ABSTRACT
Metformin is an oral hypoglycemic agent and it is the primary medication for managing hyperglycemia. The relationship between metformin, gut microbiota and their combined effects on metabolic health and cognitive function remains inadequately explored, particularly concerning the modulation of gut microbes by metformin. Metformin recognized for its role in diabetes management, emerging evidence highlights its influence on gut microbiota and suggesting that its therapeutic effects extend beyond the gastrointestinal tract. The review delves into metformin’s capacity to modulate epigenetic pathways, including DNA methylation and histone modifications, thereby regulating gene expression related to inflammation and metabolism and modification of Key beneficial microbes, such as Akkermansia muciniphila and various Lactobacillus species, promoting systemic health through the production of SCFAs and modulation of gut-brain axis signaling. The role of gut microbiota in influencing neuroinflammation, cognitive function and metabolic health is well-explored in this review, revealing metformin’s potential to enhance cognitive performance, protect against neurodegenerative disorders and reduce neuroinflammation. This review provides critical insights into the mechanistic underpinnings of metformin’s actions, paving the way for future research on its broader applications in metabolic and cognitive health.
INTRODUCTION
Metformin has served as an oral hypoglycemic agent and remains the primary medication for managing hyperglycemia. Current research is exploring its potential for various other uses beyond its initial approval. Significant findings indicate that metformin can act as an adjunct therapy in the treatment of several cancers, including breast, blood, colorectal, endometrial, melanoma and bone cancers. Its applications also extend to treating obesity and liver conditions like Non-Alcoholic Fatty Liver Disease (NAFLD). More recently, attention has shifted to its effects on cardiovascular conditions, such as vascular diseases, atherosclerosis, pulmonary arterial hypertension and arrhythmias. Emerging uses include its potential role in anti-aging therapies and kidney diseases. While metformin is often contraindicated in patients with significant renal impairment due to its association with diabetes, research into its AMPK signalling pathway suggests it may improve renal function and positively influence all the aforementioned conditions (Lv and Guo, 2020) (Buet al., 2022). Additionally, it is being considered as an alternative for managing gestational diabetes, with studies indicating that it may be comparable to insulin therapy in controlling hyperglycemia (Paschouet al., 2023). In the context of Polycystic Ovary Syndrome (PCOS), metformin is utilized to decrease insulin resistance. It is viewed as a second-line treatment option for patients with hyperglycemia, following the use of oral contraceptives (Kim, 2021). In addition, metformin has proven to be highly effective in treating tuberculosis, thanks to its antimicrobial effects (Maliket al., 2018). Continued investigations are revealing additional potential uses for metformin, largely due to its exceptional mechanisms, including the ability to alter the composition of gut microbiota (Muelleret al., 2021). This review aims to explore the intricate relationship between metformin, gut microbiota and their collective impact on metabolic health and cognitive function, providing insights into the multifaceted roles of this medication beyond traditional diabetes management. Emerging evidence suggests that the influence of metformin on gut microbiota extends beyond the gastrointestinal tract, impacting systemic health through mechanisms involving epigenetic regulation and neuroimmune pathways. Metformin’s ability to modulate epigenetic processes, such as DNA methylation and histone modifications, facilitates the expression of genes involved in inflammation and metabolism. Additionally, its effects on the gut-brain axis underscore the potential of metformin to reduce neuroinflammation and enhance cognitive function by promoting beneficial microbial metabolites.
Impact of Metformin on Gut Microbiota
Metformin in GIT
Recent studies suggest that metformin primarily targets the gut rather than the liver. In experiments where metformin was administered intravenously, no significant glucose-lowering effect was observed, further supporting the notion that metformin acts locally in the gut rather than exerting solely systemic effects. This hypothesis is linked to the OCT 1, a protein present in intestinal cell lines, which is likely involved in the absorption of metformin. Alterations in this protein can lead to gastrointestinal intolerance.
Metformin impact on gut microbiota
The gut harbors a diverse array of bacteria and metformin treatment significantly enhances the abundance of beneficial gut microbiota, which plays a vital role in promoting gut. Key beneficial microbes, including Akkermansia muciniphila, Butyricimonas, Parabacteroides and various Lactobacillus species (such as Lactobacillus intestinalis and Lactobacillus johnsonii), are increased. This contributes to improved intestinal integrity, enhanced metabolic processes and the production of SCFAs. Other beneficial genera, including Christensenella, Alloprevotella, Romboutsia, Anaerotruncus, Roseburia, Alistipes and Coprococcus, further promote gut homeostasis and reduce pro-inflammatory markers.
Reduction of harmful bacteria by metformin
Metformin treatment effectively reduces several harmful bacteria associated with gut dysbiosis, such as Clostridium perfringens and Escherichia coli, which are linked to pathogenic infections. The decrease in inflammation-associated genera, including Desulfovibrio, Papillibacter, Dorea and Muribaculum, alongside pathogenic bacteria like Proteus and Klebsiella, underscores metformin’s role in mitigating gut-related infections. This modification of the gut microbiome, through the suppression of harmful genera such as Acinetobacter, Achromobacter and Azorhiziphilus, highlights metformin’s protective effects and its potential to maintain a balanced gut microbiota, ultimately supporting overall metabolic health and reducing inflammation (Leeet al., 2021).
SAFAs and metabolic benefits
Metformin influences the composition of gut microbiota by directly impacting bacterial growth and modifying the intestinal environment. It promotes the proliferation of bacteria that produce SCFAs in individuals with T2DM, resulting in increased SCFA levels in the colon, which ultimately enhances host metabolism (Muelleret al., 2021). Propionate has been well-studied as a substrate for hepatic gluconeogenesis. Evidence suggests that propionate is converted to glucose through intestinal gluconeogenesis before reaching the liver, providing metabolic benefits in maintaining energy balance. This is demonstrated by reduced adiposity and body weight despite similar food intake levels, alongside improved glucose regulation, characterized by decreased hepatic glucose output. Thus, SCFAs improving glucose regulation by reducing hepatic glucose and activation of intestinal gluconeogenesis and heightened GLP-1 secretion, which is potential for glycemic control by influencing intestinal gluconeogenesis.
Influence on bile and FXR signaling
Enhancing intestinal mucosal barrier
The alterations in gut microbiota induced by metformin are vital for its anti-inflammatory effects. Recent studies show that metformin enhances the protective role of the intestinal mucosal barrier by increasing the abundance of Akkermansia muciniphila and the count of goblet cells, which leads to a thicker mucus layer that alleviates intestinal inflammation. Moreover, metformin raises the relative abundance of Lactobacillus spp. and Akkermansia spp., effectively correcting the microbial imbalance and colonic inflammation caused by experimental colitis, while also maintaining the integrity of the mucus barrier (Keet al., 2021).
Anti-tumor effects via gut microbes
Metformin effects on the gut microbiome also contribute to its anti-tumor properties. Oral administration of metformin, as opposed to intraperitoneal injection, was found to inhibit tumor growth in mice on a high-fat diet. Furthermore, the transfer of gut bacteria from metformin-treated mice to other mice significantly reduced tumor progression, linked to an increase in SCFA-producing bacteria and a reduction in the expression of genes involved in cholesterol synthesis within the tumor (Broadfieldet al., 2022).
Impact on small intestinal microbiome
Metformin extended the lifespan of C. elegans in co-culture with Escherichia coli, possibly due to the metabolite agmatine derived from gut microbiota. However, the relationship between metformin’s lifespan-extending effects in T2DM patients and elevated agmatine levels remains hypothetical. Most clinical observations concerning the impact of metformin on gut microbiota mainly focus on changes in fecal microbiota.
However, metformin also affects the microbiome in the small intestine, inducing distinct modifications in the microbiota of the duodenum, jejunum and ileum in rodent models. The shifts in microbial composition in the small bowel correspond with changes in the activity of genes related to glucose and fatty acid absorption in the intestines, enhancing the beneficial metabolic effects of metformin. Consequently, the microbiota across various segments of the gastrointestinal tract may serve different functions (Chenget al., 2024). Figure 1 represents the various pathways where metformin influence on gut microbes.
Epigenetic pathways
The beneficial effects of metformin on gut microbiota extend beyond the gut, influencing systemic health through various mechanisms. Among these, its ability to modulate epigenetic pathways, particularly those involved in inflammation and metabolism, has gained significant attention. Epigenetic pathways are biological mechanisms that regulate gene expression without altering the underlying DNA sequence. These pathways involve chemical modifications to DNA, such as methylation, or to histone proteins, which compact and organize DNA in the nucleus, affecting gene accessibility (Handyet al., 2011). These modifications can activate or deactivate genes, depending on the type and location of the modification. The main mechanisms include DNA methylation, which typically silences gene expression by adding methyl groups to cytosine residues and histone modifications, such as acetylation, which generally promotes gene expression by loosening chromatin structure.
Influence on DNA methylation and Histone Acetylation
Change in SCFAs through Epigenetic effects
Metformin reshapes the gut microbiota, particularly promoting the growth of beneficial bacteria such as Akkermansia muciniphila and Bifidobacterium species. These bacteria produce Short-Chain Fatty Acids (SCFAs), including acetate, propionate and butyrate, through the fermentation of dietary fibers. By increasing the abundance of SCFA-producing bacteria, metformin enhances Fiber fermentation, leading to greater SCFA production, which contributes to its metabolic and anti-inflammatory effects (Hermanet al., 2022). SCFAs themselves are also known to influence epigenetic pathways, particularly through inhibiting HDACs, which leads to increased histone acetylation and anti-inflammatory gene expression. Additionally, bacterial communities in the gut directly influence epigenetic reprogramming via DNA methylation, further affecting host metabolism. This interaction between the gut microbiota and epigenetic changes creates a feedback loop, where changes in gene expression can influence microbial composition and vice versa.
Epigenetic impact on inflammation
Histone modification reduces inflammation by decreasing the expression of inflammatory mediators such as TNF-α and IL-1β. A less inflammatory gut environment supports the growth of beneficial microbes, while reducing pro-inflammatory bacteria and the increase in SCFAs helps maintain intestinal pH.
Impact of SCFAs on Neuroprotection
SCFAs not only act locally in the gut but can also cross the blood-brain barrier, where they impact brain health by inhibiting HDACs and reducing neuroinflammation. SCFAs can also inhibit HDACs and reduce NF-κB activity in microglia, contributing to neuroprotection and the suppression of neuroinflammatory responses and metformin is known to inhibit NF-κB through AMPK activation, further promoting neuroprotection. These epigenetic modifications continue to suppress inflammation in the brain, providing protection against neurodegeneration (Sharma et al., 2020).
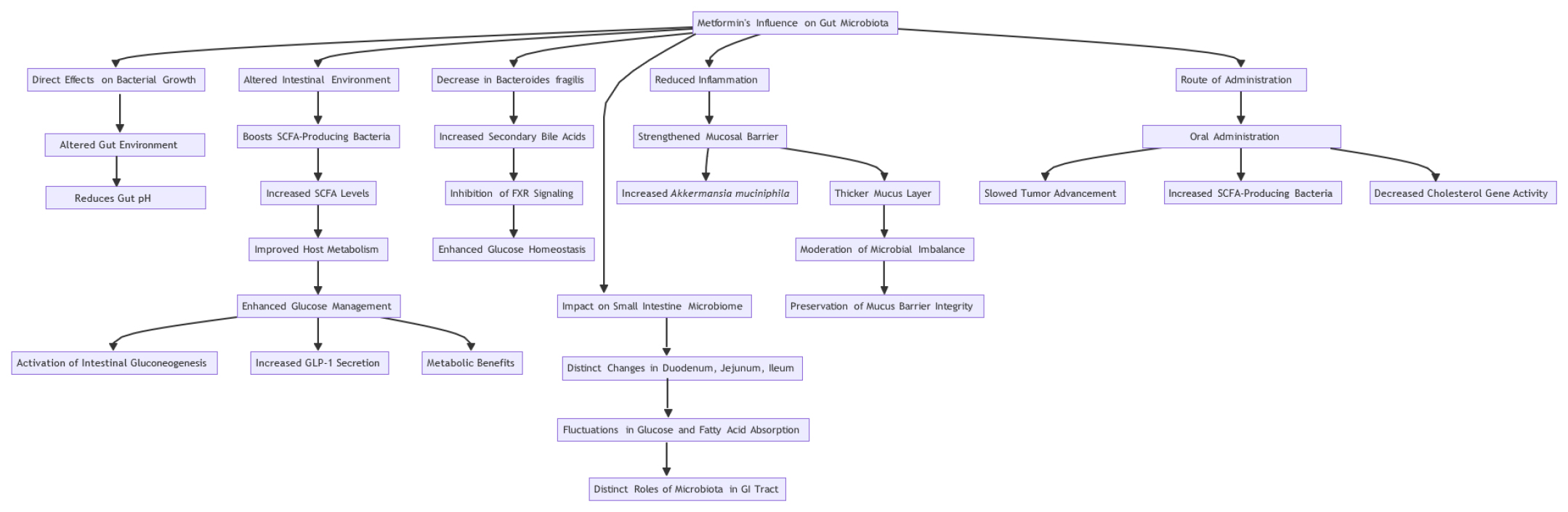
Figure 1:
Metformin influence on gut microbes.
Regulation of gene Expression by Non-coding RNAs
The regulation of gene expression in epigenetic mechanism by non-coding RNAs (e.g., miRNAs), are also affected by metformin. MiRNAs play a crucial role in fine-tuning gene expression (Hsuet al., 2021) and metformin has been shown to modulate the levels of several miRNAs involved in inflammation, metabolism and cellular stress responses, contributing to its broad-spectrum therapeutic effects. Figure 2 represents the metformin modulation of epigenetic and gut microbes pathways
Metformin Influences Neuroimmune Pathways Through Microbial Modulation
Influence on Gut-Brain Axis (GBA) and immune cells
The GBA serves as a crucial communication network linking the CNS and the gastrointestinal tract. This intricate system encompasses a variety of interactions between the enteric nervous system, autonomic nervous system, immune system, gut microbiota and neuroendocrine signaling pathways. Recent studies highlight the role of metformin in regulating immune cell activation by modulating gut immune cells, such as macrophages and dendritic cells, which subsequently leads to cytokine production (Gurtan and Sharp, 2013). The activation of peripheral immune cells has the potential to traverse the BBB, contributing to neuroinflammation. Metformin has been shown to mitigate neuroinflammation through its modulatory effects on these immune cells. Immune cells, particularly intestinal macrophages and regulatory T cells play pivotal roles in maintaining gut health and providing protection against inflammation. Metformin’s ability to influence the composition of gut microbiota, including modulation of Akkermansia muciniphila and other beneficial microbes, underscores its potential in reducing neuroinflammation and promoting overall gut-brain health. Several neurodegenerative diseases, including AD and MS, have been linked to immune responses that are driven by gut microbiota (Linet al., 2023).
Multiple Sclerosis (MS) and gut Dysbiosis
MS, as an autoimmune condition, is often characterized by gut dysbiosis and systemic inflammation. In patients with MS, inflammation originating from the gut can migrate to the brain, leading to increased neuroinflammation. Research indicates that gut microbes may trigger T-cell activation, which heightens the autoimmune assault on the myelin sheath (Tiwariet al., 2023). Notably, decreased levels of Faecalibacterium prausnitzii are associated with heightened inflammation, suggesting a link between gut microbial composition and inflammatory responses (Hoffmanet al., 2023).
Alzheimer Disease (AD) and gut Microbiota
Cognitive decline and memory impairment observed in AD are primarily attributed to the presence of amyloid beta plaques and tau protein tangles within the brain. Gut microbiota significantly influence systemic inflammation and chronic inflammation stemming from disrupted gut microbiomes can adversely affect the brain’s immune cells (Zhouet al., 2022), especially microglia, thereby contributing to the accumulation of amyloid plaques and tau tangles (Chaudhryet al., 2023). SCFAs, including butyrate and propionate produced by beneficial gut bacteria (Chenet al., 2015), are recognized for their neuroprotective properties, including the reduction of neuroinflammation and enhancement of brain health. In the context of AD pathology, a leaky gut (Hansenet al., 2018) allows inflammatory mediators, such as lipopolysaccharides, to penetrate the BBB, exacerbating inflammation within the brain. Alterations in gut microbiota characterized by a reduction in Bifidobacterium and Lactobacillus, alongside an increase in pathogenic bacteria such as Proteobacteria, are correlated with elevated systemic and neuroinflammation.
Linking Metabolic Health through microbial modulation
Gut microbiota and metabolic health
Akkermansia muciniphila and Bifidobacterium, known for enhancing gut barrier function and reducing inflammation, play a vital role in metabolic health. Pro-inflammatory cytokines such as TNF-α and IL-6 are linked to insulin resistance, obesity and other metabolic disorders. By reducing these markers, inflammation is lessened, thereby improving overall metabolic health (Krishaa et al., 2023).
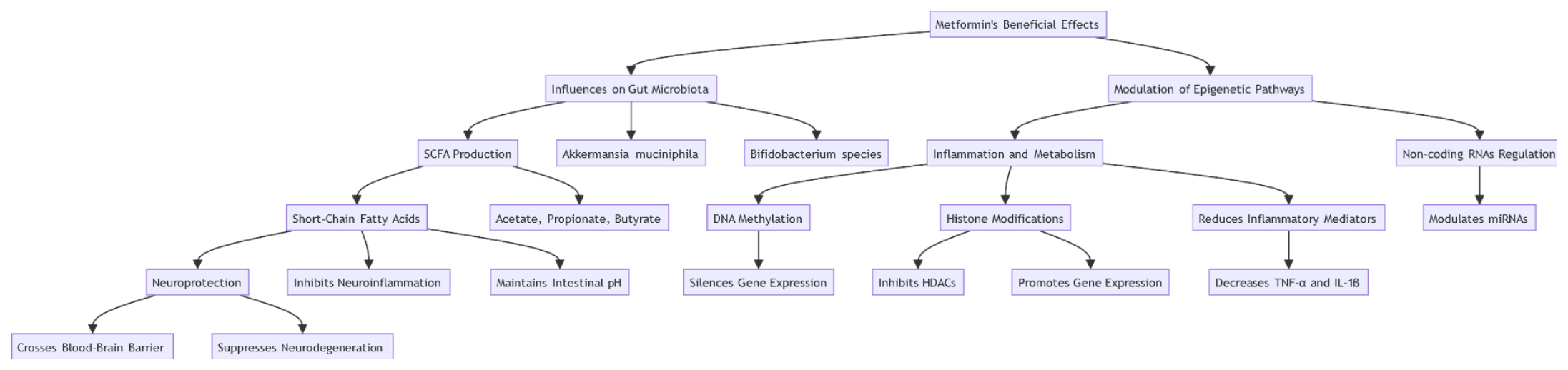
Figure 2:
Metformin modulation of epigenetic pathways.
Bile acid metabolism and Gut-Derived hormones
Metformin has been shown to influence bile acid metabolism through its effects on gut microbiota, impacting bile acid receptors like FXR, which are involved in glucose and lipid metabolism. Regulating these receptors, metformin may improve lipid profiles and insulin sensitivity. It also supports glucose metabolism by increasing the secretion of gut-derived hormones like GLP-1, which enhances insulin secretion (Isopet al., 2023).
Indole derivatives and Insulin sensitivity
Recent studies have linked indole derivatives, produced by gut bacteria via tryptophan metabolism, to improved insulin sensitivity and reduced inflammation. Metformin may enhance or inhibit specific bacterial pathways involved in indole production. Moreover, bacterial enzymes like β-glucuronidase could influence metformin’s bioavailability by altering its pharmacokinetics in the gut, suggesting that metformin’s interaction with gut microbes may vary between individuals depending on their unique microbial enzyme activity (Hyunet al., 2013).
Horizontal gene Transfer and Microbial Ecosystem
Furthermore, metformin may facilitate HGT among microbial species, allowing certain bacteria to acquire genetic traits that enhance carbohydrate metabolism or promote antibiotic resistance. This selective pressure could inadvertently lead to the development of microbial ecosystems that regulate glucose homeostasis (Chenget al., 2024) and lipid metabolism more effectively.
Metformin Role in Meta-Metabolism
Metformin’s influence may extend to creating an integrated meta-metabolism, wherein microbial metabolic activities synergize with the host’s cells, resulting in a more efficient metabolic system. Moreover, metformin might indirectly affect bacteriophages -viruses that infect bacteria-by altering the gut environment in ways that either promote or suppress bacteriophage activity, leading to shifts in bacterial populations that enhance metabolic health (Hannachi and Camoin-Jau, 2021).
Linking Cognitive Health through microbial modulation
SCFAs and Neuroinflammation
Metformin’s modulation of gut microbiota promotes the growth of beneficial bacteria that produce metabolites like SCFAs, particularly butyrate. Butyrate crosses the BBB (Hsuet al., 2021) and inhibits pro-inflammatory pathways in the brain, potentially reducing chronic neuroinflammation associated with neurodegenerative diseases such as Alzheimer’s and Parkinson’s.
This reduction in neuroinflammation helps protect cognitive function (Krishaa et al., 2023).
Neurotransmitters and Cognitive Function
Gut bacteria produce neurotransmitters like serotonin, dopamine and GABA, (Dicks, 2022) which are essential for cognitive functions like memory, learning and mood regulation. Metformin encourages the growth of bacteria, such as Lactobacillus and Bifidobacterium, that enhance the production of these neurotransmitters, improving mood, reducing anxiety and positively impacting cognitive performance (Rosell-Díaz and Fernández-Real, 2024).
Gut Microbiota and BBB integrity
Gut microbiota also influence the permeability of the BBB, which protects the brain from harmful substances. A weakened BBB is linked to cognitive decline and neurodegenerative disorders. By enhancing the production of SCFAs like butyrate, metformin may strengthen the BBB. Furthermore, metformin affects BDNF, a protein crucial for neurogenesis and synaptic plasticity. Higher BDNF levels are associated with improved memory and learning and metformin’s effect on gut microbiota may increase SCFA production, indirectly boosting BDNF levels and enhancing cognitive performance (Fanget al., 2020).
MicroRNAs and Brain Gene Expression
miRNAs produced by gut microbes may be transported to the brain, where they can regulate gene expression in neurons. Metformin’s effect on gut bacteria could influence the release of these microbial miRNAs, altering brain cell function (Gurtan and Sharp, 2013). The gut mycobiome, comprising fungi like Candida and Saccharomyces, also interacts with bacteria to influence cognitive health and metformin may shift the balance of these fungal populations, affecting neuroactive metabolite production. Gut microbiota exhibit circadian rhythms (Huet al., 2022), which impact metabolic and cognitive processes like memory and attention. Metformin may help regulate these microbial fluctuations to optimize cognitive performance (Liet al., 2018).
Cognitive function through Bacterial Communication
Extracellular Vesicles (EVs) released by gut microbes carry bioactive molecules such as proteins and lipids to the brain and metformin may alter the production or content of these EVs, affecting brain inflammation, synaptic activity, or neurogenesis. The quorum-sensing mechanisms used by bacteria to communicate and coordinate activity may also have an impact on cognitive function. Metformin might influence these bacterial communication pathways, leading to changes in the release of neuroactive compounds that affect mood, cognitive flexibility, or even creativity (Xianget al., 2024).
Metformin and Glymphatic system
Metformin’s effects on gut microbiota may also indirectly influence the glymphatic system, which clears waste from the brain, potentially enhancing the removal of toxic proteins like amyloid-beta and improving cognitive function. Gut bacteria interact with the brain’s suprachiasmatic nucleus, which regulates circadian rhythms and produce gasotransmitters like hydrogen sulfide and nitric oxide that act as signaling agents in both the gut and brain. (Gheibiet al., 2020) Metformin may modulate the production of these gases, influencing brain neurotransmission and neuroplasticity.
Future Prospectives
Investigation of the molecular mechanisms by which metformin alters gut microbiome composition and its subsequent impact on metabolic pathways. Additionally, assessing individual differences in gut microbiota profiles could help predict therapeutic outcomes. Longitudinal studies are needed to evaluate the long-term effects of metformin on cognitive health, particularly among populations at risk for neurodegenerative diseases. Furthermore, exploring interactions between metformin and dietary components may enhance its therapeutic effects, while examining the role of non-coding RNAs can provide insights into mediating inflammation and metabolism. Research should also delve into gut-brain axis dynamics influenced by microbial metabolites and investigate epigenetic changes induced by metformin. The potential role of bacteriophages in gut microbiota modulation warrants further exploration, alongside the development of targeted therapies that replicate metformin’s benefits. Finally, conducting clinical trials across diverse populations will optimize treatment strategies and help determine the generalizability of metformin’s effects.
CONCLUSION
Metformin has demonstrated a multitude of beneficial properties through its modulation of gut microbes, influencing various pathways that are pivotal for both metabolic and cognitive health. Clinical research has yielded positive results for several mechanisms, A including the enhancement of beneficial microbial populations, which in turn may influence Short-Chain Fatty Acid (SCFA) production and improve insulin sensitivity. These interactions highlight the potential of metformin in harnessing gut microbiota to modulate epigenetic pathways, affecting gene expression related to metabolic functions and inflammation. Moreover, the neuroimmune pathways activated by metformin suggest a promising link between gut health and cognitive function. By reducing neuroinflammation and enhancing neuroprotection, metformin may offer therapeutic benefits for neurological conditions, potentially influencing cognitive outcomes in affected individuals. However, numerous aspects of metformin’s effects remain to be explored, necessitating further investigation into specific mechanisms and hypotheses. Both animal and human studies should be prioritized to address these unexplored dimensions of gut microbial modulation and its implications for metabolic and cognitive health. Although metformin’s metabolic functions are well-established in clinical practice, its neurological effects require more extensive research before being fully integrated into standard treatment protocols. Continued investigation in this area is crucial to unlocking the full potential of metformin in promoting comprehensive health outcomes, thereby paving the way for innovative therapeutic strategies targeting metabolic and cognitive disorders.
Cite this article:
Musharraf SS, Fayaz SM, Priyadharshini A. The Impact of Metformin on Neurological and Metabolic Function through Gut Microbiota. J Young Pharm. 2025;17(2):292-8.
ACKNOWLEDGEMENT
The authors sincerely thank the management of SRM College of Pharmacy, SRMIST for providing the necessary facilities.
ABBREVIATIONS
SCFAs | Short-chain fatty acids |
---|---|
DNA | Deoxyribonucleic acid |
NAFLD | Non-alcoholic fatty liver disease |
AMPK | Adenosine monophosphate-activated protein kinase |
PCOS | Polycystic ovary syndrome |
OCT 1 | Organic Cation Transporter |
1 T2DM | Type 2 diabetes mellitus |
GLP-1 | Glucagon-Like Peptide-1 |
GUDCA | Glycoursodeoxycholic acid |
FXR | Farnesoid X Receptor |
HDACs | Histone deacetylases |
TNF-α | Tumor Necrosis Factor-alpha |
IL-1β | Interleukin-1 beta |
pH | Potential of Hydrogen |
NF-κB | Nuclear Factor kappa-light-chain-enhancer of activated B cells |
RNAs | Ribonucleic Acids |
MiRNAs | micro Ribonucleic Acids |
GBA | Gut brain axis |
CNS | Central nervous system |
BBB | Blood brain barrier |
AD | Alzheimer’s disease |
MS | Multiple sclerosis |
GABA | Gamma-aminobutyric acid |
HGT | Horizontal gene transfer |
BDNF | Brain-derived neurotrophic factor |
EVs | Extracellular vesicles |
NSCs | Neural stem cells |
References
- Broadfield LA, Saigal A, Szamosi JC, Hammill JA, Bezverbnaya K, Wang D, Gautam J, Tsakiridis EE, Di Pastena F, McNicol J, Wu J, Syed S, Lally JSV, Raphenya AR, Blouin M-J, Pollak M, Sacconi A, Blandino G, McArthur AG, Steinberg GR, et al. Metformin-induced reductions in tumor growth involves modulation of the gut microbiome. Molecular Metabolism. 2022;61 [CrossRef] | [Google Scholar]
- Bu Y, Peng M, Tang X, Xu X, Wu Y, Chen AF, Yang X, et al. Protective effects of metformin in various cardiovascular diseases: Clinical evidence and AMPK-dependent mechanisms. Journal of Cellular and Molecular Medicine. 2022;26(19):4886-4903. [CrossRef] | [Google Scholar]
- Chaudhry TS, Senapati SG, Gadam S, Mannam HPSS, Voruganti HV, Abbasi Z, Abhinav T, Challa AB, Pallipamu N, Bheemisetty N, Arunachalam SP, et al. The impact of microbiota on the gut–brain axis: Examining the complex interplay and implications. Journal of Clinical Medicine. 2023;12(16):5231 [CrossRef] | [Google Scholar]
- Chen HP, Zhao YT, Zhao TC. Histone deacetylases and mechanisms of regulation of gene expression. Critical Reviews in Oncogenesis. 2015;20(1–2):35-47. [CrossRef] | [Google Scholar]
- Cheng M, Ren L, Jia X, Wang J, Cong B. Understanding the action mechanisms of metformin in the gastrointestinal tract. Frontiers in Pharmacology. 2024;15 [CrossRef] | [Google Scholar]
- Dicks LMT. Gut bacteria and neurotransmitters. Microorganisms. 2022;10(9):1838 [CrossRef] | [Google Scholar]
- Fang W, Zhang J, Hong L, Huang W, Dai X, Ye Q, Chen X, et al. Metformin ameliorates stress-induced depression-like behaviors via enhancing the expression of BDNF by activating AMPK/CREB-mediated histone acetylation. Journal of Affective Disorders. 2020;260:302-313. [CrossRef] | [Google Scholar]
- Gheibi S, Samsonov AP, Gheibi S, Vazquez AB, Kashfi K. Regulation of carbohydrate metabolism by nitric oxide and hydrogen sulfide: Implications in diabetes. Biochemical Pharmacology. 2020;176 [CrossRef] | [Google Scholar]
- Gurtan AM, Sharp PA. The role of miRNAs in regulating gene expression networks. Journal of Molecular Biology. 2013;425(19):3582-3600. [CrossRef] | [Google Scholar]
- Handy DE, Castro R, Loscalzo J. Epigenetic modifications: Basic mechanisms and role in cardiovascular disease. Circulation. 2011;123(19):2145-2156. [CrossRef] | [Google Scholar]
- Hannachi N, Camoin-Jau L. Drug response diversity: A hidden bacterium?. Journal of Personalized Medicine. 2021;11(5):345 [CrossRef] | [Google Scholar]
- Hansen DV, Hanson JE, Sheng M. Microglia in Alzheimer’s disease. The Journal of Cell Biology. 2018;217(2):459-472. [CrossRef] | [Google Scholar]
- Herman R, Kravos NA, Jensterle M, Janež A, Dolžan V. Metformin and insulin resistance: A review of the underlying mechanisms behind changes in GLUT4-mediated glucose transport. International Journal of Molecular Sciences. 2022;23(3):1264 [CrossRef] | [Google Scholar]
- Hoffman K, Brownell Z, Doyle WJ, Ochoa-Repáraz J. The immunomodulatory roles of the gut microbiome in autoimmune diseases of the central nervous system: Multiple sclerosis as a model. Journal of Autoimmunity. 2023;137 [CrossRef] | [Google Scholar]
- Hsu S-K, Cheng K-C, Mgbeahuruike MO, Lin Y-H, Wu C-Y, Wang HD, Yen C-H, Chiu C-C, Sheu S-J, et al. New insight into the effects of metformin on diabetic retinopathy, aging and cancer: Nonapoptotic cell death, immunosuppression and effects beyond the AMPK pathway. International Journal of Molecular Sciences. 2021;22(17):9453 [CrossRef] | [Google Scholar]
- Hu Y, Zhou Y, Yang Y, Tang H, Si Y, Chen Z, Shi Y, Fang H, et al. Metformin protects against diabetes-induced cognitive dysfunction by inhibiting mitochondrial fission protein DRP1. Frontiers in Pharmacology. 2022;13 [CrossRef] | [Google Scholar]
- Hyun B, Shin S, Lee A, Lee S, Song Y, Ha N-J, Cho K-H, Kim K, et al. Metformin down-regulates TNF-α secretion via suppression of scavenger receptors in macrophages. Immune Network. 2013;13(4):123-132. [CrossRef] | [Google Scholar]
- Isop LM, Neculau AE, Necula RD, Kakucs C, Moga MA, Dima L, et al. Metformin: The winding path from understanding its molecular mechanisms to proving therapeutic benefits in neurodegenerative disorders. Pharmaceuticals. 2023;16(12):1714 [CrossRef] | [Google Scholar]
- Ke H, Li F, Deng W, Li Z, Wang S, Lv P, Chen Y, et al. Metformin exerts anti-inflammatory and mucus barrier protective effects by enriching Akkermansia muciniphila in mice with ulcerative colitis. Frontiers in Pharmacology. 2021;12 [CrossRef] | [Google Scholar]
- Kim JJ. Update on polycystic ovary syndrome. Clinical and Experimental Reproductive Medicine. 2021;48(3):194-197. [CrossRef] | [Google Scholar]
- L K, Ng TKS, Wee HN, Ching J. Gut-brain axis through the lens of gut microbiota and their relationships with Alzheimer’s disease pathology: Review and recommendations. Mechanisms of Ageing and Development. 2023;211 [CrossRef] | [Google Scholar]
- Lee CB, Chae SU, Jo SJ, Jerng UM, Bae SK. The relationship between the gut microbiome and metformin as a key for treating type 2 diabetes mellitus. International Journal of Molecular Sciences. 2021;22(7):3566 [CrossRef] | [Google Scholar]
- Li Y, Hao Y, Fan F, Zhang B. The role of microbiome in insomnia, circadian disturbance and depression. Frontiers in Psychiatry. 2018;9:669 [CrossRef] | [Google Scholar]
- Lin H, Ao H, Guo G, Liu M. The role and mechanism of metformin in inflammatory diseases. Journal of Inflammation Research. 2023;16:5545-5564. [CrossRef] | [Google Scholar]
- Lv Z, Guo Y. Metformin and its benefits for various diseases. Frontiers in Endocrinology. 2020;11:191 [CrossRef] | [Google Scholar]
- Malik F, Mehdi SF, Ali H, Patel P, Basharat A, Kumar A, Ashok F, Stein J, Brima W, Malhotra P, Roth J, et al. Is metformin poised for a second career as an antimicrobial?. Diabetes/Metabolism Research and Reviews. 2018;34(4) [CrossRef] | [Google Scholar]
- Mueller NT, Differding MK, Zhang M, Maruthur NM, Juraschek SP, Miller ER, Appel LJ, Yeh H-C, et al. Metformin affects gut microbiome composition and function and circulating short-chain fatty acids: A randomized trial. Diabetes Care. 2021;44(7):1462-1471. [CrossRef] | [Google Scholar]
- Paschou SA, Shalit A, Gerontiti E, Athanasiadou KI, Kalampokas T, Psaltopoulou T, Lambrinoudaki I, Anastasiou E, Wolffenbuttel BHR, Goulis DG, et al. Efficacy and safety of metformin during pregnancy: An update. Endocrine. 2024;83(2):259-269. [CrossRef] | [Google Scholar]
- Rosell-Díaz M, Fernández-Real JM. Metformin, cognitive function and changes in the gut microbiome. Endocrine Reviews. 2024;45(2):210-226. [CrossRef] | [Google Scholar]
- Sharma M, Li Y, Stoll ML, Tollefsbol TO. The epigenetic connection between the gut microbiome in obesity and diabetes. Frontiers in Genetics. 2019;10:1329 [CrossRef] | [Google Scholar]
- Sun L, Xie C, Wang G, Wu Y, Wu Q, Wang X, Liu J, Deng Y, Xia J, Chen B, Zhang S, Yun C, Lian G, Zhang X, Zhang H, Bisson WH, Shi J, Gao X, Ge P, Jiang C, et al. Gut microbiota and intestinal FXR mediate the clinical benefits of metformin. Nature Medicine. 2018;24(12):1919-1929. [CrossRef] | [Google Scholar]
- Tiwari P, Dwivedi R, Bansal M, Tripathi M, Dada R. Role of gut microbiota in neurological disorders and its therapeutic significance. Journal of Clinical Medicine. 2023;12(4):1650 [CrossRef] | [Google Scholar]
- Xiang M, Liu Y, Guo Q, Liao C, Xiao L, Xiang M, Guan X, Liu J, et al. Metformin enhances the therapeutic effects of extracellular vesicles derived from human periodontal ligament stem cells on periodontitis. Scientific Reports. 2024;14(1) [CrossRef] | [Google Scholar]
- Zhou X, Baumann R, Gao X, Mendoza M, Singh S, Katz Sand I, Xia Z, Cox LM, Chitnis T, Yoon H, Moles L, Caillier SJ, Santaniello A, Ackermann G, Harroud A, Lincoln R, Gomez R, González Peña A, Digga E, Baranzini SE, et al. Gut microbiome of multiple sclerosis patients and paired household healthy controls reveal associations with disease risk and course. Cell. 2022;185(19) [CrossRef] | [Google Scholar]