DOI: 10.5530/097515050522
ABSTRACT
3–dimensional (3D) printing offers the chance to fabricate medications with more than a single active ingredient categorised by various properties and with diverse dissolution profiles. As a result, making composite medications may affect the decline in the number of used products. 3D printing in pharmaceuticals is a portion of the novel development called additive manufacturing, which means manufacturing three-dimensional compact substances from a digital file. Additive manufacturing is taken into consideration in the medical sector as it has the potential to advance treatment for certain conditions. The technology used mostly in the 3D printing of healthcare devices is termed powder bed fusion. Powder bed fusion is typically used for healthcare devices, as it can work with a wide variety of materials, including titanium, and nylon. Recent developments in technology and improved research in this sector can guarantee extra safe and effective cures. This technology appears to be an innovative tool that provides greater flexibility in medicine manufacturing and is likely to transform medication distribution schemes to a more diverse level in the future.
INTRODUCTION
Three–dimensional (3D) printing technology is an innovative quick prototyping method in which solid matter is fabricated by dropping some deposits into the structure. Rapid prototyping comprises the creation of physical models using computer–aided design in three dimensions.1 3D printing technology (3DPT) has allowed extraordinary elasticity in the scheme and build–up of composite substances, which can be used in modified, and programmable medication. It is an effective approach to overcome some of the predictability of medicinal unit operations. Currently, 3DPT is one of the wildest emerging divisions of technology, art, and science, and it is still extending its uses. 3DPT, as defined by the International Standard Organisation (ISO), is the construction of items by the testimony of a material with a print head, and nozzle/technology.2 The 3DPT is an additive manufacturing (AM) technique that is constructed on the principle of the deposition/solidification of consecutive coatings to form the digitally considered 3DPT.3 3DPT has been discovered for its capability to yield medicinal preparations as laser–based writing, printing–based inkjet, and nozzle–based deposition systems. In comparison to the normally used subtractive and developmental manufacturing approaches, this technique of additive manufacturing (AM) in which the portions are organised from 3D model information in the method of linking ingredients layer by layer, Rapid prototyping (RP) is a useful method of AM, and its benefits include reduced prototyping time, and costs, easy changes of manufactured goods at an intended level, the possibility of building up small things, personalised product series, or structures difficult to mould with subtractive methods. The first 3DPT used in pharmaceutics was achieved by inkjet printing (IJP) a binder solution on a powder bed, binding, therefore, the particles composed. The procedure was repetitive till the last anticipated structure was achieved.4
The primary 3DPT drug accepted by the Food and Drug Administration (FDA) in 2016 was IJP, which was the technique used to fabricate Spritam (levetiracetam) tablets. Significant advancements in imaging practises have been derived from numerous advancements in post-treatment software, allowing the generation of 3D computer–generated replicas in an ideal mode. The progressive abilities of MRI and CT to obtain volumetric data sets with isotropic voxels have occasioned the better usage of novel methods such as Three–Dimensional Volume Rendering (3DVR) and maximum intensity projection (MIP) for difficult circumstances in predictable radiological practise, moving from 2D images to 3D imaging development. The 3D arrangement has been described in numerous studies to develop the imagining of structural arrangements and their 3D connections to vessels, tumours, or additional compromised arrangements, providing more precise evidence than 2D images. Developments in programmed separation and image handling using computer–aided design (CAD) files have also opened the possibility for the generation of 3D computer–generated and printable replicas in a more precise method.6
This novel procedure of medical preparation, including separation, and 3DP, can be summarised in eight steps:7
- Image procurement.
- Dissection and transformation to SLG format.
- CAD usages.
- Generation of 3D printable copies.
- 3DP.
- Post-processing.
- Quality and cleaning control, and final.
- Surgery.
Among the above process is image attainment (primarily from RI or CT scan). The second is the breakdown, DICOM files are altered into CAD–TL files. Once breakdown is accomplished, these files are used for 3D picturing, and computer–generated clinical recreation. Steps 2 and 3 need the use of exact imagery and CAD software.8 In today’s world, both proprietary and open–source software solutions are available, with or without CE, and FDA approval. If the medical team considers having a 3DPT model for the case useful, the progression is maintained with the 3DPT of the anatomical model (Steps 4 and 5). The CAD model must be converted and prepared so that it can be read by the 3DPT machine. The 3D printing vendor usually provides the software essential for this. Post-processing, cleaning, and washing the model for a better surface finish follows 3DPT (Steps 6 and 7). Surgical planning by surgeons is also based on a 3DPT model, as well as residency training, and patient experiences. Three–dimensional printing of bone structures is frank and economic, but the process of scheming soft tissue structures is inflated, and time–consuming.9
This technology has also been designated to provide a substitute for therapeutic bulk production. To be sure, in 2015, the world’s first primarily 3DPT drug (Spritam) was accepted by the FDA, permitting the manufacture of an exclusive medication product that had both more drug stacking (up to 1000 mg), and speedy disintegration. The 3DPT used was a scaled–up binder jet imprinter, which presented four different fixed–dose powers. As the scheme was an alternate bulk manufacturing method, FDA authorization was completed via predictable governing pathways. An alternative possible bid for 3DPT dosage forms is for the bulk customization of drug products, a development that has been used nearly exclusively to produce hearing aids to date, with 10 million hearing aids presently in circulation worldwide. Due to the high level of design freedom as well as the on–claim competence offered by 3DPT, this technology has been forecast to disrupt the way that one treats patients, moving away from mass manufacture towards custom–made treatments (e.g., with tailored designs, sizes, dosages, and dose mixtures), as well as on the front–line (e.g., in hospitals or public pharmacies). Through the reorganisation of the manufacturing method, this new usage pathway could allow patients to ensure relaxed access to drugs within their community and diminish waiting times for those medications that need spontaneous grounding, or compounding by a pharmacist.
3DPT has also been specified for fabricating custom–made medication products in hard–to-reach regions, such as in calamity areas, for military processes, in low-or middle–income nations, and even for space operations. Due to being digitised in nature, DP is well located to be connected to other digital health tools, including artificial intelligence and isolated diagnostic tools like smart monitors and point–of-care tests, which could be directed to a clinician or data assessment and the generation of personalised treatment, facilitating the easy review and adjustment of treatments, or dosages.10
3DPT can promote the production of custom–made precision medications, including the capability to pick the ideal dose, appearance, flavour, dosage form, and discharge profile, while also decreasing the number of doses given to each patient by custom–designed multi–drug dosage forms with modified discharge profiles of separately contained medication. The altering of medication design may help various groups of patients. For instance, patients with vision deficiency could have modified designs to recognise drugs, and paediatric patients may have real personalised flavour cover, and arrival of drugs to develop compliance. Furthermore, opioids can be 3DPT with misuse–preventing, and alcohol–resistant properties.11
Three–dimensional (3D) printing in compounding enhances the elasticity to alter medication substances and their discharge profiles for each patient. It’s possible that nutritional supplement consumers would be interested in 3DPT supplements with controlled-release designs. Pharmacy studies have shown that pharmacists can print nutritional supplements in polypills in conjunction with set medicines to upsurge the interest and obedience of patients while reducing pill fatigue and ensuring the safety and uniformity of co-administered supplements. Pharmacists need to familiarise themselves with probable alimentary complement interactions with medicaments and personalise the dosage forms accordingly.12 In Data-Enriched Edible Pharmaceuticals (DEEP), quick response (QR) codes can be added directly to dosage forms to ensure that the medication is removed from its packaging with the information, patient information, and pharmacy information it needs to be abused. QR codes are readable on a smartphone with a QR code interpretation. An additional compound QR code is a so-called uniform.13 The Resource Radar (URL) is recommended as an alternative to using QR codes because of the URL’s capability to convey the patient extra complete information about the medication through a webpage. An Electric Health Record webpage can be accessed via a smartphone URL scanner, providing direct access to a patient’s confidential health information, even when medications are removed from packaging, and enabling clear communication between patients, and healthcare providers.14
Furthermore, limited studies have exploited the new 3DPT to lengthen the gastrointestinal withholding time of curcumin,15 acyclovir,16 amoxicillin,17 domperidone,18 riboflavin,19 and propranolol hydrochloride.20
TYPES OF 3D PRINTING
Inkjet Printing
This methodology to modify medication originates from the similar procedure of computer–operated IJP. It was improved for medicinal usage by the replacement of the ink with medicinal solutions having medicines and the usual paper with eatable pieces known as substrates. Dose adjustments are made by changing the number of sheets printed in a particular area or altering the part to be printed. The medication and excipients are considered in a proportion such that it has the probability of printing microdots on top of an eatable substrate. The two main printing types employed in IJP are thermal IJP and piezoelectric inkjet printers.
IJP (Figure 1) is characterised by drop generation and installation into two main types: CIJ and DOD inkjet lithography. The CIJ includes a non-stop pour of ink through an opening, and the generation of droplets and confession on top of the substrate will be organised by piezoelectric transducers at the hole, and the electrostatic field that supports the charged drops depositing on the substrate. Unlike the DOD- IJP, the development of the globule is based on demand by getting indications that it needs fewer amounts of ink than the constant IJP. Two categories of print heads are used for drop–on-demand printing, i.e., warm air print heads and piezoelectric print heads.21
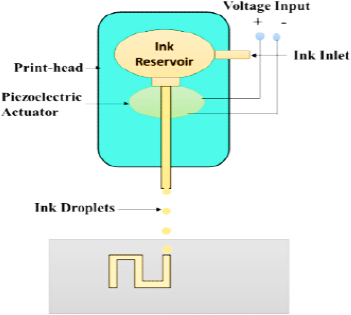
Figure 1.
Sketch of an Inkjet printer.
Amongst the two forms of printheads, the piezoelectric printheads are more beneficial for drug distribution usages as they can operate at room temperature, and an extensive variety of biocompatible, less volatile diluents can be used. While the thermal head needs more temperature and can cause deprivation of the active constituents.22
Fused Deposition Modelling (FDM)
FDM (Figure 2) is a method of physical extrusion in which a polymer strand is melted and extruded with a hot jet, layer by layer, to create an object. Hot–melt extrusion is used to make the drug–loaded strands regularly (HME). FDM 3DPT allows for the creation of substances with geometrical structures that aren’t possible with fine particle compaction, such as deep arrangements, and tablets of numerous designs (sphere, torus, cube, pyramid). To provide controlled release and quick discharge dose systems, it is also likely to change the drug discharge designs by modifying the infill proportion. One of the primary restrictions of FDM was the high printing temperatures essential for extrusion and printing, which caused a threat of drug deprivation. Though, now, the use of novel resources has enabled printing at a variety of lower temperatures to overwhelm this subject.23
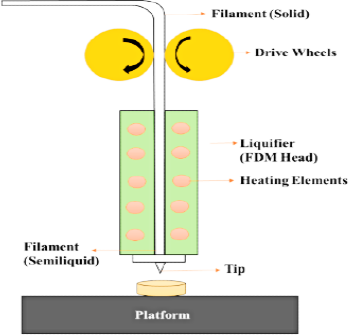
Figure 2.
Sketch of a fused deposition modelling.
Constituents must have appropriate rheological characteristics to make handling easier. These characteristics are dependent on the jet diameter, pressure control, feed amount, and other factors related to the feed material’s thermal properties, such as thermal conductivity, compactness, or glass transition temperature.24
Laser Dependent Writing Systems
It is of mainly two types.
Stereolithographic Method (SLG)
SLG (Figure 3) technology includes the curing of light–sensitive compounds (photopolymerization) to create 3D substances. By scanning an absorbed Ultraviolet (UV) laser over a photopolymerizable material in a coat–by-coat fashion, SLG uses a digital reflecting approach to induce an organic reaction in the photopolymer, which causes the gelation of the uncovered area.25 To complete the article’s fragments, this procedure is applied coat after coat.
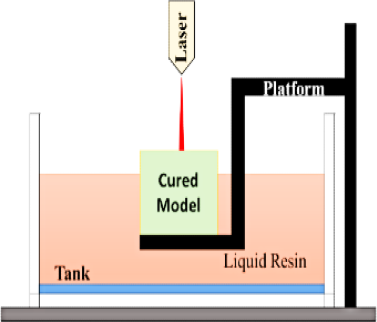
Figure 3.
Sketch of a stereolithography.
In SLG printers, energy is converted into molten photopolymerizable gum using an ultraviolet light ray as a laser. The ultraviolet light ray is steered through the superficial layer of melted gum by baffles on the axes x and y, precisely recreating the previously designed 3D Model.26
When a coating solidifies, the elevating stage raises its position to the height of a new coat of molten gum. The method is repeated another time, and so on until the 3D masterpiece is completed coat by coat. The viscosity of the cured coatings is determined by the energy of the UV light that the gum is exposed to. The gum should be FDA–approved for human use and can harden when subjected to laser beams.27
Selective Laser Sintering (SLS)
SLS (Figure 4) is a fine particle solidification method where a powder material is sintered under the melting temperature of the polymeric substance using a laser ray, which assists in melting and combining the polymeric substances, and forms a coating. Then the platform is let down, and a fresh fine particle bed is conveyed from the feed section, which afterward becomes exposed to the laser ray, and forms a coat–by-coat 3D assembly. The coat viscosity and printed item’s resolution depend on the laser usage, strength, speed of laser movement over the platform, and particle size of the powder blend. The benefit of SLS is the single–step printing procedure with high resolution, no usage of organic diluents, and no necessity for post-printing drying. This method has the drawback of API deprivation due to the melting method of laser sintering, the inadequate choice of photosensitive polymers, and the printing of hollow arrangements.28
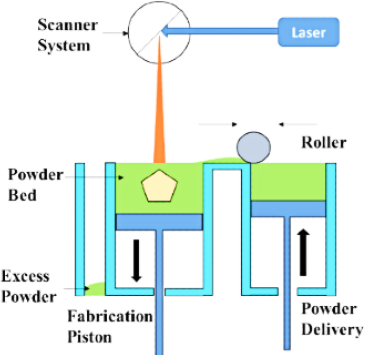
Figure 4.
A Sketch of selective laser sintering.
Usages of 3DPT in the Pharmaceutical Field
Various claims about 3DPT in the pharma arena29–31 are enlisted in Table 1.
3DP Technology | Dosage Form | Drugs Used |
---|---|---|
Binder Jet Printing | Tablets and cubic Tabular Devices | Paracetamol and Pseudoephedrine |
Direct Powder Extrusion | Tablets | Tramadol and Itraconazole |
Extrusion Based Printing | Tablets | Captopril |
Fused Deposition Modelling | Tablets and caplets | 4–AminoSalicylic Acid, Haloperidol, and Caffeine |
Inkjet 3DP | Nanosuspension | Folic Acid |
Pressure–assisted micro syringes | Tablets, and semisolids | Levetiracetam and Ramipril |
Selective Laser Sintering | Tablets | Lopinavir and Lidocaine HCl |
Semisolid Extrusion | Tablets, and suppositories | Levetiracetam, Glipizide, and Tacrolimus |
Thermal Inkjet Printing | Solid dosage forms | Prednisolone |
CONCLUSION
3D printing technology (3DPT) will likely be useful to the pharmaceutical industry. This could lead to customised medications. It would also improve cost–effectiveness and speed up production. 3DPT for making three-dimensional objects. It has become a standard method of manufacturing. Augmented design manufacturing reduces lead time and tooling costs for new yields. Several manufacturing methods are discussed, as well as some of the most common uses of 3DPT in healthcare. Only in the pharmaceutical field). Various types of 3DPT are being used in more and more research. The ability to manufacture modified 3DPs as a benefit of all the available 3DPT medicines is one of the most important features. The ability to modify the discharge profile and dosage of a 3D–printed tablet. The ability to use FDM HME to print injectable strategies or net inserts with pharmaceuticals could open a range of new possibilities for 3DPT in the medical industry. To create 3DPT products, research is necessary. The high temperatures of the process also limit how many medications can be formulated into filaments. Though, the versatility of 3D printed goods, and the sheer volume of industrial benefits that 3DPT offers, the research is worth it. More 3DPT may leave traces in the future. The technology has evolved into a widely used industrial tool that can be used in many biomedical science disciplines. However, the technology is potentially useful. In general, medical applications need various monitoring methods.
References
- Bose S, Ke D, Sahasrabudhe H, Bandyopadhyay A. Additive manufacturing of biomaterials. Prog Mater Sci. 2018;93:45-111. [PubMed] | [CrossRef] | [Google Scholar]
- Bandyopadhyay A, Bose S, Das S. 3D printing of biomaterials. MRS Bull. 2015;40(2):108-15. [CrossRef] | [Google Scholar]
- Mohapatra S, Kar RK, Biswal PK, Bindhani S. Approaches of 3D printing in current drug delivery. Sensors International. 2022;3:100146 [CrossRef] | [Google Scholar]
- Walczak R, Adamski K, Inkjet Adamski K. Inkjet 3D printing of microfluidic structures-on the selection of the printer towards printing your own microfluidic chips. J Micromech Microeng. 2015;25(8):085013 [CrossRef] | [Google Scholar]
- Ravenel JG, McAdams HP, Remy-Jardin M, Remy J. Multidimensional imaging of the thorax: Practical applications. J Thorac Imaging. 2001;16(4):269-81. [PubMed] | [CrossRef] | [Google Scholar]
- Griffith LG, Swartz MA. Capturing complex 3D tissue physiology . Nat Rev Mol Cell Biol. 2006;7(3):211-24. [PubMed] | [CrossRef] | [Google Scholar]
- Billiet T, Vandenhaute M, Schelfhout J, Van Vlierberghe S, Dubruel P. A review of trends and limitations in hydrogel-rapid prototyping for tissue engineering. Biomaterials. 2012;33(26):6020-41. [PubMed] | [CrossRef] | [Google Scholar]
- Dawood A, Marti Marti BM, Sauret-Jackson V, Darwood A. 3D printing in dentistry. Br Dent J. 2015;219(11):521-9. [PubMed] | [CrossRef] | [Google Scholar]
- William OOropallo W, Piegl LA. Ten challenges in 3d printing. Eng Comput. 2016;32(1):135-48. [CrossRef] | [Google Scholar]
- Gopinathan J, Noh I. Recent trends in bioinks for 3D printing. Biomater Res. 2018;22(1):1-5:11. [PubMed] | [CrossRef] | [Google Scholar]
- Campbell T, Williams C, Ivanova O, Garrett B. “’Could 3D printing change the world”’, Technologies, Potential, and Implications of Additive. Manufacturing, Atlantic Council, Washington, DC. 2011;3:1-16. [PubMed] | [CrossRef] | [Google Scholar]
- Kikuchi R, Yoshikawa S, Jayaraman PK, Zheng J, Maekawa T. Embedding QR codes onto B-spline surfaces for 3D printing. Comput Aided Des. 2018;102:215-23. [CrossRef] | [Google Scholar]
- Furet B, Poullain P, Garnier S. 3D printing for construction based on a complex wall of polymer-foam and concrete. Addit Manuf. 2019;28:58-64. [CrossRef] | [Google Scholar]
- Von Erlach T, Saxton S, Shi Y, Minahan D, Reker D, Javid F, Lee YA, Schoellhammer C, Esfandiary T, Cleveland C, Booth L, et al. Robotically handled whole-tissue culture system for the screening of oral drug formulations. Nat Biomed Eng. 2020;4(5):544-59. [PubMed] | [CrossRef] | [Google Scholar]
- Chandrasekar PH, Abraham OC, Klein J, Alangaden G, Chalasani G, Cassells L, Dansey R, Abella S, Karanes C, Peters W, Baynes R, et al. Low infectious morbidity after intensive chemotherapy and autologous peripheral blood progenitor cell transplantation in the outpatient setting for women with breast cancer. Clin Infect Dis. 2001;32(4):546-51. [PubMed] | [CrossRef] | [Google Scholar]
- Dorsey J, Gonska T. Bacterial overgrowth, dysbiosis, inflammation, and dysmotility in the Cystic Fibrosis intestine. J Cyst Fibros. 2017;16 Suppl 2:S14-23 [PubMed] | [CrossRef] | [Google Scholar]
- Singpanna K, Chareonying T, Patrojanasophon P, Rojanarata T, Sukma M, Opanasopit P, et al. Fabrication of a floating device of domperidone tablets using 3D-printing technologies. KEM. 2020;859:289-294. [CrossRef] | [Google Scholar]
- Fu J, Yin H, Yu X, Xie C, Jiang H, Jin Y, Sheng F, et al. Combination of 3D printing technologies and compressed tablets for preparation of riboflavin floating tablet-in-device (TiD) systems. Int J Pharm. 2018;549(1-2):370-9. [PubMed] | [CrossRef] | [Google Scholar]
- Karavasili C, Eleftheriadis GK, Gioumouxouzis C Andriotis EG, Fatouros DG. Mucosal drug delivery and 3D printing technologies: A focus on special patient populations. Adv Drug Deliv Rev. 2021;176:113858 [PubMed] | [CrossRef] | [Google Scholar]
- Griffey J. “The types of 3-D printing”. Libr Technol Rep. 2014;50(5):8-12. [PubMed] | [CrossRef] | [Google Scholar]
- Guo Y, Patanwala HS, Bognet B, Ma AWK. Inkjet and inkjet-based 3D printing: Connecting fluid properties and printing performance. RPJ. 2017;23(3):562-76. [CrossRef] | [Google Scholar]
- Melocchi A, Parietti F, Loreti G, Maroni A, Gazzaniga A, Zema L, et al. 3D printing by fused deposition modeling (FDM) of a swellable/erodible capsular device for oral pulsatile release of drugs. J Drug Deliv Sci Technol. 2015;30:360-7. [CrossRef] | [Google Scholar]
- Dumpa N, Butreddy A, Wang H, Komanduri N, Bandari S, Repka MA, et al. 3D printing in personalized drug delivery: An overview of hot-melt extrusion-based fused deposition modeling. Int J Pharm. 2021;600:120501 [PubMed] | [CrossRef] | [Google Scholar]
- Robles-Martinez P, Xu X, Trenfield SJ, Awad A, Goyanes A, Telford R, Basit AW, Gaisford S, et al. 3D printing of a multi-layered polypill containing six drugs using a novel stereolithographic method. Pharmaceutics. 2019;11(6):274 [PubMed] | [CrossRef] | [Google Scholar]
- Martinez PR, Goyanes A, Basit AW, Gaisford S. Fabrication of drug-loaded hydrogels with stereolithographic 3D printing. Int J Pharm. 2017;532(1):313-7. [PubMed] | [CrossRef] | [Google Scholar]
- Xu X, Robles-Martinez P, Madla CM, Joubert F, Goyanes A, Basit AW, Gaisford S, et al. Stereolithography (SLA) 3D printing of an antihypertensive polyprintlet: CCase study of an unexpected photopolymer-drug reaction. Addit Manuf. 2020;33:101071 [CrossRef] | [Google Scholar]
- Charoo NA, Barakh Ali SF, Mohamed EM, Kuttolamadom MA, Ozkan T, Khan MA, Rahman Z, et al. Selective laser sintering 3D printing – an overview of the technology and pharmaceutical applications. Drug Dev Ind Pharm. 2020;46(6):869-77. [PubMed] | [CrossRef] | [Google Scholar]
- Gao G, Ahn M, Cho WW, Kim BS, Cho DW. 3D printing of pharmaceutical application: Drug screening and drug delivery. Pharmaceutics. 2021;13(9):1373 [PubMed] | [CrossRef] | [Google Scholar]
- Esmaeili S, Shahali M, Kordjamshidi A, Torkpoor Z, Namdari F, Samandari SS, Ghadiri Nejad M, Khandan A, et al. An artificial blood vessel fabricated by 3D printing for pharmaceutical application. Int J Nanomedicine. 2019;6(3):183-94. [PubMed] | [CrossRef] | [Google Scholar]
- Sampat BN, Shadlen KC. Indian pharmaceutical patent prosecution: The changing role of Section 3 (d). PLoOS OneNE. 2018;13(4):e0194714 [PubMed] | [CrossRef] | [Google Scholar]