ABSTRACT
Background
Kidney stones form due to the crystallization of minerals and salts in the urinary tract. Despite advancements in understanding their pathophysiology, treatment options remain expensive and often lead to recurrence. Herbal therapies have gained attention as alternative treatments. This study evaluates the anti-urolithiatic activity of methanolic extracts of Nelumbo nucifera, Anogeissus latifolia, Leucas aspera, and their polyherbal formulation (PHF) in a urolithic rat model.
Materials and Methods
A 28-day study used a CaOx-induced rat model (70 mg/ kg) to induce urolithiasis. Rats received N. nucifera, A. latifolia, and L. aspera extracts (250/500 mg/kg), Cystone (750 mg/kg), or PHF (375 mg/kg). Urine, serum, and kidney analyses, including LDH, GSH, LPO, and histopathology, were performed (p <0.001).
Results
Methanolic extracts significantly reduced elevated biochemical markers (creatinine, calcium, phosphorus, uric acid, and alkaline phosphatase), restored urinary pH, and increased urine volume. PHF exhibited the highest efficacy, outperforming individual extracts. Antioxidant activity was enhanced, reducing kidney stone formation. N. nucifera showed the most potent activity among single extracts.
Conclusion
The phytoconstituents, particularly saponins, played a key role in reducing calcium oxalate crystal formation and recurrence. This study highlights polyherbal formulations as cost-effective and efficient alternatives for managing kidney stones.
INTRODUCTION
Kidney stones are one of the most common nephrological disorders (Rodriguez Cuellaret al., 2020). Kidney stones recur due to urinary tract formation, causing pain, hematuria, and hydronephrosis. Prevalence (16% globally) varies by diet, lifestyle, location, sex, and genetics, with India having the highest incidence (Madsen 2013; Guhaet al., 2019). About 70-80% of kidney stones consist of calcium phosphates and oxalates, with 1-5% prevalence in Asia. They form via crystal nucleation and aggregation of calcium, magnesium, phosphates, cystine, and uric acid, affecting both genders equally (Öneret al., 2015; Teoet al., 2021; Edvardsson et al., 2022). In modern times, kidney stone treatment often involves complex, sophisticated, and expensive surgical procedures. However, herbal remedies provide a cost-effective and widely accepted alternative (Papatsoriset al., 2024). Traditional medicinal plants, particularly in Ayurveda, have long been used to prevent crystallization, nucleation, and aggregation of urinary calculi, offering therapeutic benefits in the management of urolithiasis along with other pharmacological advantages (Rukariet al., 2024). The lotus, native to Asia, is revered as a sacred plant in traditional cultures. In tropical regions, it features a whip-shaped rhizome that retains its green coloration and continues to flower throughout its lifecycle (Caoet al., 2021). The lotus contains proteins, carbohydrates, and sugars (arabinose, galactose, mannose, xylose, rhamnose, glucuronic acid). It is rich in magnesium, calcium salts of ghattic acid, and phytochemicals like alkaloids, flavonoids, phenols, terpenoids, sterols, saponins, tannins, coumarins, quinones, and ellagic acid (Khanet al., 2024). Extensive phytochemical screening has successfully identified and extracted key bioactive compounds, particularly saponins, in significant proportions (Dalmarco,et al., 2010). The lotus contains vitamins, lipids, glycosides, and essential oils. Leucas aspera (Lamiaceae) derives its name from the Greek “Leukos” (white) and is widespread in India, especially the Himalayas, and Sri Lanka. The lotus is among the largest flowering plants. (Abdel-Alimet al., 2023). Another notable species, Anogeissus latifolia, commonly known as Gum Ghatti, Axle-wood, or the Indian Gum Tree, belongs to the Combretaceae family (Singhet al., 2023). It is widely distributed across Asian countries such as India, Sri Lanka, Myanmar, and Nepal, thriving in tropical and subtropical regions (Mahawaret al., 2024). This study aims to evaluate the in vitro and in vivo anti-urolithiatic activity of NNE, ALE, LAE, and PHF, focusing on the extraction and pharmacological significance of saponins.
MATERIALS AND METHODS
Collection of Plant Material and Preparation of Extract
N. nucifera, A. latifolia, and L. aspera were collected from Sri Venkateswara University, Tirupati, and authenticated by Dr. K. Madhava Chetty (IDs: 1078, 1080, 1088). Plants were air-dried for seven days in the shade.
Chemical Agents and Apparatus Required
Calcium Oxalate-AR, (Merck, India), and Cystone (Himalaya Company Limited, India) are selected for the practical studies. If needed selection of the required chemicals and reagents were included with analytical grades. Auto analyzer Semi followed by UV- spectrophotometer UV-1700 Pharma-spec, Shimadzu.
Animals
Male Wistar rats (150-200 g) were procured and acclimatized to laboratory conditions before the study. They were housed in a controlled environment with regulated temperature, relative humidity, and hygienic conditions. Standard feed and water were provided ad libitum in accordance with quarantine protocols. Animal experiments followed CPCSEA guidelines and were approved by IAEC, Jeeva Life Sciences (CPCSEA/IAEC/ JLS/18/07/22/029), adhering to ethical regulations.
Acute-toxicity studies
Calcium oxalate-induced urolithiasis rat model
To induce urolithiasis in rats, all groups (except the control group) were fed a commercially available pelleted diet with drinking water containing calcium oxalate (70 mg/kg, intraperitoneally) for 28 days (Table 1). Renal and serum biochemical parameters, along with histopathological studies, were subsequently performed (Ćorić-Martinovićet al., 2024).
Group No. | Group Name | Treatment |
---|---|---|
Normal | Normal control | Vehicle for 28 days. |
Control | Disease control | Water with Calcium oxalate 70 mg/kg/i.p was given to the individual rats for 28 days. |
Standard | Standard group | Cystone (750 mg/kg, orally) is given from the 14th to the 28th day with Calcium oxalate 70 mg/kg in drinking water. |
Group 1 | Test group 1 | NNE (250 mg/kg, orally) is given from the 14th to the 28th day with Calcium oxalate 70 mg/kg in drinking water. |
Group 2 | Test group 2 | NNE (500 mg/kg, orally) is given from the 14th to the 28th day with Calcium oxalate 70 mg/kg in drinking water. |
Group 3 | Test group 3 | ALE (250 mg/kg, orally) is given from the 14th to the 28th day with Calcium oxalate 70 mg/kg in drinking water. |
Group 4 | Test group 4 | ALE (500 mg/kg, orally) is given from the 14th to the 28th day with Calcium oxalate 70 mg/kg in drinking water. |
Group 5 | Test group 5 | LAE (250 mg/kg, orally) is given from the 14th to the 28th day with Calcium oxalate 70 mg/kg in drinking water. |
Group 6 | Test group 6 | LAE (500 mg/kg, orally) is given from the 14th to the 28th day Calcium oxalate 70 mg/kg in drinking water. |
Group 7 | Test group 7 | PHF (375 mg/kg, orally) is given from the 14th to the 28th day with Calcium oxalate 70 mg/kg in drinking water. |
Kidney’s Histological Studies
Histopathological evaluation was carried out using a microtome. The kidneys were isolated, fixed in formalin (10%), and embedded in paraffin for 5 mm thick sections. Sections measuring 5 mm thick were stained with hematoxylin and eosin. The specimen was examined under a microscope (Li et al., 2023).
Statistical Analysis
All the results were scrutinized by analysis of variance (Dunnett’s comparison test) using Prism (v8.2) and articulated as mean and SD. The significance (p<0.05) was considered.
RESULTS
Preliminary phytochemical screening Evaluation
Preliminary phytochemical evaluation revealed the presence of supportive active phyto-constituents such as alkaloids, flavonoids, tannins, phenols, saponins, phytosterol, terpenoids, and glycosides (Table 2).
Results | ||||
---|---|---|---|---|
Sl. No. | Phytochemicals | NNE | ALE | LAE |
1. | Alkaloids | – | + | + |
2. | Flavonoids | + | + | + |
3. | Tannins | + | + | + |
4. | Phenols | + | + | + |
5. | Carbohydrates | + | + | + |
6. | Saponins | + | + | + |
7. | Phytosterol | + | + | – |
8. | Terpenoids | + | + | + |
9. | Glycosides | + | + | + |
10. | Anthraquinones | – | – | – |
Acute oral toxicity
In acute oral toxicity evaluated on selected rats (both sexes) for 14 days, no signs and symptoms of toxicity were noticed at the limit-test dose of 2000 mg/kg administered as per the OECD guidelines.
Anti-urolithiatic activity on Calcium oxalate induced rat model
Methanolic extracts of NNE, ALE, LAE, and PHF improved creatinine, calcium, phosphorus, uric acid, and ALP in CaOx-induced rats. Ethylene glycol raised calcium, magnesium, and phosphate in the disease group, while extracts reduced these levels. PHF showed superior efficacy, also increasing oxalate levels more effectively than high-dose extracts (Tables 3, 4 and Figure 1).
Concentration (μg/mL) | DPPH Assay (AA) | DPPH Assay (NNE) | DPPH Assay (ALE) | DPPH Assay (LAE) | OH* Radical Assay (AA) | OH* Radical Assay (NNE) | OH* Radical Assay (ALE) | OH* Radical Assay (LAE) | Superoxide Radical Assay (AA) | Superoxide Radical Assay (NNE) | Superoxide Radical Assay (ALE) | Superoxide Radical Assay (LAE) |
---|---|---|---|---|---|---|---|---|---|---|---|---|
1000 | 61.81±0.24 | 44.7±0.26 | 36.84±0.53 | 32.91±0.76 | 59.72±0.21 | 42.6±0.23 | 30.81±0.23 | 34.74±0.53 | 58.72±0.45 | 37.49±0.52 | 29.81±0.53 | 33.74±0.63 |
500 | 57.78±1.02 | 33.52±0.55 | 29.74±0.70 | 29.48±0.75 | 55.69±0.23 | 31.42±0.52 | 27.38±0.56 | 27.64±0.48 | 54.69±0.56 | 29.12±0.45 | 26.38±0.35 | 26.64±0.25 |
250 | 51.25±0.096 | 30.92±0.77 | 25.77±0.46 | 25.77±0.46 | 49.48±0.53 | 28.82±0.25 | 23.67±0.53 | 23.67±0.56 | 47.19±0.42 | 26.16±0.41 | 22.67±0.12 | 22.67±0.53 |
125 | 40.21±2.31 | 26.64±0.42 | 24.96±0.71 | 24.86±0.40 | 38.11±0.25 | 24.54±0.23 | 22.76±0.58 | 22.86±0.86 | 37.11±0.53 | 22.94±0.12 | 21.76±0.13 | 21.86±0.36 |
62.5 | 36.31±0.36 | 25.18±0.29 | 22.6±0.86 | 23.42±0.40 | 34.21±0.15 | 23.07±0.56 | 21.32±0.42 | 20.5±0.12 | 33.21±0.52 | 21.67±0.23 | 20.32±0.63 | 19.5±0.31 |
IC50 (μg/ mL) | 82.25±0.23 | 85.56±0.15 | 75.25±0.45 | 68.56±0.52 | 86.32±0.23 | 82.25±0.35 | 76.22±0.65 | 58.56±0.85 | 80.25±0.52 | 78.46±0.19 | 73.25±0.49 | 62.56±0.45 |
Results | |||||
---|---|---|---|---|---|
Groups | Weight of Calcium Reduced (mg) | ||||
Test 1 | Test 2 | Test 3 | MEAN | % Dissolution | |
Blank | 5 | 5 | 5 | 5.00 | 0 |
STD (Neeri) | 3.8 | 3.9 | 3.5 | 4.01*** | 80.26 |
NNE (100 mg/mL) | 3.9 | 4.2 | 3.5 | 4.11*** | 82.29 |
ALE (100 mg/mL) | 2.9 | 3.8 | 3.5 | 3.73*** | 74.53 |
LAE (100 mg/mL) | 3.5 | 3.9 | 3.9 | 4.04*** | 8.78 |
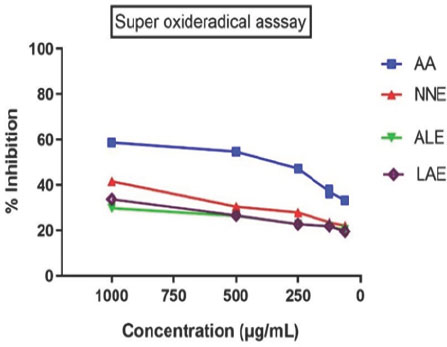
Figure 1:
Effect of extracts on in vitro antioxidant activity. *p<0.05, **p<0.01, ***p<0.01. Values are mean±SEM, n=6, when compared with control by using one-way ANOVA followed by Tukey’s multiple comparison test. ANOVA: Analysis of variance, SEM: Standard error of the mean, NNE, ALE, LAE, and PHF: Nelumbo nucifera, Anogeissus latifolia, Leucas aspera, and PHF extract.
In vitro anti-urolithic activity
Titrimetric analysis measured calcium oxalate stone dissolution. Nelumbo nucifera and Leucas aspera showed 80% dissolution, while Anogeissus latifolia achieved 74%, compared to the blank and positive control (Neeri) (Figure 2). This demonstrates that selected plants showed significant anti-urolithiasis activity in vitro. These plants can be used effectively to treat urolithiasis.
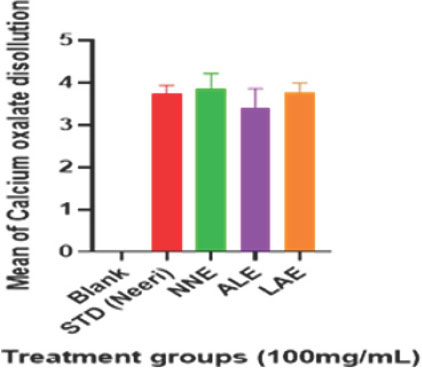
Figure 2:
Effect of extracts on in vitro anti-urolithiasis activity. *p<0.05, **p<0.01, ***p<0.01. Values are mean±SEM, n=6, when compared with control by using one-way ANOVA followed by Tukey’s multiple comparison test. ANOVA: Analysis of variance, SEM: Standard error of the mean, NNE, ALE, LAE, and PHF: Nelumbo nucifera, Anogeissus latifolia, Leucas aspera, and PHF extract.
Calcium oxalate-induced renal stones caused crystal deposits, tubular atrophy, cystic changes, and inflammation. Cystone (70 mg/kg) and plant extracts (250-500 mg/kg, 28 days) significantly reduced these abnormalities (Figure 3). CaOx treatment significantly (p<0.01) reduced urine volume without altering pH. Cystone and plant extracts (250-500 mg/kg) increased urine volume (p<0.05-0.01) compared to controls, with no pH change. Similarly, rats treated with N. nucifera (NNE), A. latifolia (ALE), L. aspera (LAE), and PHF at doses of 250, 500, and 375 mg/kg body weight showed a significant (p<0.05 to 0.01) increase in urine volume, without affecting urine pH (Figure 4).
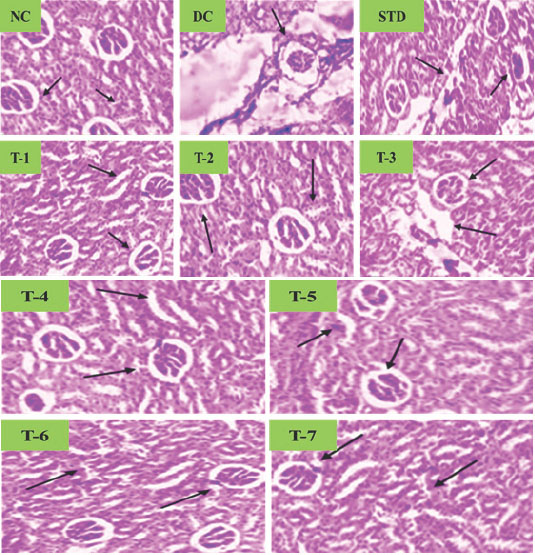
Figure 3:
Effect of selected plant extracts on Calcium oxalate-induced Urolithiasis Rats on kidney tissue histology.
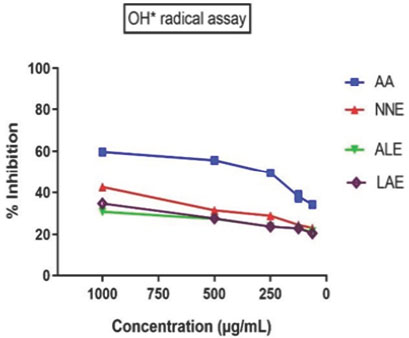
Figure 4:
Hydroxyl (OH·) radical scavenging assay. The graph depicts the percentage inhibition of hydroxyl radicals at varying concentrations (1000-250 μg/mL) for AA (Ascorbic Acid), NNE (sample), ALE (sample), and LAE (sample). Data points represent mean values, showing a dose-dependent inhibition trend.
CaOx (70 mg/kg, i.p.) induced hyperoxaluria, altering urinary markers and causing tubular damage. Cystone (500-750 mg/ kg) and plant extracts (250-500 mg/kg) restored levels, reduced oxidative stress, and prevented lithiasis. PHF was most effective. Histological analysis showed reduced tubular damage and crystal deposits with treatment.
DISCUSSION
In the current study, the calcium oxalate-induced model in Wistar rats was used as a representation of the human urinary system (Wanget al., 2025). Previous research has shown that renal crystal deposition is more pronounced in male rats compared to females (Peeterset al., 2022; Shi,et al., 2025). Calcium Oxalate (CaOx) renal stone formation begins with the deposition of calcium phosphate plaques, also known as Randall’s plaques. These plaques attract cations, which, in turn, form insoluble calcium oxalate salts that deposit on the renal interstitium. The oxalate crystals then attach to renal cells, damaging cell walls, promoting crystal aggregation, and facilitating further crystal growth, ultimately leading to retention in the renal tubules (Kauret al., 2025; Chung H.J. 2014; Kostovet al., 2025). NNE, ALE, LAE, and PHF reduced urinary oxalate levels and LDH activity, lowering oxalate accumulation. CaOx crystals increased LPO and oxidative stress, leading to kidney damage. Treated groups showed reduced urine output and filtration rates, causing nitrogenous waste buildup (Ranet al., 2025; Kanlayaet al., 2024). Treatments with PHF reduced the deposition of calcium oxalate crystals and lowered the levels of creatinine, phosphorus, and uric acid. The extracts of NNE, ALE, and LAE also decreased calcium oxalate deposition, minimizing damage to the urinary system (Simhadri and Leslie, 2024).
The deposition of CaOx crystals causes renal and histological damage in various parts of the renal system. A microscopic study of kidney sections from lithiatic control rats showed CaOx crystals in tubular and interstitial spaces, accompanied by glomerular congestion and tubular necrosis. However, treatment with NNE, ALE, LAE, and PHF extracts resulted in a reduction of CaOx crystal deposition. High concentrations of CaOx can lead to renal tubular obstruction, glomerular impairment, and tubular necrosis (Kaleet al., 2024; Houet al., 2025; Rajet al., 2024). The plant extracts showed stone-dissolution properties, likely due to their saponin content. NNE, ALE, and LAE contained saponins, flavonoids, and phytosterols, contributing to anti-lithiatic and antioxidant effects. These phytochemicals may prevent CaOx supersaturation, reducing crystal deposition. The antioxidant activity improved renal markers by inhibiting oxidative stress. The study confirms their inhibitory and preventive effects on urolithiasis (Mohamedet al., 2024).
CONCLUSION
These studies conclude that the administration of NNE, ALE, LAE, and particularly PHF decreases urinary stone formation. Therefore, in the early stages of stone formation, Nelumbo nucifera, Anogeissus latifolia, and Leucas aspera plant extracts are recommended in the recurrent pattern of renal stone disease.
The mechanism behind this effect may be due to antioxidant nephroprotective properties as well as a reduction in urinary stone-forming constituent concentrations. However, further research is needed to determine the precise mechanism of this behavior.
Cite this article:
Panda S, Erigi NC, Gaddapuram TK. In vitro Anti-Urolithiatic Evaluation of a Polyherbal Formulation against Calcium Oxalate-Induced Urolithiasis in Rats. J Young Pharm. 2025;17(2):470-6.
REFERENCES
- Abdel-Alim, M. E., Serag, M. S., Moussa, H. R., Elgendy, M. A., Mohesien, M. T., & Salim, N. S. (2023). Phytochemical Screening and Antioxidant Potential of Lotus corniculatus and Amaranthus viridis. Egyptian Journal of Botany, 63(2), 665–681. https://doi.org/10.21608/ejbo.2023.158720.2118
- Cao, D., Lin, Z., Huang, L., Damaris, R. N., & Yang, P. (2021). Genome-wide analysis of AP2/ERF superfamily in lotus (Nelumbo nucifera) and the association between NnADAP and rhizome morphology. BMC Genomics, 22(1), 171. https://doi.org/10.1186/s12864-021-07473-w
- Chung, H.-J. (2014). The role of Randall plaques on kidney stone formation. Translational Andrology and Urology, 3(3), 251–254. https://doi.org/10.3978/j.issn.2223-4683.2014.07.03
- Ćorić-Martinović, V., Delalić, Đ., Brežni, T., Vuković, J., & Prkačin, I. (2024). Wcn24-2065 the effect of the presence of multiple (accessory) renal arteries on biochemical parameters of renal function and hemodynamic measurements. Kidney International Reports, 9(4), S327. https://doi.org/10.1016/j.ekir.2024.02.632
- Dalmarco, E., Koelzer, J., Pizzolatti, M., Frode, T., & Dalmarco, J. (2010). Isolation and identification of bioactive compounds responsible for the antibacterial efficacy of Lotus corniculatus var. Sã o Gabriel. International Journal of Green Pharmacy, 4(2). https://doi.org/10.4103/0973-8258.63886
- Edvardsson, V. O., & Sas, D. J. (2022). Urinary stone disease and nephrocalcinosis. In F. Emma, S. L. Goldstein, A. Bagga, C. M. Bates, R. Shroff (Eds.), Pediatric nephrology (pp. 1295–1322). Springer International Publishing. https://doi.org/10.1007/978-3-030-52719-8_53
- Guha, M., Banerjee, H., Mitra, P., & Das, M. (2019). The demographic diversity of food intake and prevalence of kidney stone diseases in the Indian continent. Foods, 8(1), 37. https://doi.org/10.3390/foods8010037
- Hou, Y., Liu, Y., Yang, Y., Xu, S., & Yang, F. (2025). Exposure to MC-LR activates the RAF/ERK signaling pathway, leading to renal inflammation and tissue damage in mice. Journal of Toxicology and Environmental Health. Part A, 88(7), 301–309. https://doi.org/10.1080/15287394.2024.2435632
- Kale, M. D., Kadam, S. P., Shravage, B. V., & Nikam, V. S. (2024). From computational prediction to experimental validation: Hesperidin’s anti-Urolithiatic activity in sodium oxalate-induced urolithiasis models in fruit flies and mice. Toxicology and Applied Pharmacology, 492, (Article 117104). https://doi.org/10.1016/j.taap.2024.117104
- Kanlaya, R., Kuljiratansiri, R., Peerapen, P., & Thongboonkerd, V. (2024). The inhibitory effects of epigallocatechin-3-gallate on calcium oxalate monohydrate crystal growth, aggregation and crystal-cell adhesion. Biomedicine and Pharmacotherapy, 170, (Article 115988). https://doi.org/10.1016/j.biopha.2023.115988
- Kaur, M., Varanasi, R., Nayak, D., Tandon, S., Agrawal, V., & Tandon, C. (2025). Molecular insights into cell signaling pathways in kidney stone formation. Urolithiasis, 53(1), 30. https://doi.org/10.1007/s00240-025-01702-7
- Khan, S., Ahsan, F., Mahmood, T., & Bano, S. (2024). Anogeissus latifolia: A Comprehensive Review from Ethanobotanical Insights to Future Pharmacological Frontiers. Chemistry and Biodiversity, 21(12), Article e202401378. https://doi.org/10.1002/cbdv.202401378
- Kostov, K., Simeonova, T., Ignatov, B., & Eftimova, T. (2025). Evaluation of individual cardiovascular risk in pre-dialysis CKD patients by using the ratio of calcium-phosphorus product to estimated glomerular filtration rate (Ca× P/eGFR). Biomedicines, 13(1), 235. https://doi.org/10.3390/biomedicines13010235
- Li, B., Liu, Z., Shao, L., Qiu, B., Bu, H., & Tian, J. (2024). Point transformer with federated learning for predicting breast cancer HER2 status from hematoxylin and eosin-stained whole slide images. http://arxiv.org/abs/2312.06454. Proceedings of the AAAI Conference on Artificial Intelligence, 38(4), 3000–3008. https://doi.org/10.1609/aaai.v38i4.28082
- Madsen, M. G. (2013). Urinary biomarkers in hydronephrosis. Danish Medical Journal, 60(2), Article B4582. PubMed: 23461995
- Mahawar, S., Arya, A., Singh, H., & Kumar, S. (2024). A comprehensive review of medicinal plants of Northeast India used in urolithiasis. Journal of Drug Research in Ayurvedic Sciences, 9(5), 293–309. https://doi.org/10.4103/jdras.jdras_367_23
- Mohamed, D. A., Mabrok, H. B., Ramadan, A. A., & Elbakry, H. F. (2024). The potential role of alkaline diets in prevention of calcium oxalate kidney stone formation. Food and Function, 15(24), 12033–12046. https://doi.org/10.1039/d4fo03567d
- Öner, M., Khan, A., & Khan, S. R. (2015). Importance of calcium-based scales in kidney stone. In Mineral scales and deposits (pp. 393–416). Elsevier. https://doi.org/10.1016/B978-0-444-63228-9.00015-2
- Papatsoris, A., Alba, A. B., Galán Llopis, J. A., Musafer, M. A., Alameedee, M., Ather, H., Caballero-Romeu, J. P., Costa-Bauzá, A., Dellis, A., El Howairis, M., Gambaro, G., Geavlete, B., Halinski, A., Hess, B., Jaffry, S., Kok, D., Kouicem, H., Llanes, L., Lopez Martinez, J. M., . . . Tuerk, C. (2024). Management of urinary stones: State of the art and future perspectives by experts in stone disease. Archivio Italiano di Urologia, Andrologia, 96(2), Article 12703. https://doi.org/10.4081/aiua.2024.12703
- Peeters, L., Foubert, K., Breynaert, A., Schreurs, G., Verhulst, A., Pieters, L., & Hermans, N. (2022). Effects of medicagenic acid metabolites, originating from biotransformation of an Herniaria hirsuta extract, on calcium oxalate crystallization in vitro. Journal of Ethnopharmacology, 285, Article 114860. https://doi.org/10.1016/j.jep.2021.114860
- Raj, S., Gnana Soundara Rajan, M. S., Ramasamy, S., Rajamony Suthies Goldy, R. I., Ariyamuthu, R., Sudhagar, M., Gandhi, S., Shoba, P., & Gurusamy, M. (2024). An in vitro anti-urolithiasis activity of a herbal formulation: Spinacia oleracea L. and Coriandrum sativum L. Clinical Complementary Medicine and Pharmacology, 4(1), Article 100124. https://doi.org/10.1016/j.ccmp.2023.100124
- Ran, Y., Liu, Z., Ma, H., Li, C., Zhou, J., Li, D., Guo, Z., Dan, L., Zhao, Z., Zou, Z., Su, S., & Yin, Z. (2025). Associations between 25-hydroxyvitamin D/calcium/alkaline phosphatase levels and the risk of developing kidney stones: Results from NHANES (2013–2018)-based and Mendelian randomization studies. Medicine, 104(4), Article e41323. https://doi.org/10.1097/MD.0000000000041323
- Rodriguez Cuellar, C. I., Wang, P. Z. T., Freundlich, M., & Filler, G. (2020). Educational review: Role of the pediatric nephrologists in the work-up and management of kidney stones. Pediatric Nephrology, 35(3), 383–397. https://doi.org/10.1007/s00467-018-4179-9
- Rukari, T. G., Varadekar, M. M., Dhuri, P. B., Walke, S. S., Kashalikar, V. M., Shetye, S. S., & Jagtap, V. A. (2024). Concise review on Nature’s cure for kidney stones-unveiling the potential of surrounding. Medicinal Plants, 11(11), 595–603. https://doi.org/10.13040/IJPSR.0975-8232.IJP.11(11).595-03
- Sewell, F., Ragan, I., Horgan, G., Andrew, D., Holmes, T., Manou, I., Müller, B. P., Rowan, T., Schmitt, B. G., & Corvaro, M. (2024). New supporting data to guide the use of evident toxicity in acute oral toxicity studies (OECD TG 420). Regulatory Toxicology and Pharmacology: RTP, 146, Article 105517. https://doi.org/10.1016/j.yrtph.2023.105517
- Shi, M., Su, X., Xiang, H., Song, Q., & Yang, S. (2025). Advances in the mechanism of urinary proteins in calcium oxalate kidney stone formation. Urolithiasis, 53(1), 27. https://doi.org/10.1007/s00240-025-01703-6
- Simhadri, P. K., & Leslie, S. W. (2024). Calcium deposition and other renal crystal diseases. Stat pearls [Internet]. In Stat Pearls Publishing. https://www.ncbi.nlm.nih.gov/books/NBK599516/#article-154870.s2
- Singh, P., Singh, V., Tiwari, R. C., Negi, D., & Pal, S. (2023). Dhava (Anogeissus latifolia): Axle wood from the treasure of Ayurveda. Journal of Ayurveda and Integrated Medical Sciences, 8(6), 174–183. https://doi.org/10.21760/jaims.8.6.28
- Teo, Z. L., Tham, Y.-C., Yu, M., Chee, M. L., Rim, T. H., Cheung, N., Bikbov, M. M., Wang, Y. X., Tang, Y., Lu, Y., Wong, I. Y., Ting, D. S. W., Tan, G. S. W., Jonas, J. B., Sabanayagam, C., Wong, T. Y., & Cheng, C.-Y. (2021). Global prevalence of diabetic retinopathy and projection of burden through 2045: Systematic review and meta-analysis. Ophthalmology, 128(11), 1580–1591. https://doi.org/10.1016/j.ophtha.2021.04.027
- Wang, B., Tan, Z., She, W., Wang, X., Guan, X., Tao, Z., Guo, F., Xu, H., & Deng, Y. (2025). Characterizing chemokine signaling pathways and hub genes in calcium oxalate-induced kidney stone formation: Insights from rodent models. Biochemical Genetics. https://doi.org/10.1007/s10528-025-11036-z