation for effective encapsulation and release of retinoic acid.
According to morphological analysis, the formulations F1 and F2 had noticeably less globules. Because there were insufficient globules in both formulations, it is likely that the specific ratios of cholesterol, retinoic acid and CTAB. Conversely, F3 formulation possessed the greatest quantity of globules and it’s achieved by particular mixture of CTAB, cholesterol and retinoic acid in quatsome production. The globules in F3 indicates enhanced self-assembly and stability, essential for effective drug delivery systems.
Morphology
Formulations F1 and F2 demonstrated a reduced quantity of spherical globules, signifying that these formulations yielded fewer particles with analogous morphology. F3 was selected for further tests, including in vitro release analysis, due to its superior globule formation (Figure 2).
Particle Size And Poly Dispersibility Index
Globule size was only reported for F3, with a measurement of 104.5 nm (Figure 3). This size falls within the nanometer range, which is typical for quatsomes and suggests good potential for applications in drug delivery, where smaller particle sizes can improve bioavailability and cellular uptake. The PDI for F3 was 0.027, indicating a highly uniform particle size distribution.
Zeta Potential
The zeta potential of F3 was recorded at -41.9 mV, which indicates a stable formulation (Figure 3). Zeta potential is a critical factor in predicting the stability of colloidal dispersions; values greater than ±30 mV typically suggest good stability due to sufficient repulsive forces between particles to prevent aggregation. The absence of zeta potential data for F1 and F2 again points to the possibility that these formulations were either not stable or not fully characterized.
Entrapment Efficiency
The encapsulation efficiency for F3 was 85.2%, signifying that a substantial proportion of the active ingredient was effectively contained within the quatsomes.
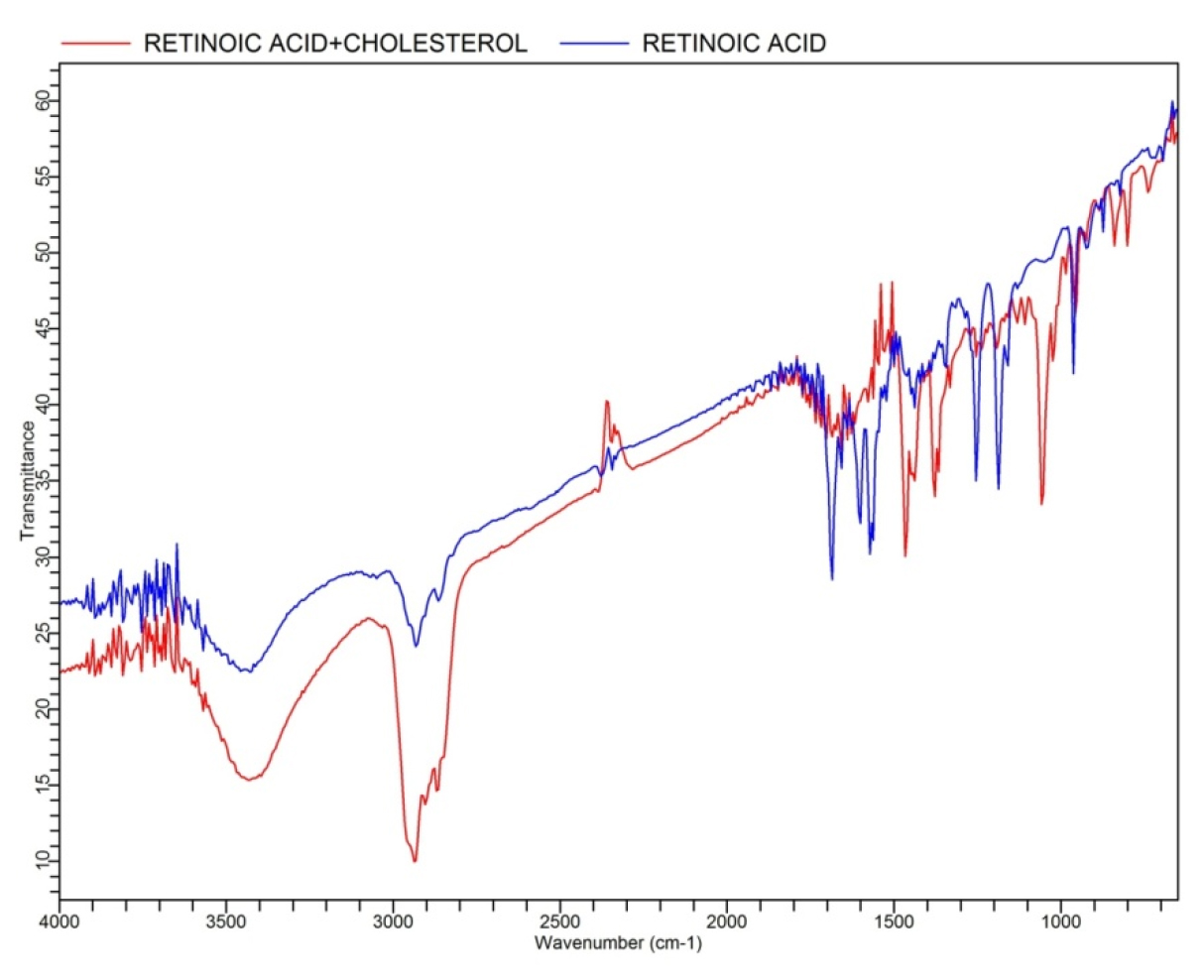
Figure 1:
FTIR spectrum for Retinoic acid+cholesterol.
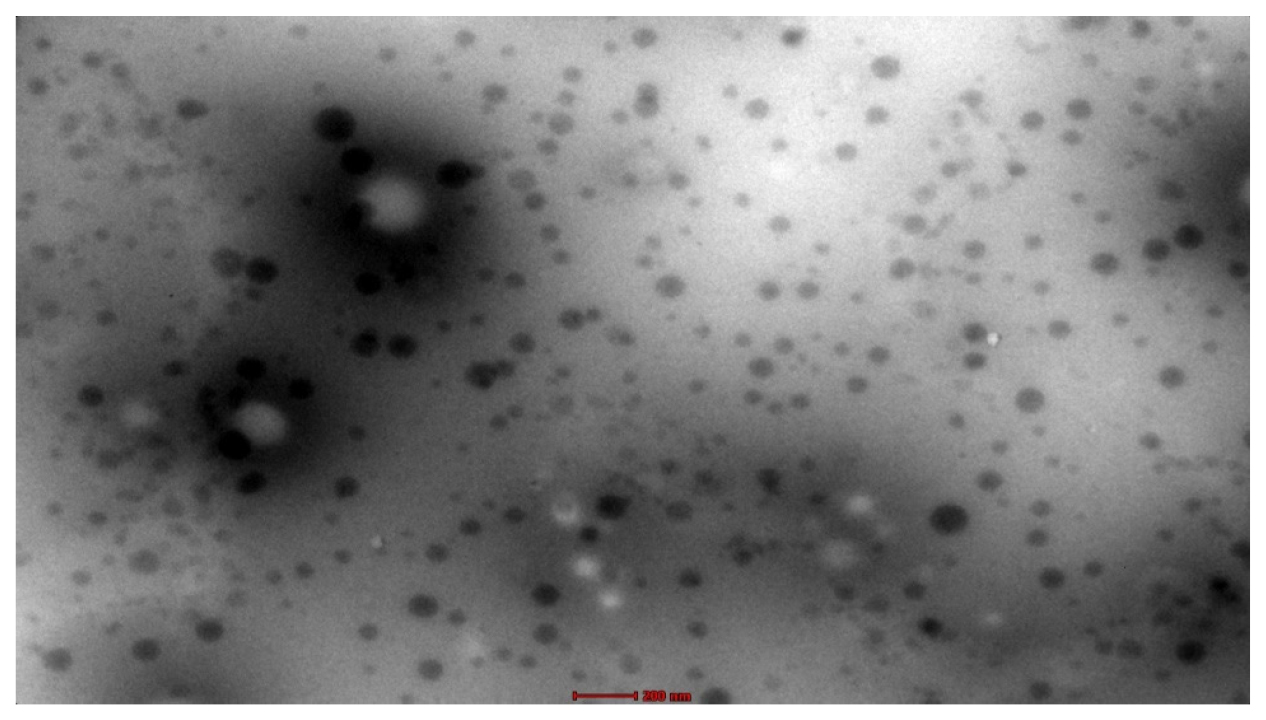
Figure 2:
Transmission Electron microscope of globules in F3.
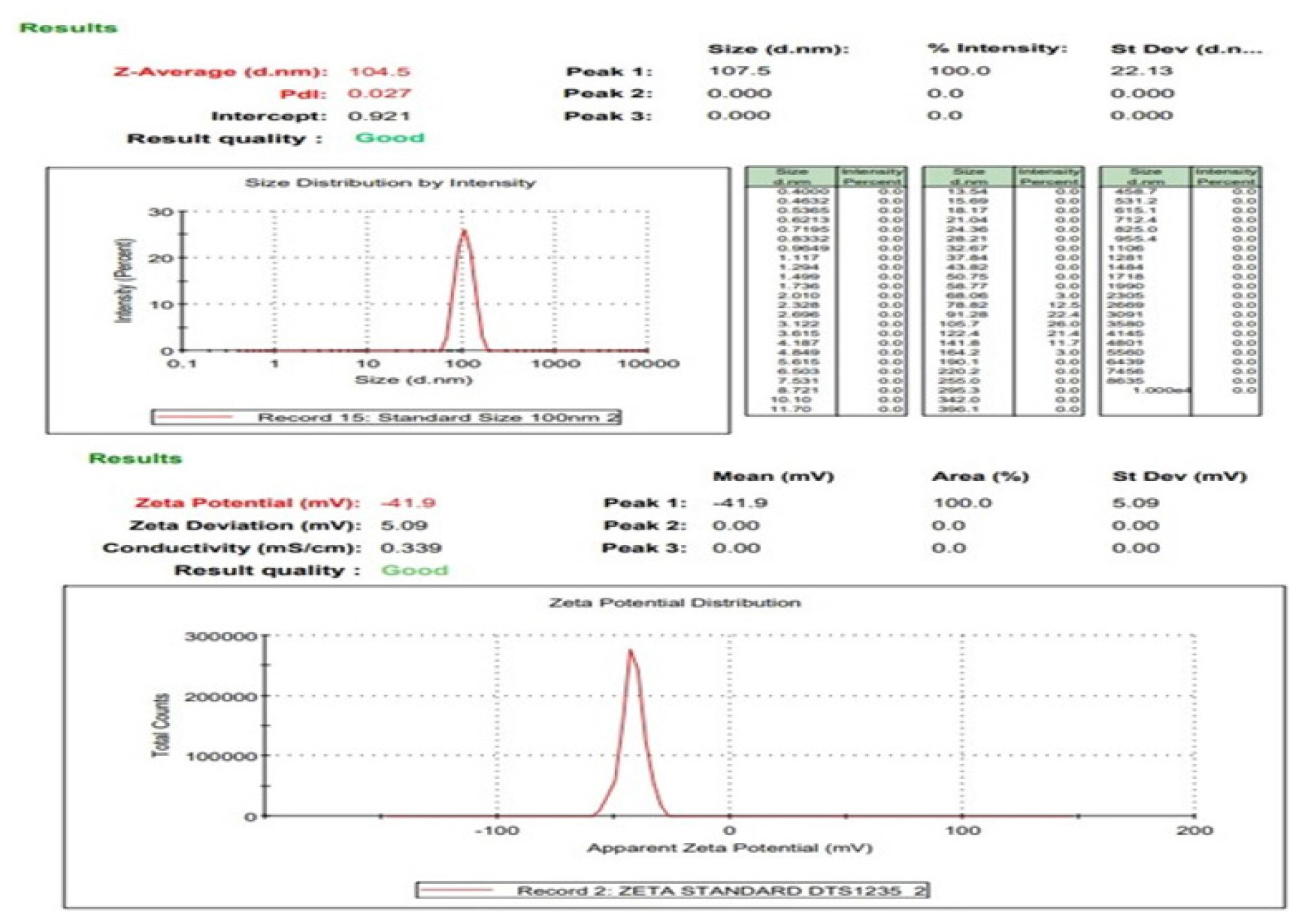
Figure 3:
Particle size and zeta potential of quatsome (F3).
In vitro Drug Release Studies
The in vitro drug release profile of the F3 formulation was monitored over a period of 600 hr and the cumulative percentage of drug release at various time intervals is as follows:
The in vitro drug release profile of the F3 formulation was observed for 600 hr, with the cumulative percentage of drug release recorded at various time intervals as follows (Figure 4).
The in vitro release profile of the F3 formulation demonstrates a sustained and regulated drug release over 600 hr, with a cumulative drug release of 90.45%. The release pattern illustrates the capability of the F3 quatsome formulation for sustained distribution, beneficial for therapeutic applications necessitating extended release.
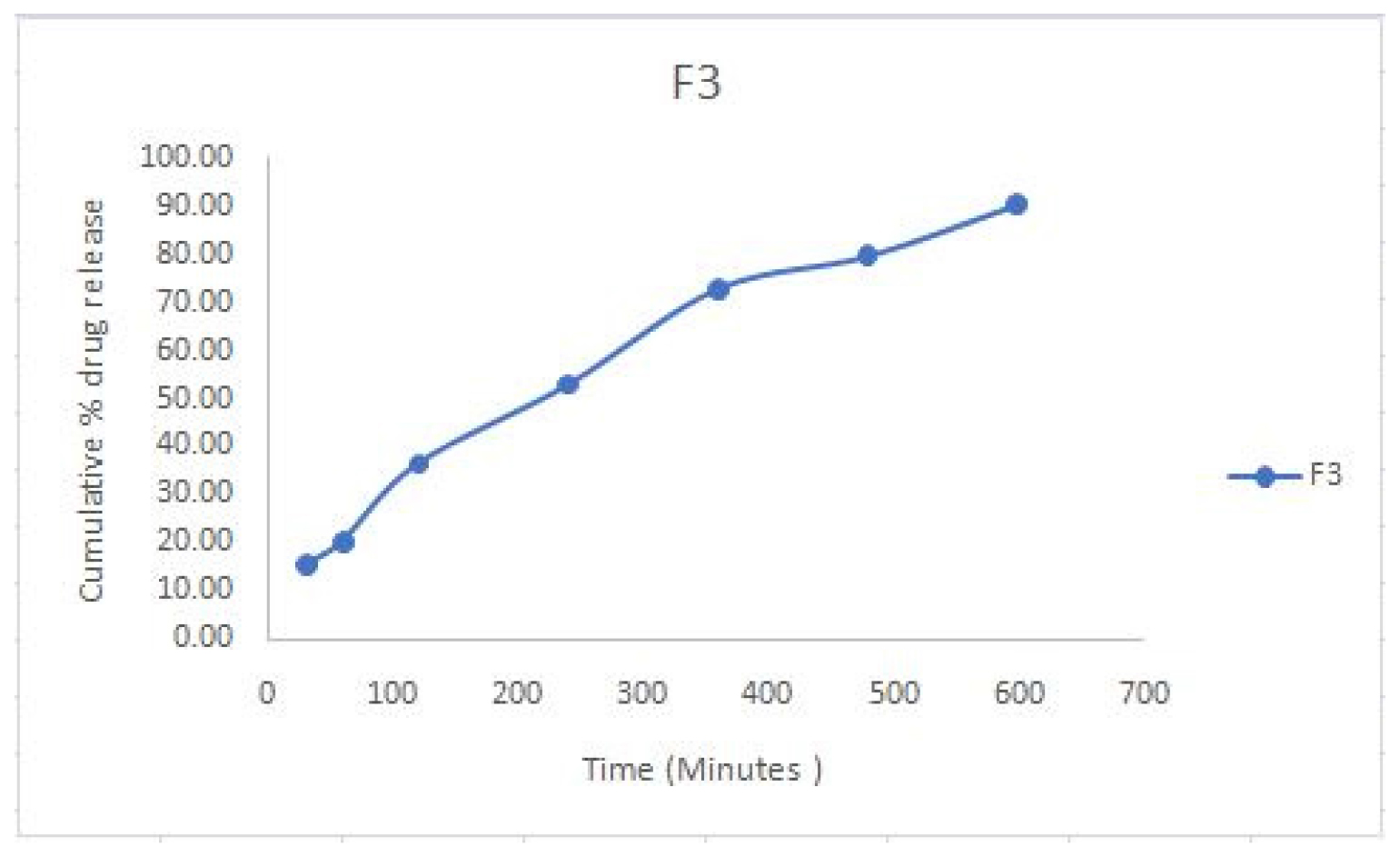
Figure 4:
In vitro release studies, Samples were analysed in triplicate (Mean±SD) and n=3.
Release of Kinetics
The release data has been used for in vitro kinetic examined by various kinetic equations, including zero-order, first-order, Higuchi and Korsmeyer-Peppas models. The release constant was determined by examining the slope of in vitro release graph and the regression coefficient (r2) was calculated (Figure 5). The first-order equation was the best appropriate model for explaining the in vitro release kinetics of quatsomal preparations (formulation F3). The findings of this investigation showed that the release rate is not much depended on drug concentration. Further F3 formulation followed Higuchi’s model which characterizes the drug release process as diffusion mechanism. The Korsmeyer-Peppas model was used to examine the drug release behavior, revealing that the characteristics of Fickian anomalous diffusion.13 The ‘n’ values utilized in the model varied from 0.2 to 0.5.
DISCUSSION
The Retinoic Acid, Cholesterol’s solubility in benzene was moderate, reflecting its partial affinity for non-polar solvents. CTAB demonstrated a strong preference for water, with high solubility and significantly lower solubility in all other solvents, indicating its polar nature.8 Its poor solubility in ethyl acetate and benzene suggests that it is incompatible with non-polar environments. PVA was highly soluble in water but showed reduced solubility in ethanol and poor solubility in acetone, ethyl acetate and benzene. This behavior is consistent with PVA’s hydrophilic properties, which limit its solubility in non-polar solvents.
Overall, the solubility patterns reflect the molecular structures and polarities of the compounds, with polar compounds showing higher solubility in polar solvents like water and non-polar or less polar compounds showing better solubility in non-polar solvents like benzene. These results can guide the selection of appropriate solvents for dissolving these compounds in various applications.9
The existence of this group is notable as it signifies substantial hydrogen bonding potential, which may affect interactions with both cholesterol and CTAB. Cholesterol, with a hydroxyl group, may establish hydrogen bonds with the carboxylic acid group of Retinoic Acid, indicating favorable compatibility between these 2 molecules. Alkenes are prevalent in the composition of unsaturated substances such as Retinoic Acid. The existence of this peak suggests possible π-π interactions with the unsaturated regions of Cholesterol, hence reinforcing the compatibility between Retinoic Acid and Cholesterol.10
The trans configuration may contribute to the rigidity and planarity of Retinoic Acid, affecting how it aligns or interacts with Cholesterol’s more flexible structure. CTAB may interact differently with this structure, possibly favoring interactions with other functional groups rather than the trans double bonds. This functional group might react with or interact with the hydroxyl group of Cholesterol. With CTAB, the epoxy rings may engage in weaker van der Waals interactions, given CTAB’s surfactant nature, which prefers ionic or polar interactions. Furan rings, being aromatic, might contribute to π-π stacking interactions, particularly with Cholesterol, which also has a planar aromatic-like structure in its sterol nucleus. However, furan interactions with CTAB would be minimal due to the lack of complementary structures in CTAB. The combination of these interactions suggests that Retinoic Acid is likely more compatible with Cholesterol than with CTAB.
The selection of F3 was predicated on its capacity to generate a more uniform and prolific population of quatsomes, anticipated to improve drug encapsulation efficacy and controlled release characteristics. This selection emphasizes the necessity of optimizing quatsome composition to get favorable physicochemical properties, which are crucial for their efficacy as drug carriers.9 The successful production of quatsomes in F3 establishes a promising basis for further research, intending to assess its efficacy in delivering retinoic acid. Conversely, F3 exhibited an increased quantity of spherical globules, indicating a more prolific generation of globules, which may be beneficial for specific applications requiring a greater globule density.9 This high efficiency is advantageous for therapeutic applications, guaranteeing that a substantial quantity of the medicine reaches the target region.11 The absence of encapsulation efficiency data for F1 and F2 indicates that these formulations were either less effective in encapsulating the active component or that the data was not gathered.
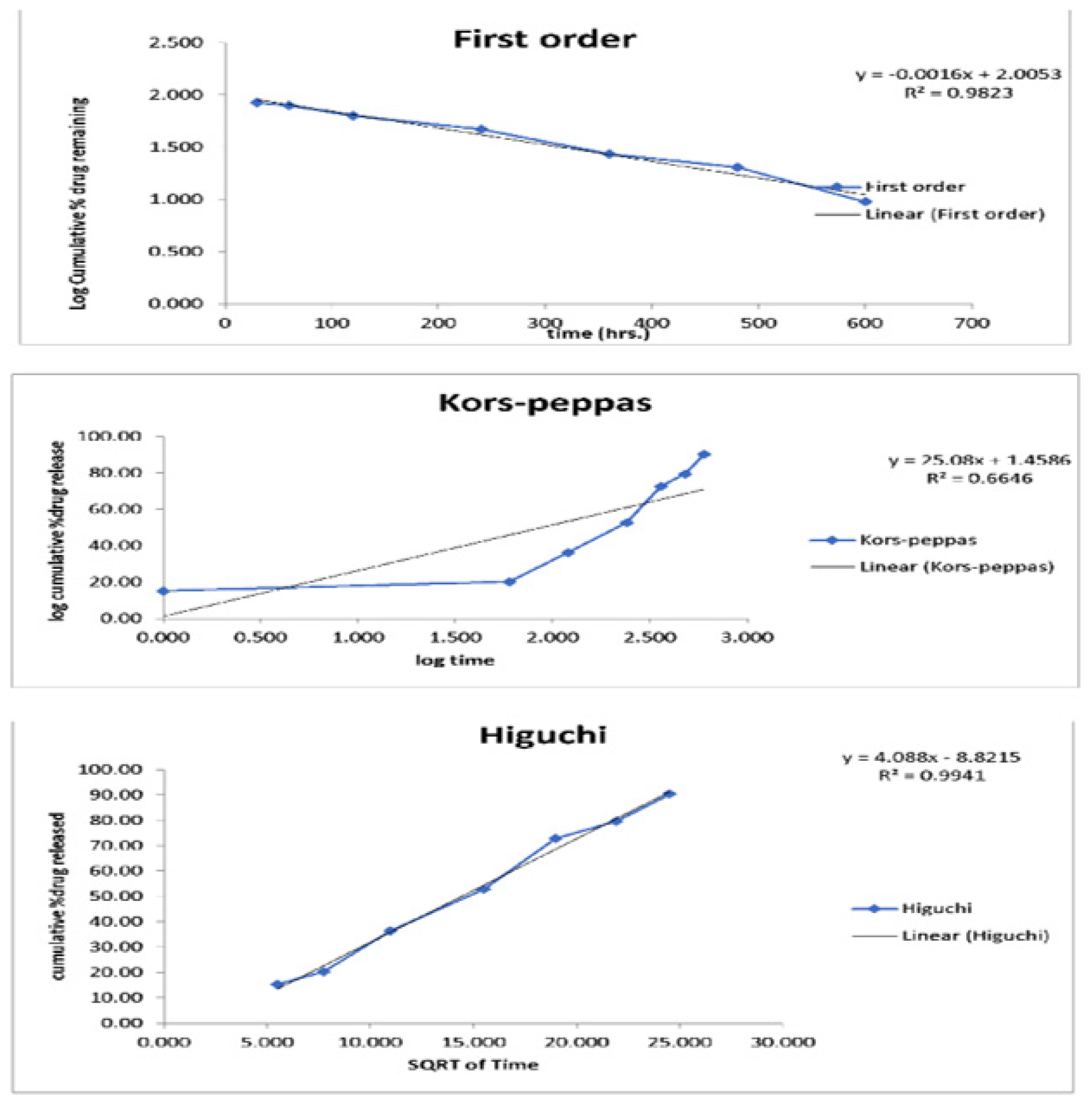
Figure 5:
Release kinetics studies.
Preliminary Phase (0-120 hr): In the 1st 120 hr, a progressive rise in drug release is noted, with 36.35% of the drug having been released. The gradual initial release is due to the regulated diffusion of the drug from the quatsome matrix, which inhibits an immediate burst release. The regulated early release demonstrates the stability of the F3 formulation and its capacity to inhibit premature drug leakage. Intermediate Phase (120-360 hr): Between 120 and 360 hr, the release rate markedly escalates, culminating in a cumulative release of 72.82% by 360 hr. This phase of accelerated release indicates that with time, the drug increasingly diffuses from the quatsome matrix. The release kinetics in this phase likely adhere to a diffusion-controlled process, wherein the drug is progressively transferred from the inner layers of the quatsomes. Concluding Phase (360-600 hr): During the concluding period, spanning from 360 to 600 hr, the drug release persists at a modest rate, culminating in a release of 90.45% by the 600-hr point. This phase illustrates that, even after an extended duration, the formulation sustains a regulated release, guaranteeing that the majority of the encapsulated medication is accessible for therapeutic effect.12 The prolonged release during this protracted duration underscores the efficacy of F3 in ensuring long-term medication availability, which is optimal for chronic illnesses necessitating continual administration.
The F3 formulation has an optimal profile for prolonged medication delivery. The progressive and regulated release reduces the likelihood of toxicity from elevated initial concentrations, while the sustained release guarantees prolonged therapeutic effects.13 F3 is a viable choice for applications requiring prolonged drug exposure, hence promoting patient compliance and improving therapeutic outcomes.
CONCLUSION
The research on retinoic acid-loaded quatsomes for topical keloid therapy has yielded encouraging outcomes. The quatsomes had a distinct morphology characterized by many spherical globules, signifying a stable and homogeneous formulation. The globule size was 104.5 nm, deemed optimum for improved skin penetration and effective drug delivery. The low Polydispersity Index (PDI) of 0.027 further substantiates the uniformity and homogeneity of the quatsomes, guaranteeing constant performance.
The Encapsulation Efficiency (EE) of 85.2% underscores the quatsomes’ capacity to successfully load and retain retinoic acid, rendering it a highly efficient carrier. The zeta potential of -41.9 mV signifies robust stability of the quatsome formulation, minimizing the risk of aggregation and providing extended shelf life. Furthermore, the in vitro release studies indicated that 90% of the retinoic acid was released within 10 hr, implying a sustained release mechanism. This sustained release is advantageous for topical use, offering lasting therapeutic effects and reducing the necessity for frequent reapplication. These findings indicate that retinoic acid-loaded quatsomes represent a promising topical delivery, providing a stable, efficient and sustained release formulation that may enhance therapeutic outcomes for patients with this difficult skin condition. Additional clinical trials are advised to confirm these findings and investigate the complete capabilities of this novel delivery technology.
Cite this article:
Ali AA, Raja K, Soundarapandi P, Sakthivel S, Venugopal V. Formulation and Evaluation of Retinoic Acid Loaded Quatsomes for Topical Application. J Young Pharm. 2025;17(1):187-93.
ACKNOWLEDGEMENT
This research work was supported by School of Pharmacy, Sri Balaji Vidyapeeth (Deemed to be University) Puducherry, India.
ABBREVIATIONS
CTAB | Cetyl-trimethyl ammonium bromide |
---|---|
EE | Encapsulation efficiency |
UV | Ultra Violet |
n | Release exponent |
R2 | Regression |
References
- Ferrer-Tasies L, Moreno-Calvo E, Cano-Sarabia M, Aguilella-Arzo M, Angelova A, Lesieur S, et al. Quatsomes: vesicles formed by self-assembly of sterols and quaternary ammonium surfactants. Langmuir. 2013;2013:20 [PubMed] | [CrossRef] | [Google Scholar]
- Battista S, Köber M, Bellio P, Celenza G, Galantini L, Vargas-Nadal G, et al. Quatsomes formulated with l-Prolinol-Derived surfactants as antibacterial nanocarriers of (+)- usnic acid with antioxidant activity. ACS Appl Nano Mater. 2022;2022:20 [PubMed] | [CrossRef] | [Google Scholar]
- Ginat DT. [cited Oct 18, 2024];In: Neuroimaging pharmacopoeia. 2015;363-365 [PubMed] | [CrossRef] | [Google Scholar]
- Sarohan AR. COVID-19: endogenous retinoic acid theory and retinoic acid depletion syndrome. Med Hypotheses. 2020;2020:20 [PubMed] | [CrossRef] | [Google Scholar]
- Rostron C. [cited Oct 18, 2024];In: Drug design and development. 2020 [PubMed] | [CrossRef] | [Google Scholar]
- Liu X, Ardizzone A, Sui B, Anzola M, Liu T, Veciana J, et al. [cited Oct 18, 2024];In: Om. 2017:134 [CrossRef] | [Google Scholar]
- Ardizzone A, Kurhuzenkau S, Illa-Tuset S, Faraudo J, Bondar M, Hagan D, et al. Nanostructuring lipophilic dyes in water using stable vesicles, Quatsomes, as scaffolds and their use as probes for bioimaging. Small. 2018;14(16) [PubMed] | [CrossRef] | [Google Scholar]
- Avdeef A. [cited Oct 18, 2024];In: Predicting Solubility of New Drugs. 2024:48-63. [CrossRef] | [Google Scholar]
- Vinayagam V, Palaniraja K, Ravi Y, Jayashankar A, Venugopal V. Innovative formulation of ethosomes: A study on properties and drug release kinetics. J Young Pharm. 2024;2024:20 [CrossRef] | [Google Scholar]
- Kato H, Nakamura A, Shimizu M. Effect of surfactant micelle size on the dispersibility of aqueous carbon black particle suspensions prepared by ultrasonication. Powder Technol. 2022;2022:20 [CrossRef] | [Google Scholar]
- Brgles M, Jurašin D, Sikirić MD, Frkanec R, Tomašić J. Entrapment of ovalbumin into liposomes-factors affecting entrapment efficiency, liposome size and zeta potential. J Liposome Res. 2008;2008:20 [PubMed] | [CrossRef] | [Google Scholar]
- Paswan SK, Saini TR. Comparative evaluation of drug release methods employed for nanoparticle drug release studies. Diss Technol. 2021;2021:20 [CrossRef] | [Google Scholar]
- AlMajed Z, Salkho NM, Sulieman H, Husseini GA. Modeling of the release kinetics of Sonosensitive targeted liposomes. Biomedicines. 2022;2022:20 [PubMed] | [CrossRef] | [Google Scholar]