ABSTRACT
Nanosponges (NSs) are a novel class of nanomaterials that have garnered substantial interest due to their unique properties and potential applications in drug delivery. The primary objective of this review is to provide the basics of nanosponge-based drug delivery systems, preparation techniques, and evaluation methods. It also covers associated aspects like challenges phased in industry and research in the manufacturing of NSs. NSs are tiny, mesh-like structures that can be loaded with drugs to improve solubility, stability, extended release, and bioavailability across various dosage forms. They can encapsulate a wide range of substances, including proteins, enzymes, hydrophilic and lipophilic chemicals, vaccines, and antibodies, and can be made from either inorganic or organic materials. NSs offer several advantages, such as compatibility, sterility, cost-effectiveness, extended release, better stability, reduced side effects, versatility, taste masking, and formulation enhancement. The review also elucidates different types of NS, their preparation methods, prospective applications, and evaluation processes. Overall, NSs have demonstrated considerable promise, with the potential to transform nanomedicine.
INTRODUCTION
Nanosponges (NSs) are insoluble, high-absorption materials with a virus-like structure, allowing drugs to be loaded into them and directed to tumor cells. They can be synthesized by varying crosslinker ratios and are suitable for encapsulating proteins, enzymes, compounds, vaccines, and antibodies. NS can harden compounds and mask taste, making it 5 times better for breast cancer drugs than conventional methods and treating various diseases (Mahalekshmiet al., 2023). NS, a type of nanoparticle, can be regenerated through light heating, inert gases, solvents, and pH adjustments, with applications in flower culture, flame retardancy, pharmaceuticals, and cosmetics. NS, a type of nanoparticle, can be regenerated through light heating, inert gases, solvents, and pH adjustments, with applications in flower culture, flame retardancy, pharmaceuticals, and cosmetics (Shivani and Kumar Poladi, 2015). Poly(isobutyl-cyanoacrylate) nanocrystals with hydrophilic cores absorb medicinal molecules via electrostatic contact, offering higher entrapment efficiency and resistance to molecular degradation (Keriloset al., 2024).
NS, a 3D structure that can absorb liquid, is easy to create, but better at entrapping small molecules. Its crystallization degree affects drug loading, making it a potential drug delivery system for tablets or capsules (Shringirishiet al., 2014). The morphology of nanosponges is shown in Figure 1.
ADVANTAGES
Compatibility and Sterility: NS are compatible with many excipients and carriers. They are self-sterilizing due to 0.25 μM pore size, no microbial penetration (Bhowmiket al., 2018).
Cost Effective and Free Flowing: These can be inexpensive and free flowing.
Extended Release: NS can release for up to 12 hr, more flexibility and elegance to the formulation.
Better Stability and Less Side Effects: These topical oil control products offer lower doses, improved stability, and reduced side effects, while also modifying drug release profiles for improved systemic exposure.
Versatility: NS are versatile, can create new product forms and enhance physical, chemical and thermal stability of formulations.
Taste Masking and Formulation Improvement: NS can mask the unwanted taste and turn immiscible liquids into solid dosage forms, increase solubility and stability for poorly water-soluble drugs.
Biodegradable and non-toxic: The NS is both biodegradable and non-toxic, though totally free from allergens, mutagens and irritants.
Better products: The Carries drug in system to improve the therapeutic index and the duration of action is a better product.
Delivery: One can deliver hydrophilic and lipophilic substances through it, delay release from it, while increasing its absorption and solubility (Sultana, 2024).
DISADVANTAGES
Loading Capacity Variability: NS work depending on loading capacity and dose release rate.
Size Limitation: NS are better for smaller molecules than larger ones (Agrawalet al., 2020).
Crystallization Impact: Crystallization in NS affects load bearing capacity and overall performance.
Para-Crystalline Variability: Different para-crystalline forms of NS have different loading capacities (Shindeet al., 2024).
TYPES OF NANOSPONGE
NS can be developed into various types based on polymer, concentration, and production method. Cyclomaltoheptaose-based NS is a common type. Metal Organic Frameworks (MOFs) offer flexibility in design and synthesis, using methods like deployment, precipitation gelation, electrochemical deposition, and solvothermal gelation (Annammadevi and Anusha, 2022). Figure 2 shows the classification of nanosponges.
Monometallic nanosponge
It only contains one metal precursor; this type of metal NS is the most fundamental. In nanoporous applications, the first monometallic oxide has been synthesized in year of 1995, Antonelli and Ying reported on the invention of TiO2, utilizing alkyl (trimethyl) bromide as a 3 nm pore surfactant during production. However, several metals, including copper, silver, golden, platinum, and palladium, are shown on the template-free single-metal NS.
Bimetallic nanosponge
Bimetal NS presents stability, diverse pores, and exceptional catalytic activity. Due to its availability and lower cost, Pd is the preferred choice. Researchers have successfully developed a three-dimensional, highly porous Pd-Cu bimetallic NS alloy using Al (NO3) for improved electrocatalytic activity. Effective Al extraction is critical for attaining the necessary porosity and catalytic activity during the synthesis process (Kaur and Kumar 2019).
Polymetallic nanosponge
In recent decades, the focus has shifted towards creating polymetal Nanostructures due to improvements in catalytic activity. These NS have potential applications in fuel cells, water purification, and catalysis. NaBH4 plays a role in producing template-free trimetal NS of Pt53Ru39Ni8, with hydrogen bubbles serving as a dynamic template. Polymetal NS have higher catalytic activity than bimetalic NS (Biswaset al., 2016).
Metal Oxide nanosponge
Organic plastics including surfactant, starches, and polysaccharides are included in the synthesis, along with inorganic plastics like zeolite and alumina. These plastics are then utilized to replace or remove the template or to employ strong acids and foundations. Two types of template-based techniques are needed to synthesize the metal oxide NS.
Nanocasting nanosponge
Hard template approach uses strong, porous materials like polystyrene latex, zeolite, silica, alumina, and copolymer block as support materials. The frame is filled with metal ions, and extracted using methods like calcination, acid treatment, and basic treatment. This technology is recommended for its ease of use and adaptability, allowing architecture expansion into various forms, sizes, and lengths.
Endotemplate nanosponge
The soft template solution is a flexible framework made of cellulose-based components, polysaccharides, and surfactants. Surfactants create mesopore or micropore-functioning micelles, allowing for modification of nanostructure shape, size, and content. This technique was used to create mesostructured metal oxides in 1994. It is less expensive, easier to synthesize, and fairly successful compared to hard templates.
MATERIALS USED IN THE PREPARATION OF NANOSPONGE
Chemicals used in synthesizing NS depend on the type and extent of crosslinking, which affects drug release and encapsulation patterns and is influenced by the concentration of crosslinkers used (Tiwari and Bhattacharya, 2022). CDs combine with active pharmaceuticals to form complexes, increasing water solubility, concealing undesirable qualities, reducing adverse effects, and enhancing photographic stability. CD-based nanocarriers have high drug-loading capacity (Buradet al., 2023). Various materials used in the preparation of nanosponges are given in Figure 3 (Raoet al., 2013) (Ghurghure et al., 2018).
METHODS OF PREPARATION OF NANOSPONGE
Hypercrossed linked β-CD method
Hyper-crosslinked β-CD NS are synthesized from the reaction between the β-CD of any type with a variety of cross-linking reagents, such as epichlorohydrin, diphenyl carbonate, or citric acid, in any polar or nonpolar solvents, like water, Dimethylformamide, or Dimethyl sulfoxide. The formation between 3D β-CD molecules is through covalent bonds. The product is washed, purified, and dried as NS characterized by high surface area.
Ultrasound-assisted synthesis of nanosponge
This method makes small, round NS of the same size. It does this by mixing a polymer and a crosslinker in a flask, heating it to 90°C, and breaking it into smaller pieces. The product is rinsed, cleaned using Soxhlet extraction with ethanol, and dried in a vacuum. These NS are good for delivering drugs and soaking up other substances (Thakreet al., 2016). Pictorially the method has been explained in Figure 4.
Solvent method of nanosponge preparation
The method (Figure 5) involves using dimethylacetamide and acetonitrile as solvents, combining them with a polymer, and optimizing the crosslinker/polymer ratio. The mixture is then heated for 48 hr, cooled, and a surplus of bi-distilled water is added to extract the product. The product is vacuum-filtered and recovered (Yadavet al., 2014).
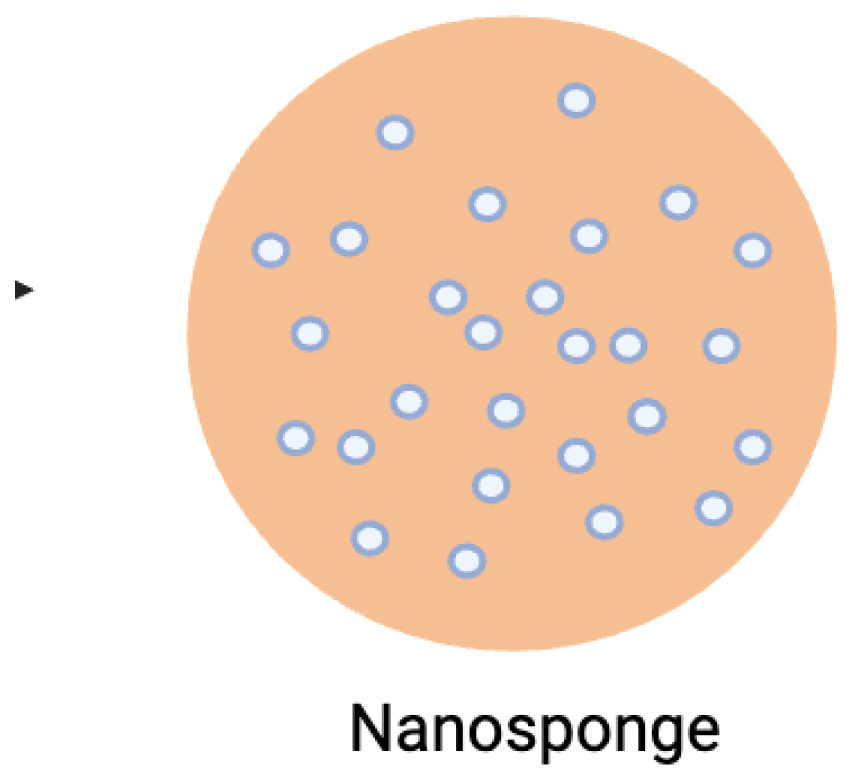
Figure 1:
Morphology of nanosponges.
Melt method of nanosponge preparation
The melt procedure involves melting both β-CDs and the crosslinker. After finely combining the remaining components, put them to a 250 mL jar that has been heated to 100°C. Following that, the reaction is performed for 5 hr using attractive magnetic mixing. Allow to cool and break down, then wash with solvents to remove byproducts and excess (Poonamet al., 2023).
Microwave synthesis of nanosponge preparation
In the microwave synthesis approach, this polymer, along with crosslinkers, can be mixed in an appropriate solvent. The mixture is then exposed to microwave irradiation, which rapidly heats up the components and accelerates the cross-linking process, developing a porous three-dimensional NS structure. The product then undergoes purification by washing and can be dried in a vacuum. This method will, therefore, provide faster, energy-efficient synthesis of uniform NS with a higher surface area for different applications.
Co-solvent evaporation method of nanosponge preparation
To create an inner phase, the NS was prepared using polymers and Eudragit RS 100, a solvent. The medication was dissolved at 35°C and mixed with PVA’s exterior phase, acting as an emulsifier. The solution was stirred for 3 hr at ambient temperature and dried in a hot-air oven at 40°C for 12 hr (Figure 6).
Solvent displacement method of nanosponge preparation
In the solvent displacement method different ratios of Ethyl Cellulose (EC) and Polyvinyl Alcohol (PVA) are employed. PVA is introduced into the aqueous solution, followed by dissolving the medication and polymer in chloroform, stirring at 1000-1500 rpm, and drying at 40°C in a hot air oven (Figure 7).
Bubble electrospinning method
Solvent evaporation method
NS is produced by dissolving EC in dichloromethane and mixing it with PVA aqueous solution. Magnetic mixing extends the reaction for 5 hr, followed by filtering and drying in an oven at 40°C for 24 hr (Chilajwaret al., 2014). Table 1 shows the Characteristics of NS Prepared by Various Methods.
Manufacturing Method | Particle Size (nm) | Advantages | Disadvantages |
---|---|---|---|
Hyper crosslinked Method | 350-500 | Customizable properties (porosity, rigidity).High porosity for enhanced drug loading.Superior thermal and chemical stability. | Time-consuming process requiring precise control.High production cost due to specialized crosslinkers.Potential toxicity of crosslinking agents. |
Ultrasound-assisted Synthesis | 97-325 | Energy-efficient compared to thermal methods.Faster synthesis process.Improved particle dispersion and uniformity. | Risk of overheating, which may alter structure.Expensive ultrasonic equipment required.Difficult to scale up for large-scale production. |
Solvent Method | 316-911 | Scalable for industrial production.Simple and straightforward synthesis.Customizable solvent choice to modify properties. | Energy-intensive due to solvent removal and purification steps.Use of hazardous solvents like dimethylformamide.Solvent residues may affect product safety. |
Co-solvent evaporation method | 105-842 | Uniform particle size.High drug loading efficiency.Simple, cost-effective process. | Batch-to-batch variability due to manual solvent evaporation.Limited to hydrophobic drugs.Residual solvents may remain, affecting safety. |
Microwave Method | 153-400 | Uniform heating, leading to more uniform Nanosponge size.Faster reaction times (minutes vs. hours).Energy-efficient synthesis. | Risk of uneven heating in larger batches.Requires specialized microwave reactors.Limited scalability for large-scale production. |
Challenges are currently being addressed in research and industrial settings
In both research and industrial environments, several innovative techniques are being explored to overcome the problems related to the manufacturing of NS. In the case of the solvent evaporation method, slow solvent removal and contamination with residual solvents are overcome by incorporating supercritical fluid technology to remove solvents quickly and efficiently and also by using microwave-assisted solvent evaporation to offer uniform heating and minimize processing time. Further, environmentally friendly, low-toxicity solvents or even solvent-free methods are being researched to reduce environmental impact and increase safety. In the solvent displacement method, uniform dispersion of NP and control of size are the problems overcome by optimizing the concentration of polymer and types of surfactants, and employing high-shear mixing and ultrasonication techniques for better consistency. The sonication method suffers from heat generation and uneven crosslinking; however, these are mitigated by optimizing sonication parameters, using cooling systems to prevent thermal degradation, and improving control over sonication time and power. Uneven heating and size distribution are the challenges being overcome in microwave-assisted synthesis by optimizing microwave power levels and using multi-mode microwave reactors, which ensure uniform energy distribution. The electrospinning technique suffers from the problem of poor thickness and uniformity control, although this is improved with recent advances in co-spinning techniques, better electrospinning equipment, and improved environmental controls such as humidity and temperature. Problems arise in the bubble electrospinning technique in terms of size control of the bubbles and porosity. The use of surfactants in the process to stabilize the bubble formation helps, along with the optimization of electrospinning parameters for better control over the final structure. All of these methods indicate that the current research in process development is focused on the optimization of process control, reproducibility, and scalability that can meet the ever-increasing demand for high-quality NS in drug delivery, tissue engineering, and environmental applications (Banoet al., 2019).
FACTORS GOVERNING THE PREPARATION OF NANOSPONGE
Different polymers used
The various polymers employed impact the performance and formulation of the NS structure. To accommodate a drug molecule and to interact the drug with the NS. The particle dimension of NS must be in the right specific range.
Temperature
Variations in temperature have an effect on a complex’s stability constant. The apparent stability is decreased at high temperatures due of the diminished forces that hold the medication on to the NS.
Degree of substitution
Type drugs
The drug molecules should possess some properties to make a complex with NS. They include molecular weight, which must be between 100 and 400 nm and less than five condensed rings. The medication must melt at no more than 250°C and have a water solubility of not more than 10 mg/mL.
Drug-nanosponge complexation
The drug-NS complexation can be disrupted by introducing the active pharmaceutical molecule into the NS. The expert approach determines the kind of polymer and the pharmaceutical molecule. The freezing process is the most effective method for medication complexation (Pritesh Patel, 2014).
Nanosponge interaction
The drug molecules whose molecular weights lie between the range of 100-400 Da must successfully saturate so that they can interact with the NS in the nanocavities. The best possible chemical and physical qualities are a must for interaction.
Types of CD and crosslinkers
CHARACTERIZATION OF NANOSPONGES
Solubility studies
The phase solubility technique is a widely used method for studying inclusion complexation and drug solubility, using phase diagrams and an Erlenmeyer flask, purified, and determined using HPLC.
Zeta potential
Zeta potential measures surface charge, and can be used in particle size measurement devices. NS samples are diluted with KCl and placed in an electrophoretic cell with 15 V/cm electric field.
Polydispersity Index (PDI) and particle size
Dynamic light scattering measures particle size using a 90 Plus particle sizer and MAS OPTION program, calculating mean diameter and PDI, which indicate the variation in particle size distribution between monodisperse and polydisperse samples.
The particle size may be measured using the following techniques: Freeze fracture electron microscopy, TEM, SEM, and atomic force microscopy (Arvapalliet al., 2017).
Sl. No. | PDI | Type of dispersion |
---|---|---|
1. | <1 | Single disperse Standard |
2. | 0.1-0.25 | Nearly Single disperse |
3. | >0.1 | Mid-range multi dispersity |
4. | >0.5 | Very multi disperse |
Scanning Electron Microscopy (SEM)
SEM is used to analyse morphological structures of NS, characterizing detailed particle structures. Samples are vacuum-sealed and coated with palladium or gold using a scanning electron microscope sputter coater unit at 15 kV acceleration voltage (Subramanian et al., 2012).
Fourier Transform Infrared spectroscopy (FTIR)
The NS formulation was optimized using potassium bromide and NS in a 1:90 ratio, compressed under 15 tons of pressure, and recorded using FTIR spectra in the 4000-400 cm-1 wavelength region, observing changes in optimized primary peaks over time.
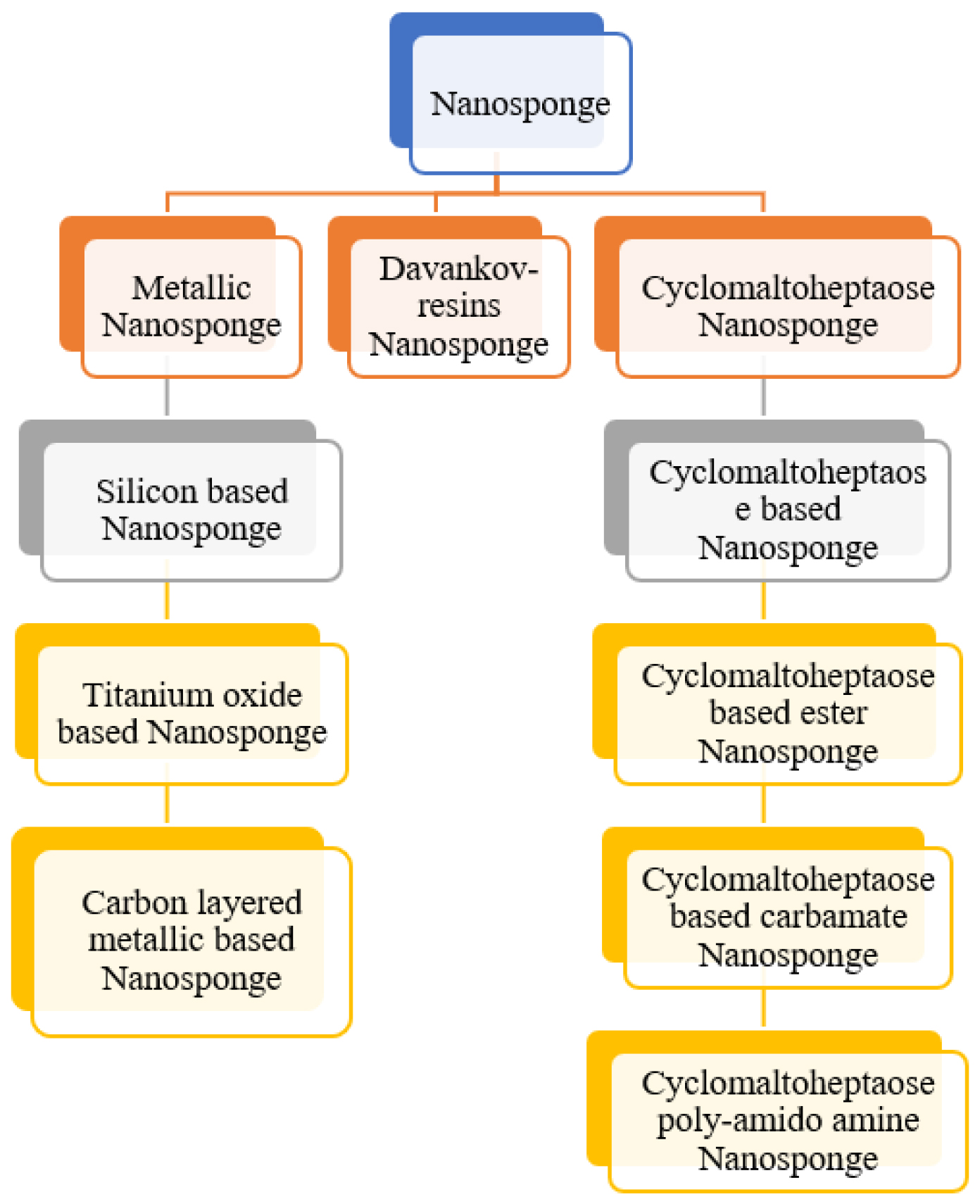
Figure 2:
Classification of NSs.
Differential Scanning Calorimetry (DSC)
DSC, the formulation batch of optimized NS was thermographed. Samples of nanosponges were stored in an aluminium pan, sealed, and heated between 40 and 400°C at a steady rate of 10°C per min. by using a 100 mL/min flow rate to purge nitrogen.
X-ray Diffractometry structure analysis (X-RD)
XRD is crucial for identifying solid-state inclusion complexes in drug molecules, with changes in diffraction patterns indicating new structures. Powder X-ray Diffraction (PXRD) patterns show solid phase formation, and complexes may produce additional peaks (M. R. P. Rao and Shirsath, 2017).
Thin layer chromatography
Thin-layer chromatography was used to determine the Rf values in these NS complex pharmaceutical molecules. The lower Rf value confirmed the presence of a complex between the NS and the drug molecule.
Raman spectroscopy
Raman spectroscopy, a molecular method using laser light, is used to identify chemical fingerprints, study molecular bond modifications, and identify vibrational modes. It offers advantages over FTIR spectroscopy, including minimal sample preparation and insensitivity to water absorption bands (Tejashriet al., 2013).
Resilience tests
NS’s toughness can be adjusted to create softer or tougher beadlets, and as crosslinking increases, the release rate decreases, allowing for improved resilience of sponges by considering the release sequence as a result of cross-linking (Singh et al., 2016).
Sl. No. | Drug | Uses | Brand Name | Manufacturer |
---|---|---|---|---|
1 | Tamoxifen | Breast cancer | Nolvadex, Soltamox. | AstraZeneca, Xanodyne Pharmaceuticals. |
2 | Iodine | Anti-septic | Iodex, Iodoflex, Iodosorb. | Smith and Nephew. |
3 | Alprostadil | Erectile dysfunction | Caverject, Edex, Prostin VR. | Pfizer, Endo Pharmaceuticals, Upjohn. |
4 | Dexamethasone | Used to treat inflammation | Neofordex, Glensoludex, Martapan. | Aspen, Hikma Pharmaceuticals. |
Sl. No. | Drug | Activity | Authors |
---|---|---|---|
1 | Fluconazole | Enhanced transdermal delivery | (Abbaset al., 2019) |
2 | Clobetasol propionate | Treatment of psoriasis | (Kumaret al., 2021) |
3 | Lemon grass oil | Antifungal | (Aldawsariet al., 2015) |
4 | Cinnamon oil | Antibacterial activity | (Kauret al., 2021) |
5 | Terbinafine Hydrochloride | Anti-fungal | (Ghoseet al., 2020) |
6 | Mupirocin | Diabetic foot ulcers | (Dhamaket al., 2024) |
7 | Boswellia carterii oil | Anti-inflammatory activity | (Talebet al., 2024) |
8 | Kynurenic acid | Antioxidant activity | (Dhakaret al., 2019) |
9 | Ferulic acid | Anticancer activity | (Rezaeiet al., 2019) |
10 | Limonene | Antibacterial | (Salehiet al., 2021) |
11 | Paclitaxel | Anticancer activity | (Mognettiet al., 2012) |
12 | Babchi Oil | Cytotoxicity | (Kumaret al., 2018) |
13 | Vaccines | Activity | Authors |
14 | mRNA | Protection mRNA | (Abou Talebet al., 2022) |
15 | Influenza | Sustained Release | (Lemboet al., 2018) |
16 | Tumor-associated antigens (TAA) | Cancer immunotherapy | (Swaminathanet al., 2016) |
17 | Enzymes | Activity | Authors |
18 | Glutathione Peroxidase | Detoxification | (Dagaet al., 2020) |
19 | Lipases | Hydrolytic | (Boscoloet al., 2010) |
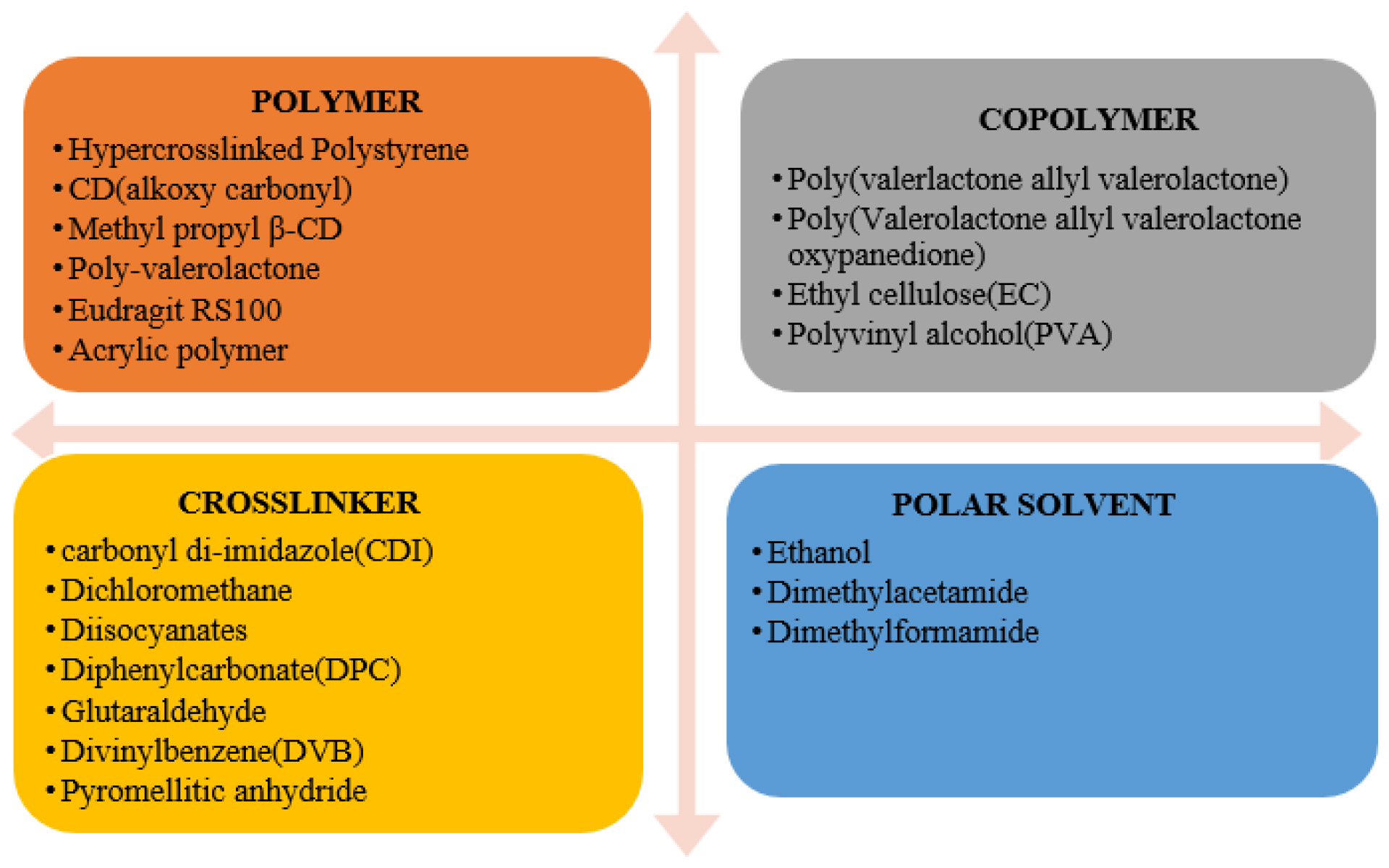
Figure 3:
Materials used in preparation of NSs.
Study Type | Drugs Used | Application | Outcomes |
---|---|---|---|
In vitro | Doxorubicin (anticancer drug) | Cancer drug delivery and controlled release. | Increased drug stability, controlled release, and reduced toxicity (Argenzianoet al., 2020). |
Ibuprofen (NSAID) | Controlled-release drug delivery. | Prolonged drug release and enhanced solubility | |
Antimicrobial peptides (AMPs) | Antimicrobial coatings for medical devices. | Enhanced antimicrobial activity and reduced bacterial resistance. | |
Methotrexate (chemotherapeutic agent) | Rheumatic arthritis | Sustained drug release, increased therapeutic efficacy (Banjareet al., 2020). | |
Resveratrol (antioxidant) | Drug delivery for neurodegenerative diseases. | Enhanced solubility and stability of resveratrol. | |
In vivo | Paclitaxel (anticancer drug) | Cancer therapy | Improved drug bioavailability, reduced systemic toxicity, and targeted delivery (Clementeet al., 2019). |
Quercetin (antioxidant) | Neuroprotective therapy. | Enhanced brain bioavailability and neuroprotective effects. | |
Econazole (antifungal drug) | Antifungal drug delivery. | Higher antifungal activity, prolonged action, and reduced dosage frequency (Srivastavaet al., 2021). | |
Rifampicin (anti-tuberculosis drug) | Anti-tuberculosis drug delivery. | Enhanced permeability, controlled release, and reduced toxicity. | |
Indomethacin (NSAID) | Anti-inflammatory drug delivery. | Reduced gastrointestinal side effects and sustained release. | |
Vaccines (antigen delivery) | Controlled vaccine delivery. | Sustained antigen release and enhanced immune response. | |
Clinical trials | Paclitaxel | Cancer therapy | Ongoing clinical evaluation for improved efficacy and reduced side effects. |
Doxorubicin | Cancer therapy | Clinical studies focusing on sustained release and reduced cardiotoxicity. | |
Insulin | Diabetes treatment | Evaluation of oral insulin delivery for improved absorption and bioavailability. | |
Curcumin | Cancer, anti-inflammatory treatments. | Early-stage trials for enhancing curcumin bioavailability and therapeutic potential. | |
Fluconazole | Antifungal drug delivery. | Clinical studies to improve fluconazole release and therapeutic efficacy. |
Efficiency loading and entrapment
A measured number of packed NS groups needs to be dispersed in a suitable solvent, sonicated, and then adequately diluted before being analyzed using a UV spectrophotometer or HPLC. The loading efficiency of NS may be calculated using the formula below.
AD = Actual drug,
FE = Filling Efficiency,
CDC = Calculated drug content.
Reaction Yield (RY)
The initial and finish weights of the basic materials may be used to calculate the RY.
PM = Practical mass,
RY = Reaction yield,
TY = Theoretical yield (polymer+drug).
Actual drug content
A 10 mg dose of drug-containing NS was mixed with 100 mL of phosphate buffer pH 7.4 solution and swirled for an hour. Filtered samples were analysed using a UV-visible spectrophotometer at 232 nm, beside a blank.
Where Nacm=actual content in a measured amount of NS Nma=Measured amount of NS
APPLICATIONS OF NANOSPONGE
Sustained drug delivery
Pharmaceuticals and Medical Devices Agency (PMDA) created insulin NS, sensitivity to pH, and efficacy in glipizide release after 12 hr, acyclovir for sustained release due to slow absorption in the gastrointestinal system.
NS as a carrier for gas delivery
In order to serve as gas carriers for gases like carbon dioxide and oxygen. The CD-based NS with improved penetration profiles. This allowed for a slower and more prolonged release of oxygen for topical administration.
Protein delivery
Solubility and bioavailability enhancer
Nanosponge in cancer therapy
Tumours can be treated using anticancer drugs delivered by NS, which focuses on peptides attaching to radiation-activated cell surface receptors, potentially enhancing the solubility of camptothecin, thereby circumventing its limitations (Iravani and Varma, 2022).
Stability enhancer
NS enhances soluble, stable, and efficient dissolution of compounds like volatile oils, medications, Bovine Serum Albumin (BSA) proteins, and resveratrol, while genetic engineering improves enzyme stability, economy, and specificity.
Drug delivery
There are several ways to employ NP, including topical, parenteral, aerosol, tablet, and capsule forms. They are useful nanocarriers for the treatment of cancer because they increase the solubility of medications including TEL, paclitaxel, and econazole nitrate (Ahmed, 2012).
Enzyme entrapment
Severe Acute Respiratory Syndrome (SARS)-CoV-2 inhibition
Diagnostic test
β-CD is extensively employed in the formulation of multiple diagnostic products. CD-NS are favored for their excellent biocompatibility, prolonged circulation time in the blood, consistent particle size distribution that enhances permeability, and their efficient ability to reach specific targets (Gidwani and Vyas, 2015).
Cosmetics
NSs are used in the cosmetics industry to protect photosensitive components by prolonging the release of volatile oils and absorbing unpleasant bodily odours. They also subtly eliminate volatile substances, leaving oral cosmetics fresh for longer, and providing a long-lasting appearance in rouge and lipsticks (Bilensoy, 2011).
DRUG RELEASE MECHANISM AND PHARMACOKINETIC STUDIES
Nanosponges release drugs in a controlled release mechanism, where the medication migrates towards the skin’s stratum corneum until fully absorbed, resulting in a pseudo-zero order pattern of drug release (Sujitha and Muzib, 2019).
RELEASE PROFILE
A rotating multicompartment cell with a dialysis membrane is used to test NS in vitro release. The donor phase contains drug-loaded NS complexes, while the receptor phase is removed and diluted. The USP Apparatus II can be used in various applications. The release data helps understand drug release mechanisms. Firstand zero-order models are fitted, and data analysis software like GraphPad Prism is used to find optimal nonlinear functions and parameters (Pyraket al., 2024). Table 2 describes various drugs that are available in the market as nanosponge formulations.
Emerging Trends and Future Research Areas in NS Technology
NSs are being developed as a promising drug delivery platform for cancer therapy, infectious disease control, and environmental remediation. They can be used in stimuli-responsive drug delivery systems that respond to environmental triggers such as pH, temperature, or light to release drugs. They allow for site-specific delivery of drugs, which avoids side effects and improves the treatment outcome. Studies have also been done using NSs with dual-mode therapy, where a combination of chemotherapy with photothermal or photodynamic therapy degrades the tumor effectively. They are also of interest for oral delivery of biologics, where there is a problem of degradation within the gastrointestinal tract (Mohiteet al., 2024) Table 3 shows the list of recent studies on nanosponges.
Another rapidly expanding area includes the control of infectious diseases with the study of biomimetic NSs, mimicking human cell membranes, that neutralize pathogens. Clinics already use antimicrobial-loaded NSs in wound dressings and medical devices to prevent infection. NS formulations for anti-viral drugs of COVID-19 and medications used in wound healing are showing productive results in clinical trials. Table 4 gives a list of nanosponge-based formulations in in vitro, in vivo, and clinical trial studies.
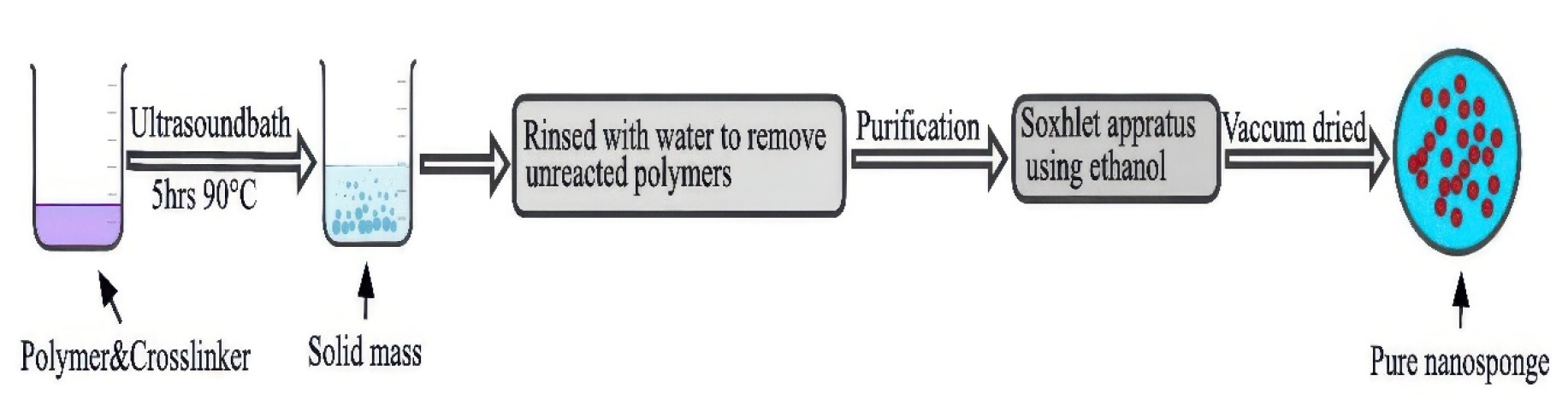
Figure 4:
Ultrasound assisted synthesis of NSs.
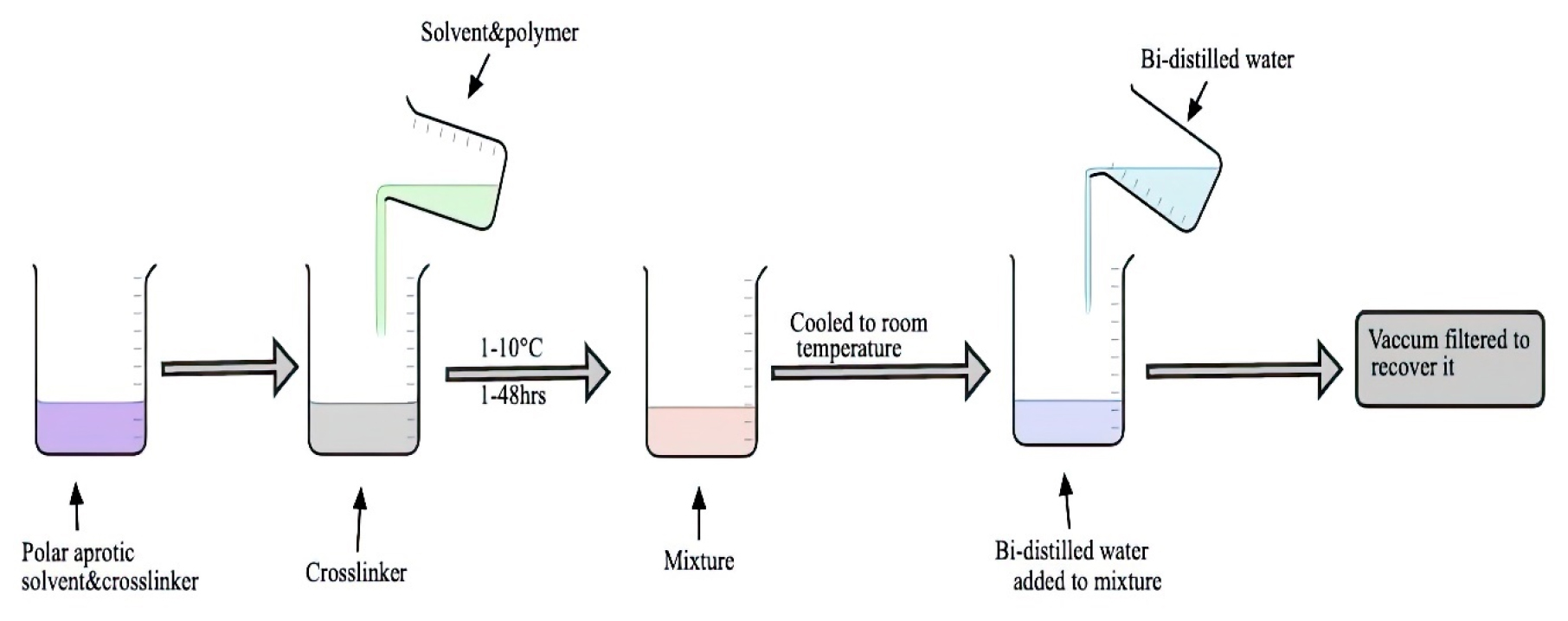
Figure 5:
Solvent method of preparation of NSs.
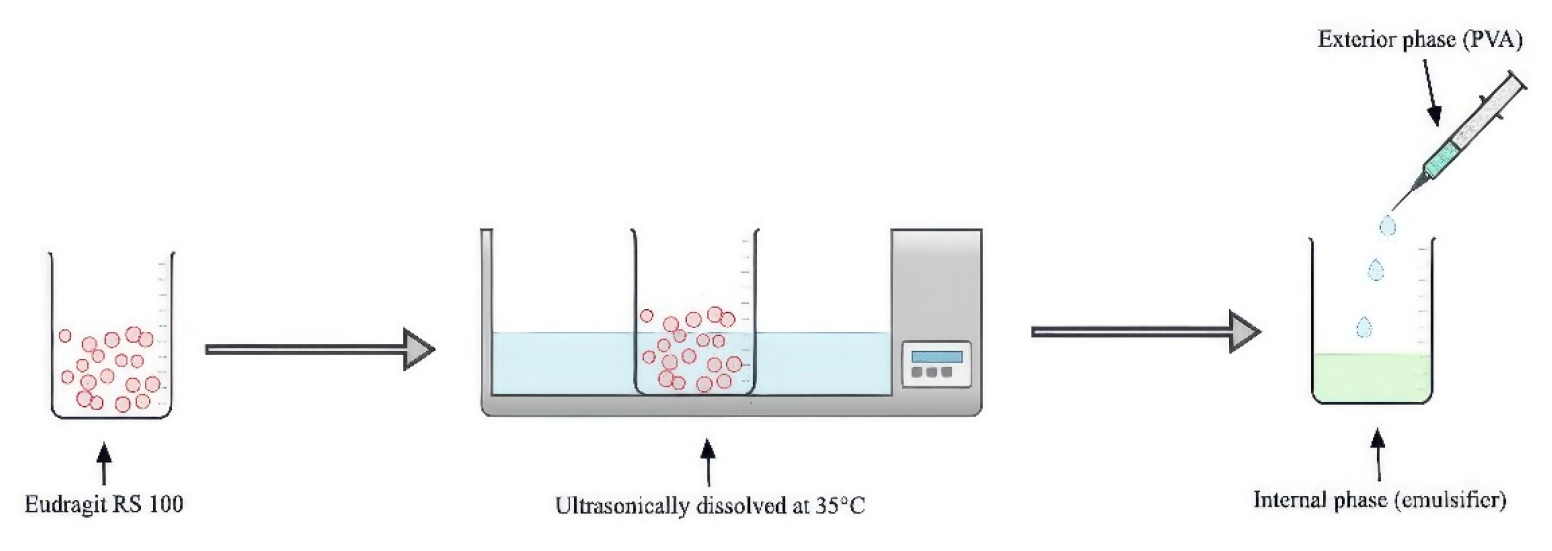
Figure 6:
Co-solvent evaporation method of preparation of NSs.
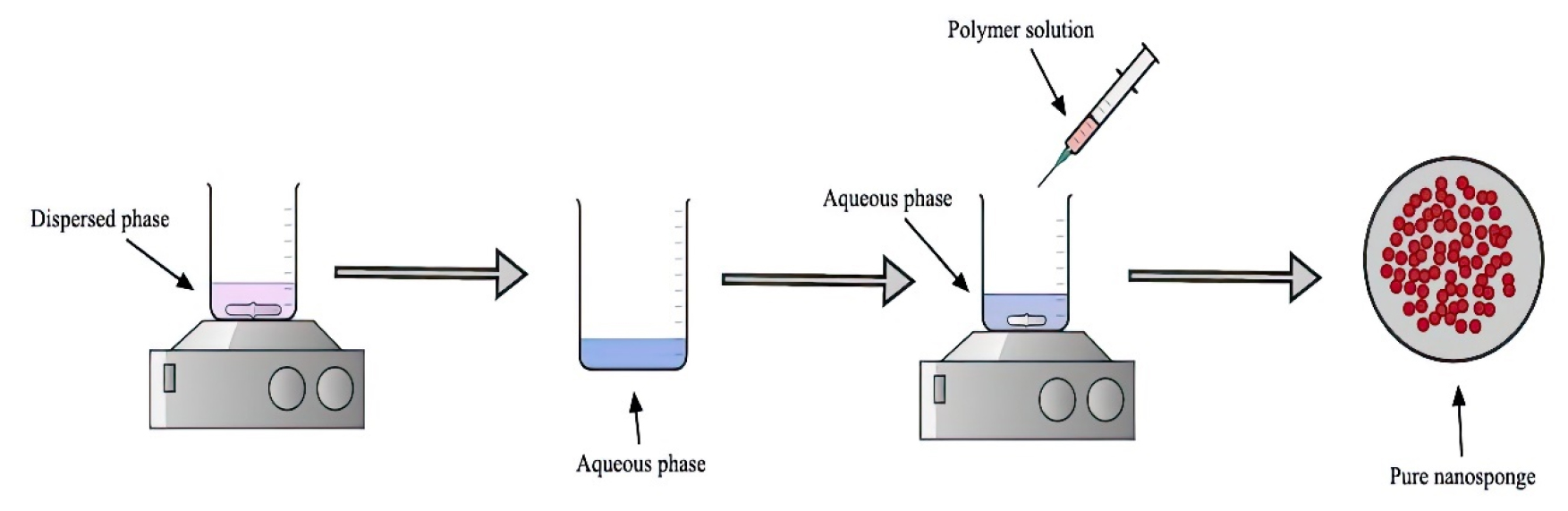
Figure 7:
Solvent displacement method of NS preparation.
Environmental applications of NSs are also being explored regarding water purification and pollution control. NSs can adsorb heavy metals, dyes, organic solvents, and others from water, thus being an effective, sustainable, and cost-effective method of environmental remediation. Future focus will be placed on overcoming scalability, toxicity, and biocompatibility while improving large-scale production methods and incorporating nanorobotic systems for targeted drug delivery (Tannouset al., 2020).
CONCLUSION
NS is a versatile class of nanomaterials with a large potential for use in different fields. Their distinctive features, such as high superficial expanse, adjustable aperture size, and functionality, make them promising candidates for numerous applications.
NS can deliver both hydrophilic and lipophilic drugs via several routes, including oral, transdermal, rectal, and parenteral routes. In particular, β-NS Improves the solubility of drugs classified as BCS Class II. which is useful for certain drug delivery techniques. The formulation design, applications, characterization techniques, synthesis methods, and modifications of NS are discussed in this extensive review. Human health has already improved owing to recent approvals of drug delivery systems based on NS technology. At the same time, ongoing research continues to explore new pharmaceuticals that will help improve therapeutic outcomes. The safety and effectiveness of such medications delivered through NS as an innovation are expected to encourage pharmaceutical companies to adopt them.
Cite this article:
Dhanush R, Sowmya C, Sujatha K. Nanosponges: An Emerging and Promising Strategy in Drug Delivery. J Young Pharm. 2025;17(2):279-91.
REFERENCES
REFERENCES
- Abbas, N., Parveen, K., Hussain, A., Latif, S., Uz Zaman, S. U., Shah, P. A., & Ahsan, M. (2019). Nanosponge-based hydrogel preparation of fluconazole for improved topical delivery. Tropical Journal of Pharmaceutical Research, 18(2), 215-222. https://doi.org/10.4314/tjpr.v18i2.1
- Abou Taleb, S., Moatasim, Y., GabAllah, M., & Asfour, M. H. (2022). Quercitrin loaded cyclodextrin based nanosponge as a promising approach for management of lung cancer and COVID-19. Journal of Drug Delivery Science and Technology, 77(July), Article 103921. https://doi.org/10.1016/j.jddst.2022.103921
- Agrawal, R. V., Gangurde, R. B., & Jadhav, K. R. (2020). Nanosponges: An overview on processing, application and evaluation, Agrawal, et al. World Journal of Pharmaceutical Research, 9. https://doi.org/10.20959/wjpr202012-18768
- Ahmed, R. Z. (2012). Nanosponges – A completely new nano-horizon: Pharmaceutical applications and recent advances.
- Aldawsari, H. M., Badr-Eldin, S. M., Labib, G. S., & El-Kamel, A. H. (2015). Design and formulation of a topical hydrogel integrating lemongrass-loaded nanosponges with an enhanced antifungal effect: In vitro/in vivo evaluation. International Journal of Nanomedicine, 10, 893-902. https://doi.org/10.2147/IJN.S74771
- Annammadevi, G. S., & Anusha, A. (2022). Study about nanosponges- an emerging technology. In D. S. A. A. Rizvi. (Ed.), 2 (pp. 32-57). Book Publisher International. https://doi.org/10.9734/bpi/capr/v2/3638E
- Argenziano, M., Gigliotti, C. L., Clemente, N., Boggio, E., Ferrara, B., Trotta, F., Pizzimenti, S., Barrera, G., Boldorini, R., Bessone, F., Dianzani, U., Cavalli, R., & Dianzani, C. (2020). Improvement in the antitumor efficacy of doxorubicin nanosponges in vitro and in mice bearing breast tumor models. Cancers, 12(1), 1-19. https://doi.org/10.3390/cancers12010162
- Arvapalli, S., Arvapally, S., Harini, M., & Harshitha, G. (2017). Formulation and in vitro evaluation of glipizide nanosponges. American Journal of PharmacyTech Res, 7(3). http://www.ajptr.com/www.ajptr.com.
- Banjare, N., Gautam, L., Behera, C., Gupta, P. N., Vyas, S., & Vyas, S. P. (2020). Cyclodextrin nanosponges based site-retentive controlled release system for treatment of rheumatic arthritis. Journal of Drug Delivery Science and Technology, 60(June), Article 101973. https://doi.org/10.1016/j.jddst.2020.101973
- Bano, N., Ray, S. K., Shukla, T., Upmanyu, N., Khare, R., Pandey, S. P., & Jain, P. (2019). Multifunctional nanosponges for the treatment of various diseases: A review. Asian Journal of Pharmacy and Pharmacology, 5(2), 235-248. https://doi.org/10.31024/ajpp.2019.5.2.4
- Bhowmik, H., Venkatesh, D. N., Kuila, A., & Kumar, K. H. (2018). Nanosponges: A review. In International Journal of Applied Pharmaceutics (Vol. 10, Issue 4, pp. 1-5). Innovare Academics Sciences Pvt. Ltd., 10(4). https://doi.org/10.22159/ijap.2018v10i4.25026
- Bilensoy, E. (2011). Cyclodextrins in pharmaceutics, cosmetics, and biomedicine: Current and future industrial applications. In Cyclodextrins in pharmaceutics, cosmetics, and biomedicine: Current and future industrial applications. https://doi.org/10.1002/9780470926819
- Biswas, G. R., Majee, S. B., & Roy, A. (2016). Combination of synthetic and natural polymers in hydrogel: An impact on drug permeation. Journal of Applied Pharmaceutical Science, 6(11), 158-164. https://doi.org/10.7324/JAPS.2016.601125
- Boscolo, B., Trotta, F., & Ghibaudi, E. (2010). High catalytic performances of Pseudomonas fluorescens lipase adsorbed on a new type of cyclodextrin-based nanosponges. Journal of Molecular Catalysis B: Enzymatic, 62(2), 155-161. https://doi.org/10.1016/j.molcatb.2009.10.002
- Burad, S., Markad, K., Kulkarni, N., & Dhole, S. (2023). Assessment and outcome on preparations, characterization of topical targeted nanosponge based drug delivery. Critical Review, 16. https://doi.org/10.22159/ajpcr.2023v16i5.46809
- Challa, R., Ahuja, A., Ali, J., & Khar, R. K. (2005). Cyclodextrins in drug delivery: An updated review. In AAPS PharmSciTech. AAPS PharmSci Editorial Office, 6(2), E329-E357. https://doi.org/10.1208/pt060243
- Chilajwar, S. V., Pednekar, P. P., Jadhav, K. R., Gupta, G. J. C., & Kadam, V. J. (2014). Cyclodextrin-based nanosponges: A propitious platform for enhancing drug delivery. In Expert Opinion on Drug Delivery. Informa Healthcare, 11(1), 111-120. https://doi.org/10.1517/17425247.2014.865013
- Clemente, N., Argenziano, M., Gigliotti, C. L., Ferrara, B., Boggio, E., Chiocchetti, A., Caldera, F., Trotta, F., Benetti, E., Annaratone, L., Ribero, S., Pizzimenti, S., Barrera, G., Dianzani, U., Cavalli, R., & Dianzani, C. (2019). Paclitaxel-loaded nanosponges inhibit growth and angiogenesis in melanoma cell models. Frontiers in Pharmacology, 10(July), 776. https://doi.org/10.3389/fphar.2019.00776
- Daga, M., de Graaf, I. A. M., Argenziano, M., Barranco, A. S. M., Loeck, M., Al-Adwi, Y., Cucci, M. A., Caldera, F., Trotta, F., Barrera, G., Casini, A., Cavalli, R., & Pizzimenti, S. (2020). Glutathione-responsive cyclodextrin-nanosponges as drug delivery systems for doxorubicin: Evaluation of toxicity and transport mechanisms in the liver. Toxicology in Vitro, 65, Article 104800. https://doi.org/10.1016/j.tiv.2020.104800
- Dhakar, N. K., Caldera, F., Bessone, F., Cecone, C., Pedrazzo, A. R., Cavalli, R., Dianzani, C., & Trotta, F. (2019). Evaluation of solubility enhancement, antioxidant activity, and cytotoxicity studies of kynurenic acid loaded cyclodextrin nanosponge. Carbohydrate Polymers, 224, Article 115168. https://doi.org/10.1016/j.carbpol.2019.115168
- Dhamak, R. S., Salve, P. S., Tiwari, D. R., Raut, P. A., & Waghmare, D. S. (2024). Enhanced therapeutic approach for diabetic foot ulcers: Comparative study and characterisation of mupirocin-loaded nanosponge gel with marketed formulation for accelerating wound healing and in vivo evaluation. Future Journal of Pharmaceutical Sciences, 10(1). https://doi.org/10.1186/s43094-024-00604-6
- Gardouh, A. R., Elhusseiny, S., & Gad, S. (2022). Records of pharmaceutical and biomedical sciences Polymeric cyclodextrin nanosponge drug delivery systems: A review. In Biomedical Science, 6(3).
- Ghose, A., Nabi, B., Rehman, S. MD, S., Alhakamy, N. A., Ahmad, O. A. A., Baboota, S., & Ali, J. (2020). Development and evaluation of polymeric nanosponge hydrogel for terbinafine hydrochloride: Statistical optimization, in vitro and in vivo studies. Polymers, 12(12), 1-19. https://doi.org/10.3390/polym12122903
- Ghurghure, S. M., Sana, M., Pathan, A., & Surwase, R. (n.d.). International Journal of Chemistry Studies Nanosponges: A Novel Approach for Targeted Drug Delivery System. http://www.chemistryjournal.in
- Gidwani, B., & Vyas, A. (2015). A comprehensive review on cyclodextrin-based carriers for delivery of chemotherapeutic cytotoxic anticancer drugs. In BioMed Research International. Hindawi Publishing, 2015, Article 198268. https://doi.org/10.1155/2015/198268
- Iravani, S., & Varma, R. S. (2022). Nanosponges for drug delivery and cancer therapy: Recent advances. In Nanomaterials. MDPI, 12(14), Article 2440. https://doi.org/10.3390/nano12142440
- Kaur, M., Nagpal, M., Singh, M., Singh, T. G., Aggarwal, G., & Dhingra, G. A. (2021). Improved antibacterial activity of topical gel-based on nanosponge carrier of cinnamon oil. BioImpacts, 11(1), 23-31. https://doi.org/10.34172/BI.2021.04
- Kaur, S., & Kumar, S. (2019). The nanosponges: An innovative drug delivery system. Asian Journal of Pharmaceutical and Clinical Research, 60-67. https://doi.org/10.22159/ajpcr.2019.v12i7.33879
- Kerilos, I. E., El-Sawy, H. S., Abu Elyazid, S. K., & Ibrahim, M. (2024). Nanosponge for enhancing solubility and bioavailability of oral drugs [Review]. In International Journal of Applied Pharmaceutics. Innovare Academics Sciences Pvt. Ltd., 9-17. https://doi.org/10.22159/ijap.2024v16i1.49490
- Kumar, S., Pooja, Trotta, F., & Rao, R. (2018). Encapsulation of babchi oil in cyclodextrin-based nanosponges: Physicochemical characterization, photodegradation, and in vitro cytotoxicity studies. Pharmaceutics, 10(4), Article 169. https://doi.org/10.3390/pharmaceutics10040169
- Kumar, S., Prasad, M., & Rao, R. (2021). Topical delivery of clobetasol propionate loaded nanosponge hydrogel for effective treatment of psoriasis: Formulation, physicochemical characterization, antipsoriatic potential and biochemical estimation. Materials Science and Engineering. C, Materials for Biological Applications, 119, Article 111605. https://doi.org/10.1016/j.msec.2020.111605
- Lembo, D., Trotta, F., & Cavalli, R. (2018). Cyclodextrin-based nanosponges as vehicles for antiviral drugs: Challenges and perspectives. Nanomedicine, 13(5), 477-480. https://doi.org/10.2217/nnm-2017-0383
- Mahalekshmi, V., Balakrishnan, N., & Parthasarathy, V. (2023). Recent advancement of nanosponges in pharmaceutical formulation for drug delivery systems. Journal of Applied Pharmaceutical Science, 13(8), 84-100. https://doi.org/10.7324/JAPS.2023.123441
- Mognetti, B., Barberis, A., Marino, S., Berta, G., De Francia, S., Trotta, F., & Cavalli, R. (2012). In vitro enhancement of anticancer activity of paclitaxel by a cremophor free cyclodextrin-based nanosponge formulation. Journal of Inclusion Phenomena and Macrocyclic Chemistry, 74(1-4), 201-210. https://doi.org/10.1007/s10847-011-0101-9
- Mohite, P., Munde, S., Pawar, A., & Singh, S. (2024). Unleashing the potential of cyclodextrin-based nanosponges in management of colon cancer: A review. Nanofabrication, 9, 1-20. https://doi.org/10.37819/nanofab.9.1823
- Pandya, B., Dabhi, F. A., V. D. Shah, & Pandya, B. D. (2023). The nanosponges: An innovative drug delivery system. In Certified [Journal] │ Dabhi, et al. European Journal of Pharmaceutical and Medical Research, 10. https://www.researchgate.net/publication/370843636.
- Poonam, R., Prakash, J., Priyanka, S., Vivekkumar, R., Nikita, B., & Varda, J. (2023). A comprehensive review on transdermal delivery of nanosponges. Asian Journal of Pharmaceutical Research and Development, 11(4), 126-128. https://doi.org/10.22270/ajprd.v11i4.1298
- Pritesh Patel, A. D. (2014). review on cyclodextrin based nanosponges prepared by different methods: physicochemical characterization, factors influencing formation and applications.
- Pyrak, B., Rogacka-Pyrak, K., Gubica, T., & Szeleszczuk, Ł. (2024). Exploring cyclodextrin-based nanosponges as drug delivery systems: Understanding the physicochemical factors influencing drug loading and release kinetics. International Journal of Molecular Sciences, 25(6), Article 3527. https://doi.org/10.3390/ijms25063527
- Rao, M., Bajaj, A., Khole, I., Munjapara, G., & Trotta, F. (2013). In vitro and in vivo evaluation of β-cyclodextrin-based nanosponges of telmisartan. Journal of Inclusion Phenomena and Macrocyclic Chemistry, 77(1-4), 135-145. https://doi.org/10.1007/s10847-012-0224-7
- Rao, M. R. P., & Shirsath, C. (2017). Enhancement of bioavailability of non-nucleoside reverse Transciptase inhibitor using nanosponges. AAPS PharmSciTech, 18(5), 1728-1738. https://doi.org/10.1208/s12249-016-0636-6
- Rezaei, A., Varshosaz, J., Fesharaki, M., Farhang, A., & Jafari, S. M. (2019). Improving the solubility and in vitro cytotoxicity (Anticancer activity) of ferulic acid by loading it into cyclodextrin nanosponges. International Journal of Nanomedicine, 14, 4589-4599. https://doi.org/10.2147/IJN.S206350
- S, S., S, A., Krishnamoorthy, K., & Rajappan, M. (2012). Nanosponges: A novel class of drug delivery system – Review. Journal of Pharmacy and Pharmaceutical Sciences, 15(1), 103-111. https://doi.org/10.18433/j3k308
- Salehi, O., Sami, M., & Rezaei, A. (2021). Limonene loaded cyclodextrin nanosponge: Preparation, characterization, antibacterial activity and controlled release. Food Bioscience, 42. https://doi.org/10.1016/j.fbio.2021.101193
- Sherje, A. P., Dravyakar, B. R., Kadam, D., & Jadhav, M. (2017). Cyclodextrin-based nanosponges: A critical review. Carbohydrate Polymers, 173(1), 37-49. https://doi.org/10.1016/j.carbpol.2017.05.086
- Shinde, S. M., More, P. U., Borkar, S. P., Yadav, V. D., Zambare, S. D., & Sontakke, S. B. (2024). New trends in emerging novel nanosponges drug delivery. Asian Journal of Pharmaceutical Research and Development, 12(2), 52-63. https://doi.org/10.22270/ajprd.v12i2.1360
- Shivani, S., & Kumar Poladi, K. (2015). Nanosponges-novel emerging drug delivery system: A review. International Journal of Pharmaceutical Sciences and Research, 6(2), 529. https://doi.org/10.13040/IJPSR.0975-8232.6(2).529-40
- Shringirishi, M., Prajapati, S. K., Mahor, A., Alok, S., Yadav, P., & Verma, A. (2014). Nanosponges: A potential nanocarrier for novel drug delivery-a review. Asian Pacific Journal of Tropical Disease, 4(Suppl. 2), S519-S526. https://doi.org/10.1016/S2222-1808(14)60667-8
- Singh, D., Soni, G. C., & Prajapati, S. K. (n.d.). Recent advances in nanosponges as drug delivery system: A review article. In European Journal of Pharmaceutical and Medical Research, 364. http://www.ejpmr.com.
- Srivastava, S., Mahor, A., Singh, G., Bansal, K., Singh, P. P., Gupta, R., Dutt, R., Alanazi, A. M., Khan, A. A., & Kesharwani, P. (2021). Formulation development, In Vitro and In Vivo Evaluation of Topical Hydrogel Formulation of Econazole Nitrate-Loaded β-Cyclodextrin Nanosponges. Journal of Pharmaceutical Sciences, 110(11), 3702-3714. https://doi.org/10.1016/j.xphs.2021.07.008
- Sujitha, Y. S., & Muzib, Y. I. (2019). Formulation and optimization of quercetin loaded nanosponges topical gel: Ex vivo, pharmacodynamic and pharmacokinetic studies. International Journal of Applied Pharmaceutics, 11(5), 156-165. https://doi.org/10.22159/ijap.2019v11i5.32850
- Sultana, N. (2024). Nanosponges- An emerging trend in drug delivery. Biological Sciences, 4(1). https://doi.org/10.55006/biolsciences.2024.4104
- Swaminathan, S., Cavalli, R., & Trotta, F. (2016). Cyclodextrin-based nanosponges: A versatile platform for cancer nanotherapeutics development. Wiley Interdisciplinary Reviews. Nanomedicine and Nanobiotechnology, 8(4), 579-601. https://doi.org/10.1002/wnan.1384
- Taleb, S. A., Ibrahim, B. M. M., Mohammed, M. A., Yassen, N. N., Hessin, A. F., Gad, S. A., & Darwish, A. B. (2024). Development and in vitro/in vivo evaluation of a nanosponge formulation loaded with Boswellia carterii oil extracts for the enhanced anti-inflammatory activity for the management of respiratory allergies. Journal of Pharmaceutical Investigation, 54(5), 643-665. https://doi.org/10.1007/s40005-024-00676-9
- Tannous, M., Trotta, F., & Cavalli, R. (2020). Nanosponges for combination drug therapy: State-of-the-art and future directions. Nanomedicine, 15(7), 643-646. https://doi.org/10.2217/nnm-2020-0007
- Tejashri, G., Amrita, B., & Darshana, J. (2013). Cyclodextrin based nanosponges for pharmaceutical use: A review. In Acta Pharmaceutica, 63(3), 335-358. https://doi.org/10.2478/acph-2013-0021
- Thakre, A. R., Gholse, Y. N., & Kasliwal, R. H. (2016). Nanosponges: A novel approach of drug delivery system. Journal of Medical Pharmaceutical and Allied Sciences. http://www.jmpas.com.
- Tiwari, K., & Bhattacharya, S. (2022). The ascension of nanosponges as a drug delivery carrier: Preparation, characterization, and applications. Journal of Materials Science. Materials in Medicine, 33(3), 28. https://doi.org/10.1007/s10856-022-06652-9
- Trotta, F., Zanetti, M., & Cavalli, R. (2012). Cyclodextrin-based nanosponges as drug carriers. In Beilstein Journal of Organic Chemistry, 8, 2091-2099. https://doi.org/10.3762/bjoc.8.235
- Yadav, G., Geeta, Y., & Hiten, P. (2014). Nanosponges-A Boon to the Targeted drug Delivery Syetem nanosponges: A boon to the targeted drug delivery system. Journal of Drug Delivery and Therapeutics, 2013(3), 151-155. http://jddtonline.info