ABSTRACT
Novasomes, a new drug carrier developed by Novavax, offers enhanced absorption and reduced side effects compared to traditional forms. These multilayered vesicles, with a diameter of 200-700 nm, maintain stability across pH and temperature ranges. They can be positively, negatively, or neutrally charged, enhancing drug encapsulation efficiency. Novasomes are versatile pharmaceutical tools used for treating ophthalmic conditions and fungal infections, improving drug bioavailability, stability, and penetration. Their ability to encapsulate a variety of drugs makes them suitable for a wide range of therapeutic applications, showing promising results in enhancing drug efficacy and improving patient outcomes. Their unique structural properties enable controlled drug release, and their excellent safety profile, demonstrated in numerous studies, supports their potential use in pharmaceutical technology, providing reassurance to the audience. Novasome’s disadvantages include the requirement for specialized equipment for production and the possibility of skin irritation. Nonetheless, they are a good choice for several medicinal applications due to their advantages in improving drug delivery. Characterization techniques include FTIR, permeation studies, DSC, zeta potential measurement, drug release, vivo studies, and stability studies. Innovative drug delivery systems, such as Novasomes, have shown promising results in improving drug efficacy and patient outcomes. They reduce systemic side effects by targeting the affected area and lowering drug dosages, minimizing potential adverse reactions. Future studies can concentrate on examining how novasome technology might be used to deliver a greater variety of drugs and vaccines, as well as creating more effective and efficient processes for developing novasome vesicles.
INTRODUCTION
Advances in pharmaceutical technology have led to innovative drug delivery methods, such as Novasome microvesicles, which are biocompatible phospholipids and single-tailed amphiphiles. These vesicles have a diameter of 200-700 nanometers, two seven-bilayered membranes, and are stable in a pH range of 2 to 13 and above the boiling point of water.1
Novasome molecules are hydrophobic tail and hydrophilic head groups, composed of glycerolipids, long-chain amino acids, and fatty alcohol derivatives. They are made from polyoxyethylene fatty acid monoesters, free fatty acids, and cholesterol. Encapsulation efficiencies range from 78-88% for aqueous materials and 99-100% for lipid materials.2
Novasome is a liposomal system that combines water-soluble and insoluble drugs for targeted release, improving therapeutic efficacy.3 Its charge-neutral bilayers minimize body incompatibility and are effective across pH levels. Recent advancements focus on improving stability and optimizing novasomal vesicles for transdermal drug delivery.4
Novasomes are being researched for improved drug delivery to body cells, enabling more controlled and accurate release of therapeutic substances. Their unique structure improves drug encapsulation efficiency. Unlike conventional drugs, novasomes can overcome low absorption rates and systemic side effects. Future research aims to optimize delivery efficiency and explore new medical applications. Collaborations with pharmaceutical companies could accelerate the commercialization of novasome technology for widespread healthcare use.5
MATERIALS AND METHODS
The review was conducted over four months, from January 19, 2024, to May 11, 2024, following approval from the Research Ethics Committee at the University of Biological and Applied Sciences (UBAS) in Lahore, Pakistan (reference number RMEC/AM/09778). This systematic review primarily used PubMed and Cochrane databases, adhering to PRISMA flow statement guidelines to ensure rigor. Relevant studies were identified using specific keywords, including “Novasomes,””Novasomes Composition and Structure,””Synthesis of Novasomes,””Characterization of Novasomes,” and “Applications of Novasomes,” targeting literature from 2010 to 2024. Additional searches extended to other electronic databases and Google Scholar. Inclusion criteria restricted studies to those published in English, with a focus on Novasomes’ composition, structure, synthesis, characterization, and applications within the 2010-2024 period on a global scale. Figure 1 presents the PRISMA flow chart detailing the systematic review process.
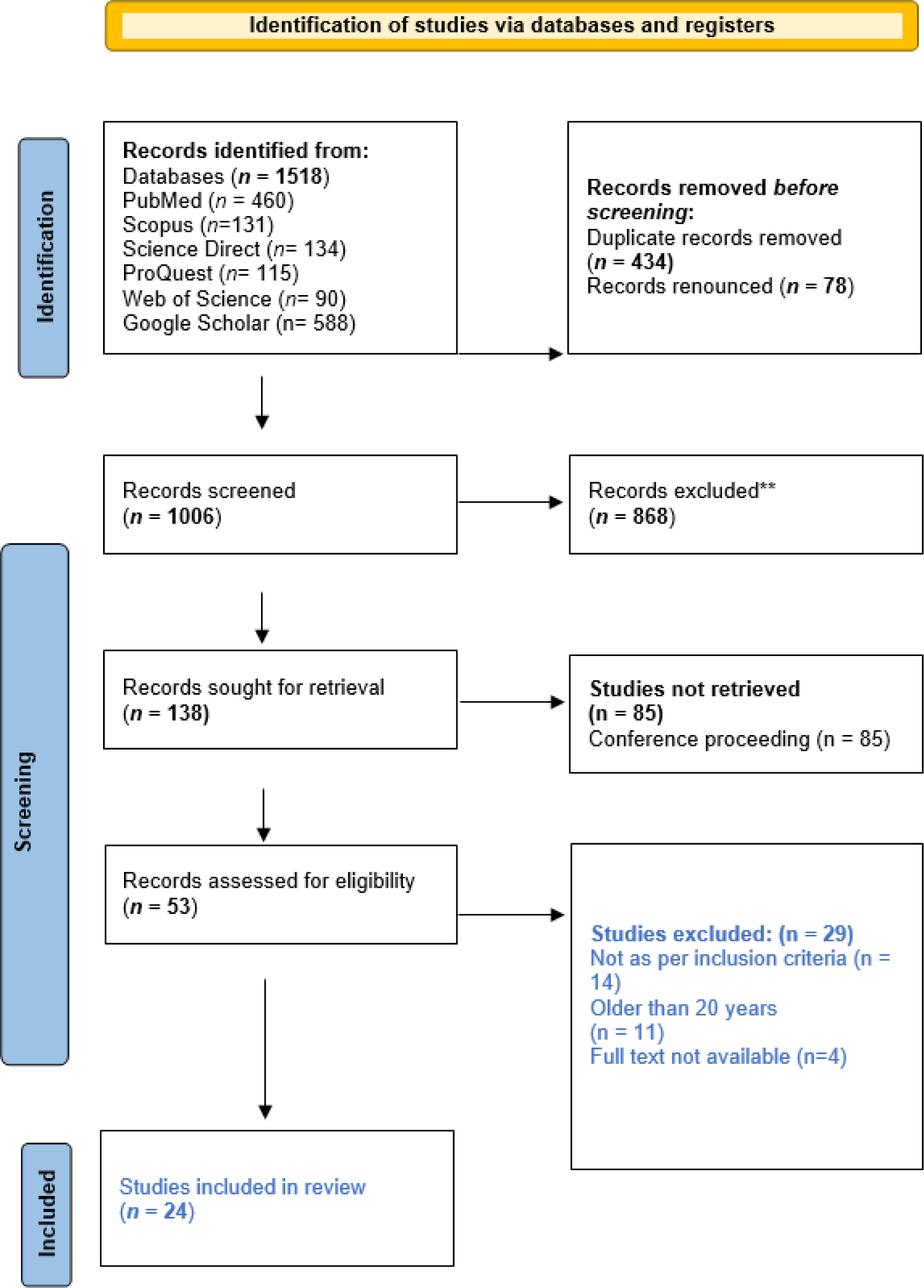
Figure 1.
PRISMA flow diagram for systematic review.
The PRISMA 2020 statement: an updated guideline for reporting systematic reviews. For more information, visit: http://www.prisma-statement.org/
Structure and Composition of Novasomes
A novasome is a vesicle made up of free fatty acids, non-ionic surfactants, and sometimes cholesterol. The presence of non-ionic surfactants alongside free fatty acids is thought to improve transdermal absorption by making the stratum corneum’s lipids more fluid and enhancing the vesicle’s flexibility. The thin-film approach was used to develop free fatty acid-drug loaded novasomes, which are high-quality, non-phospholipid vesicles in the micron size range. These bilayer nanovesicles have a large core and a high encapsulation capacity of ≥80% for both lipophilic and hydrophilic components. Vesicles have an amorphous core filled with insoluble particles like water-insoluble compounds, diamonds, and titanium dioxide. They may contain non-phospholipid surface-active agents, antioxidants, target molecules, aqueous buffers, water-soluble collagen, and other ingredients. In novasome formation, lipid-soluble molecules are placed in the core, while hydrophilic molecules mix with water in the aqueous gap between lipid bilayers. Bilayer membranes include phospholipids and biocompatible single amphiphiles.6 The structure is described in Figure 2.
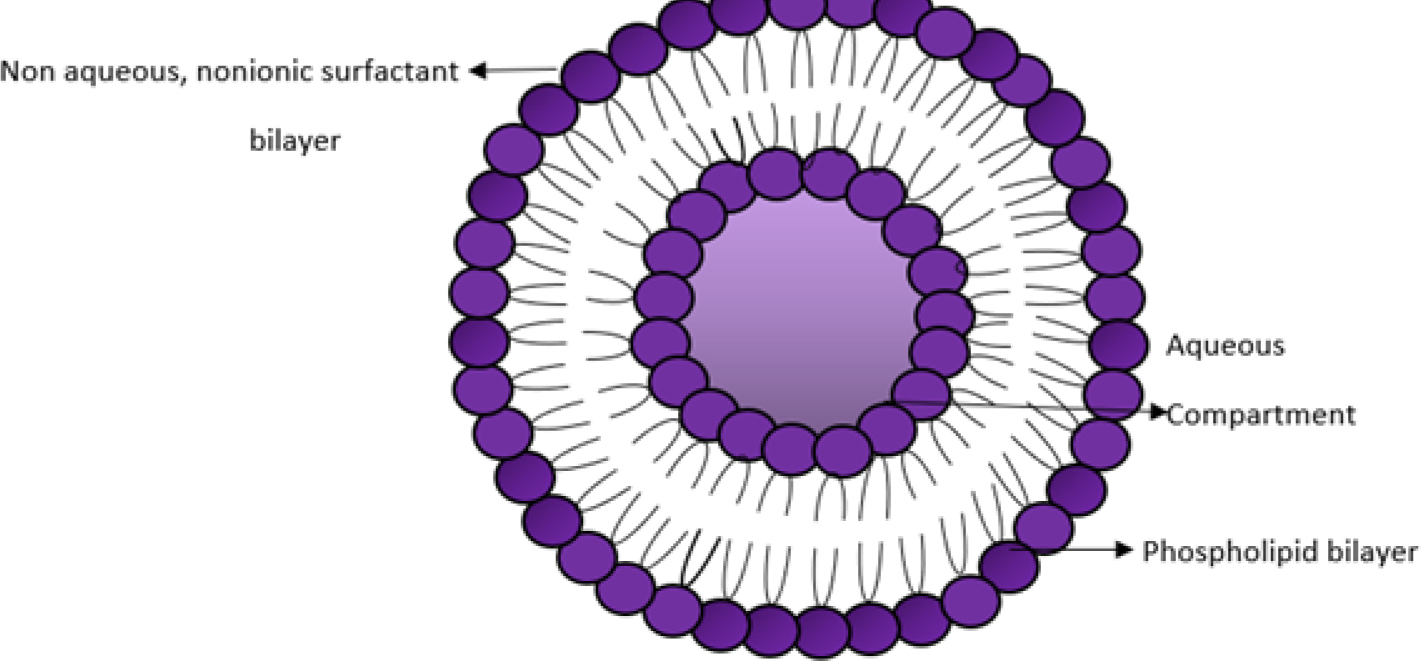
Figure 2.
Structure of Novasome.
Advantages
Novasomes are a unique type of vesicular drug delivery that has several advantages. They are more affordable than liposomes, can deliver a large amount of active ingredient due to their 80% loading efficiency, can be site-specific due to surface charge characteristics, can adhere to the skin or hair shaft, reduce product irritation, and improve stability. They also enable the integration of drugs with interactions between bilayers and can introduce both hydrophobic and hydrophilic ingredients in the same formulation.7
Limitations
Although novasomes are a novel form of vesicular drug administration, they have limitations, including the potential for instability during storage and the challenge of producing them in large quantities. Researchers are actively trying to overcome these constraints by using innovative formulation strategies and quality control procedures. With additional development, novasomes have the potential to transform drug delivery methods and improve patient outcomes.7
Mechanism of Action
The novasome’s bilayers won’t produce the ideal array configuration. It consists of channels (vacancies) that facilitate the movement of the components that are encapsulated (Figure 2). Encapsulated components, such as active chemicals (A) in the central core, migrate laterally between the bilayer vacancies by making a series of arbitrary leaps both inside and between the individual Bilayers (B). This allows a watery suspension (C) to separate the membrane bilayers and release the active substituents continuously from the lipid bilayers. The function of the microvesicle layer is controlled by its net charge, which can be either positive, negative, or neutral. For instance, skin, mucous membranes, or negatively charged hair can be mixed with microvesicles that have a positive charge. Similarly, the configuration of the novasome’s vesicles offers a continuous release mechanism that permits the regulated release of the active ingredient.7
Method of Preparation
Thin Film Hydration Technique: Novasomes are developed through the thin-film hydration method, which involves filling a 10 mL sample with Span 60, stearic acid, methanol/chloroform, and cholesterol in a flask. The organic solvents are evaporated at 40°C for 15 min, forming a translucent thin film. The film is then conditioned in a desiccator under vacuum for 2 hr to eliminate any remaining solvent residue. The film is then combined with pH 7.4 PBS containing the active ingredient. The hydrolysis stage is maintained at 60°C for one hour and rotated at 100 rpm to ensure novasome formation.7 The stepwise process is explained in Figure 3.
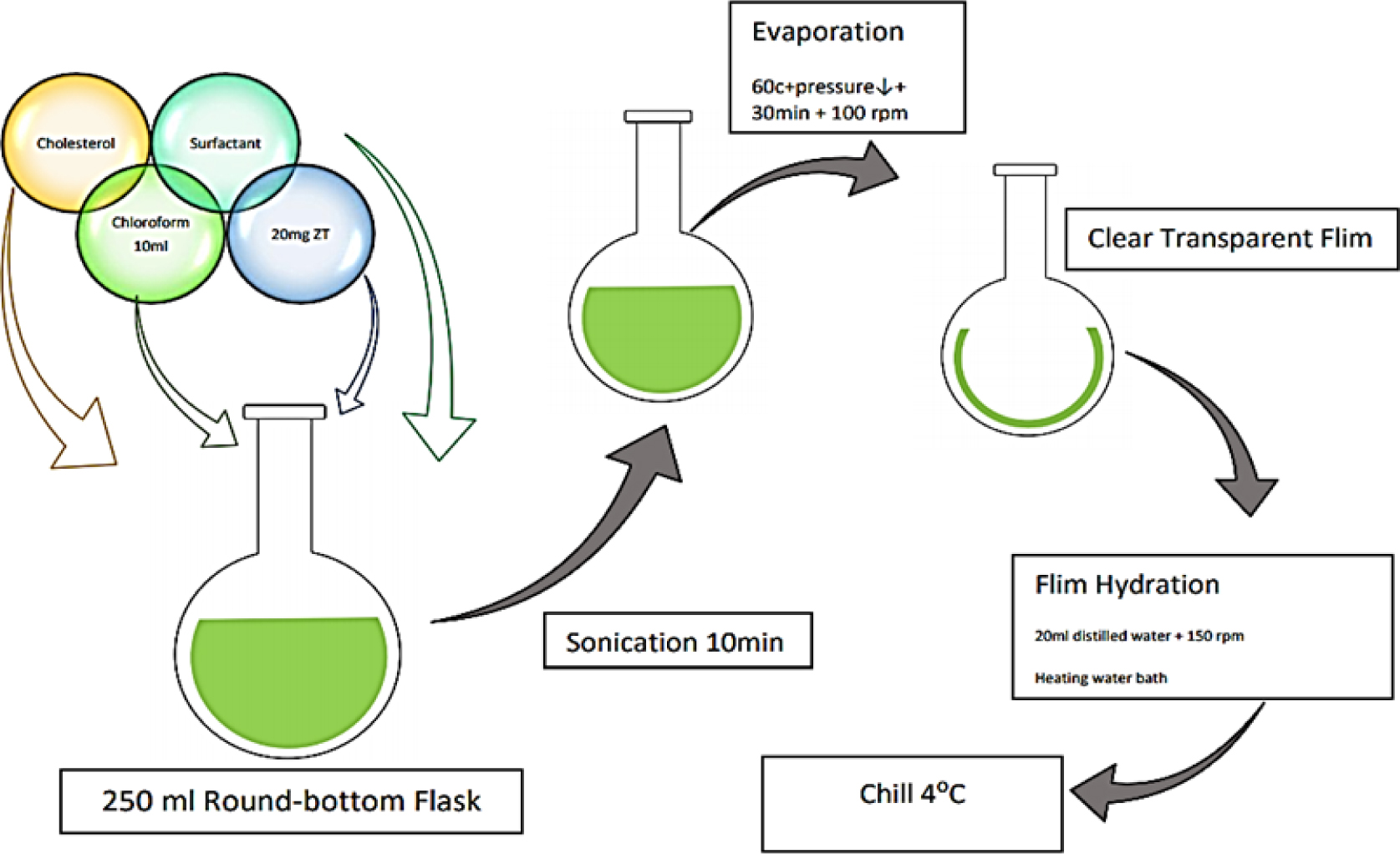
Figure 3.
Thin Film Hydration Method.
Ether Injection Method
The ether injection method is described in Figure 4. Three components are examined: the amount of cholesterol (C) at three different levels, the span 80: drug ratio (B), and the concentration of stearic acid (A). This technique keeps the temperature at 60 degrees Celsius while dissolving the surfactant in diethyl ether. Using an injection with a 14-gauge needle, this solution is introduced into an aqueous drug solution. Ether vaporization results in the formation of single-layered vesicle.8
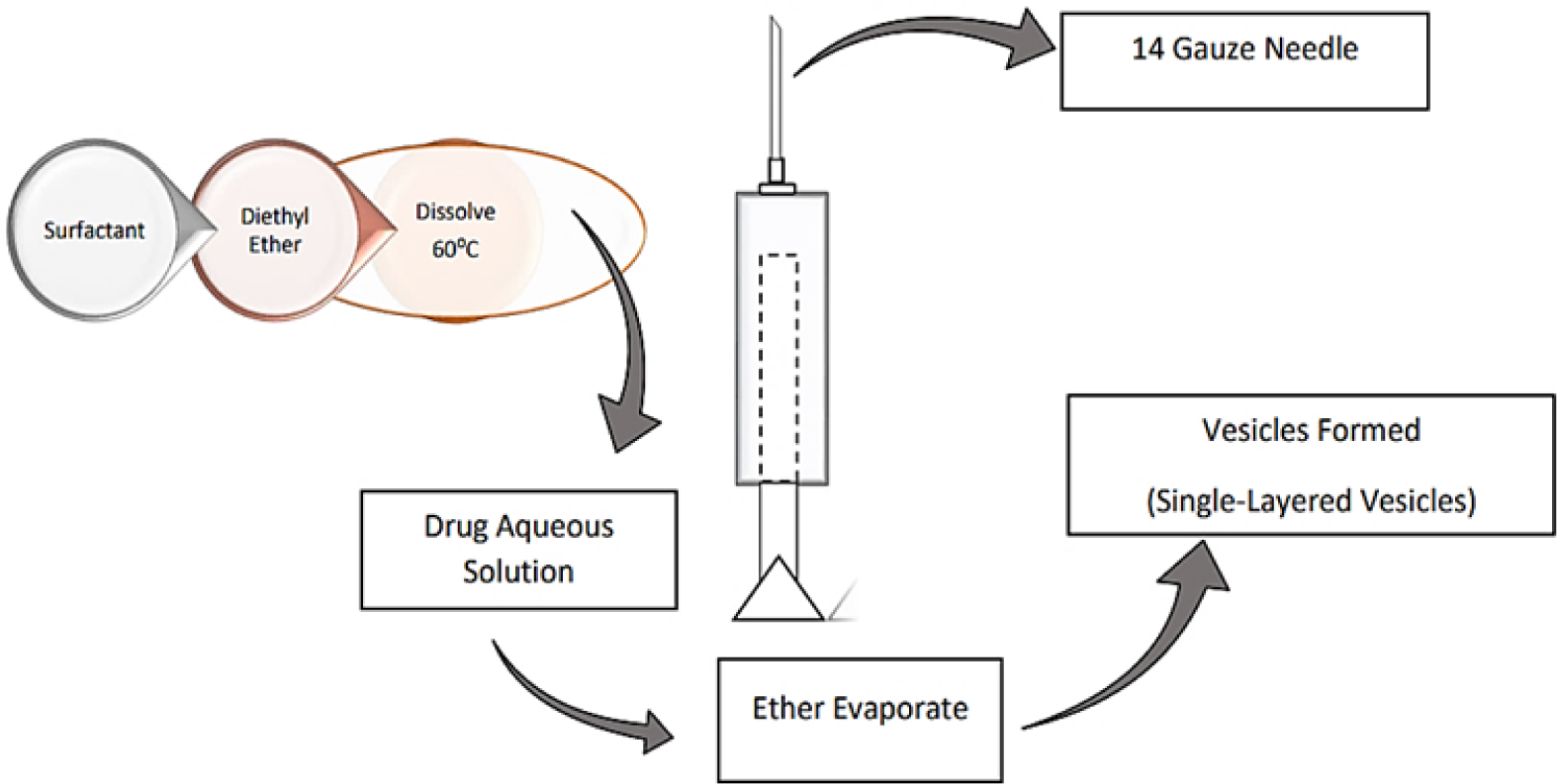
Figure 4.
Ether Injection Method.
Micro Fluidization Method
Microfluidization is a more advanced technique for formulating unilamellar vesicles with a specified size distribution at this point, as shown in Figure 5. This method is based on the submerged jet principle, involving the ultra-high-velocity interaction of two fluidized streams in precisely specified microchannels within an interaction chamber.9
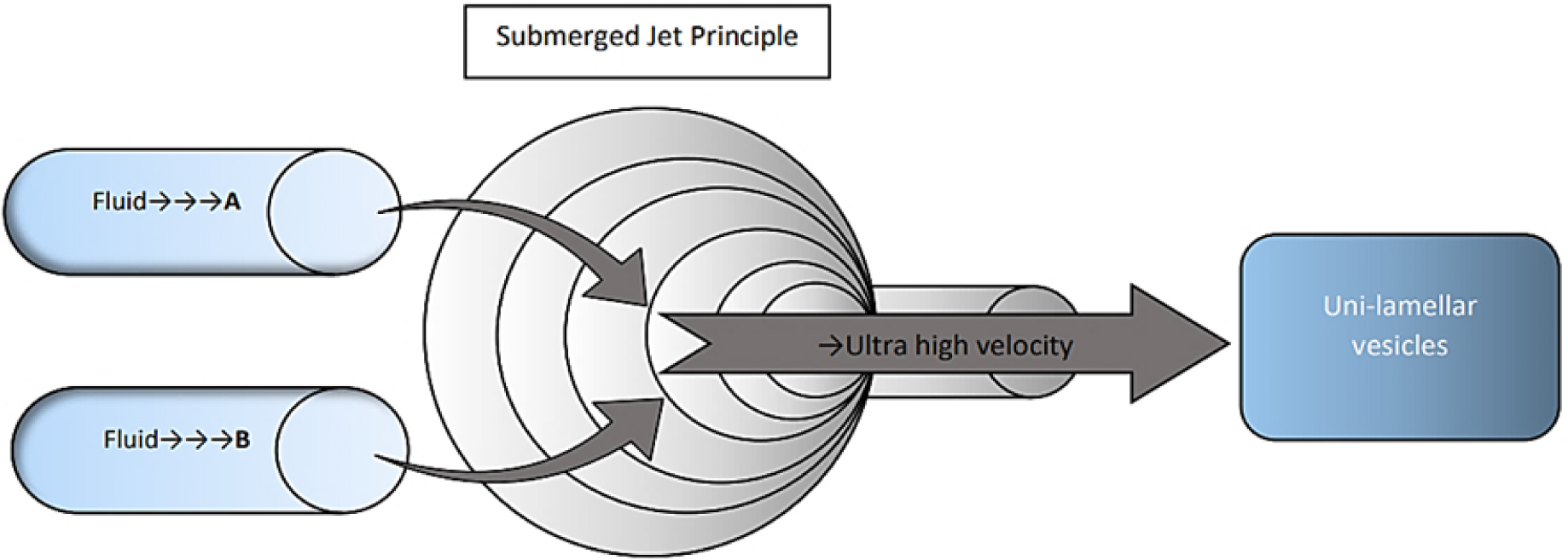
Figure 5.
Micro Fluidization Method.
Hand Shaking Method
The hand-shaking technique, as shown in Figure 6, also known as the thin film hydration method, involves dissolving a mixture of vesicle-forming chemicals (such as cholesterol, and surfactants) in a volatile organic solvent (such as methanol, diethyl ether, or chloroform) within a round-bottom flask. As the solvent is evaporated at room temperature (20°C) using a rotary evaporator, a thin layer of the solidified mixture forms on the flask’s walls. This dried surfactant film is then gently rehydrated with an aqueous phase at temperatures ranging from 0 to 60 degrees Celsius while continuous agitation is applied.10
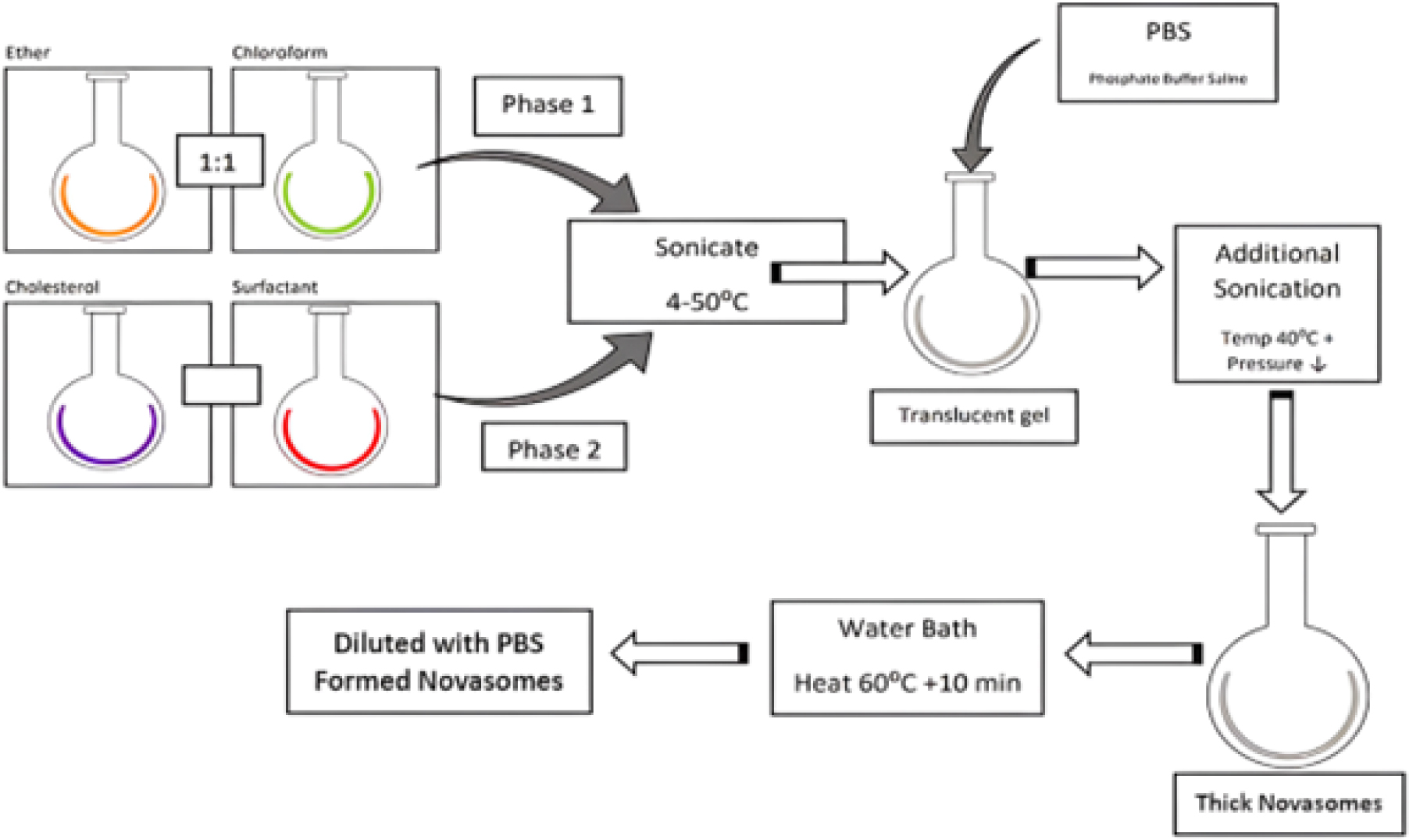
Figure 6.
Hand Shaking Method.
Reverse Phase Evaporation (REV)
The reverse phase evaporation technique is described in Figure 7. A surfactant and cholesterol combination is prepared using a 1:1 mixture of chloroform and ether. The drug is loaded into the aqueous phase, which is sonicated at 4°C-50°C. A translucent gel is formed and further sonicated. The temperature is raised to 40°C, and pressure is reduced to remove the volatile organic phase. This result in a concentrated suspension of novasomes, which can be heated in a water bath at 60°C for 10 min, diluted with PBS, and transformed into Novasomes.11
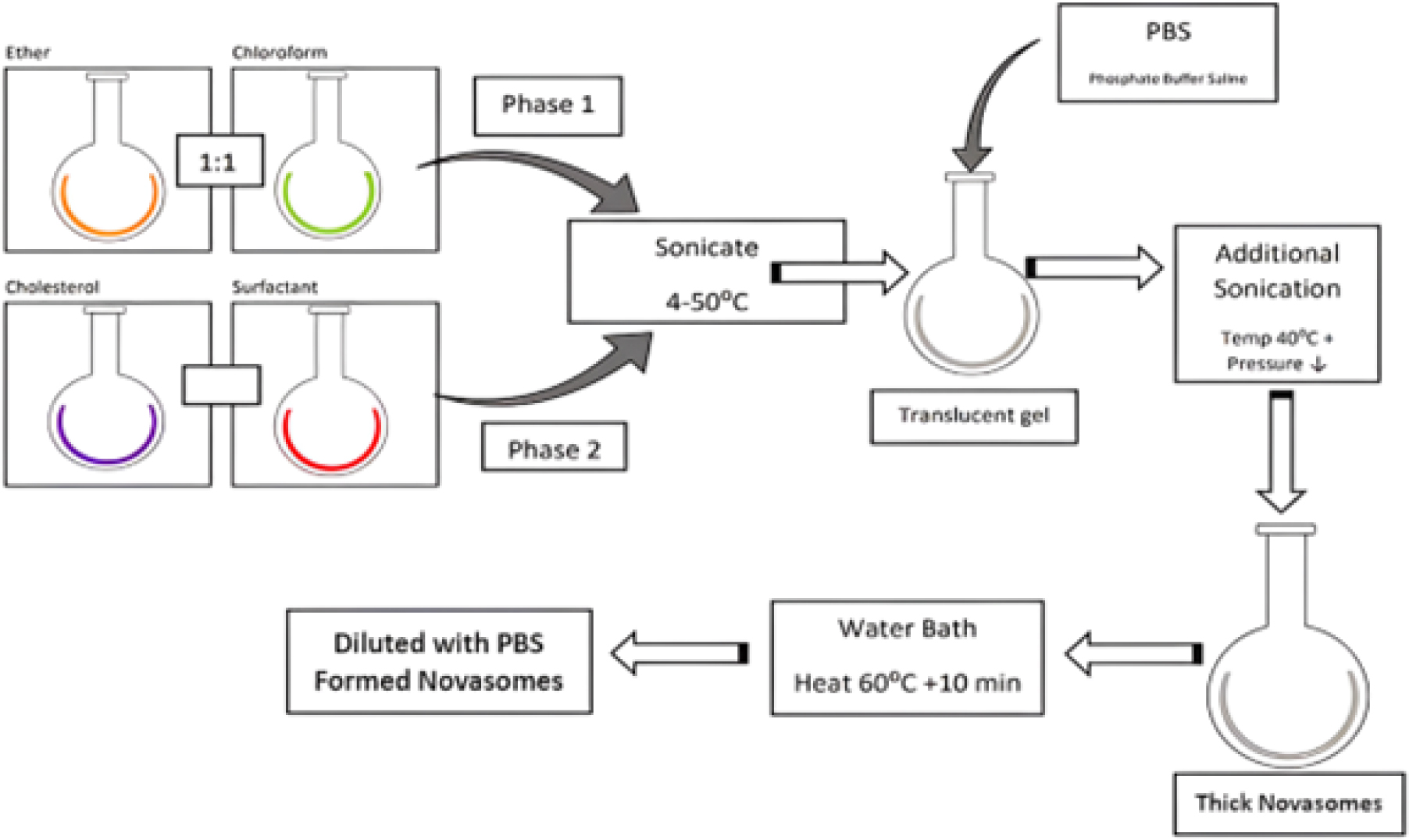
Figure 7.
Reverse Phase Evaporation Method.
Multiple Membrane Extrusion Method
In the multiple membrane extrusion method, a thin layer is produced by evaporating a solution of surfactant cholesterol along with diacetyl phosphate in chloroform. Aqueous drug polycarbonate membranes are primarily used to dampen the film. The resultant solution and suspension are then forced out through the membranes and arranged in a series of up to eight channels. This method effectively regulates the size of the particles generated.11 The process is described in Figure 8.
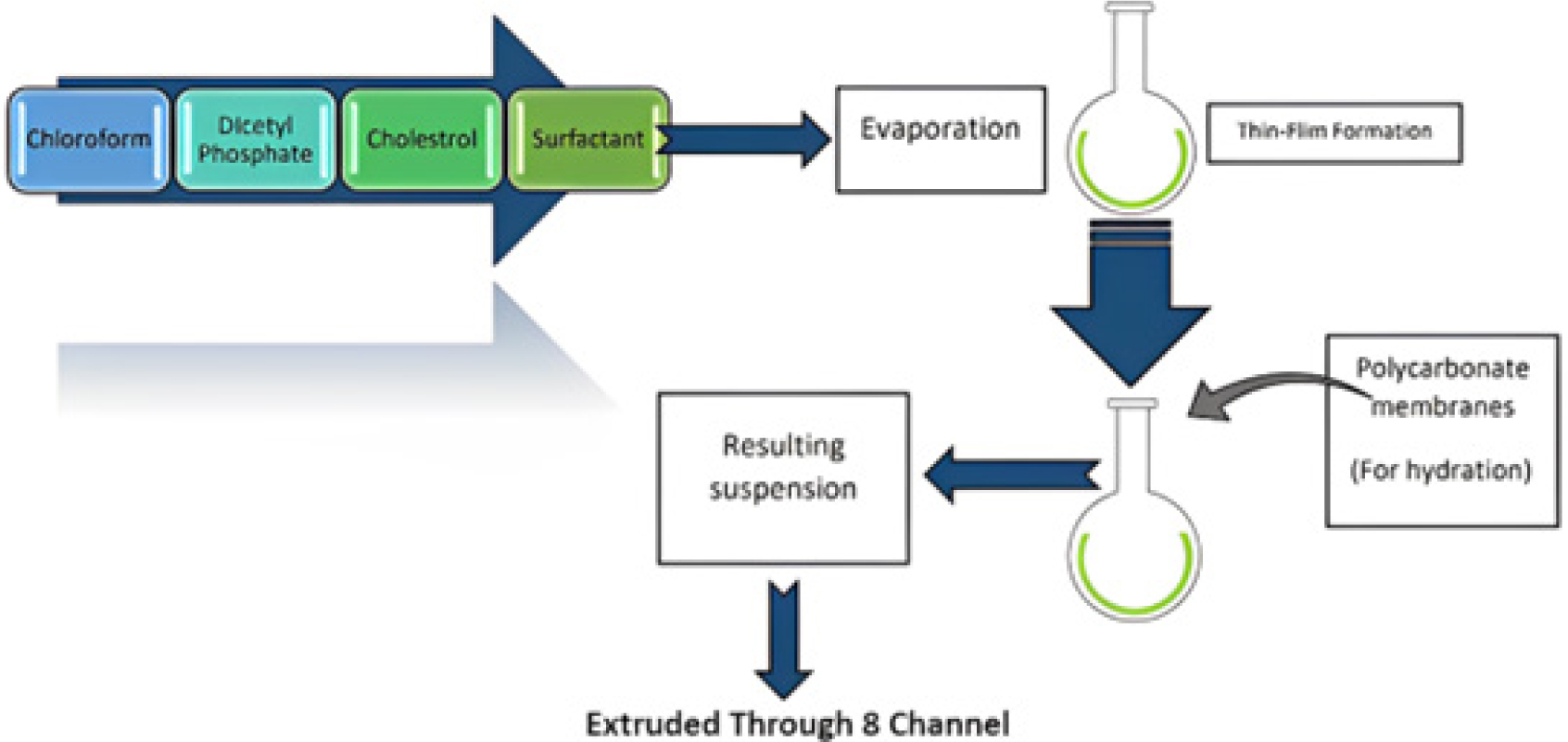
Figure 8.
Multiple Membrane Extrusion Method.
Sonication Method
Sonication, as shown in Figure 9, is an alternative method for developing novasomal vesicles. In this process, a 10 mL glass vial is filled with a mixture of cholesterol or surfactant and a portion of the drug solution in a buffer. The mixture is then subjected to sonication using a titanium probe at 60°C for 3 min, resulting in the final product.12
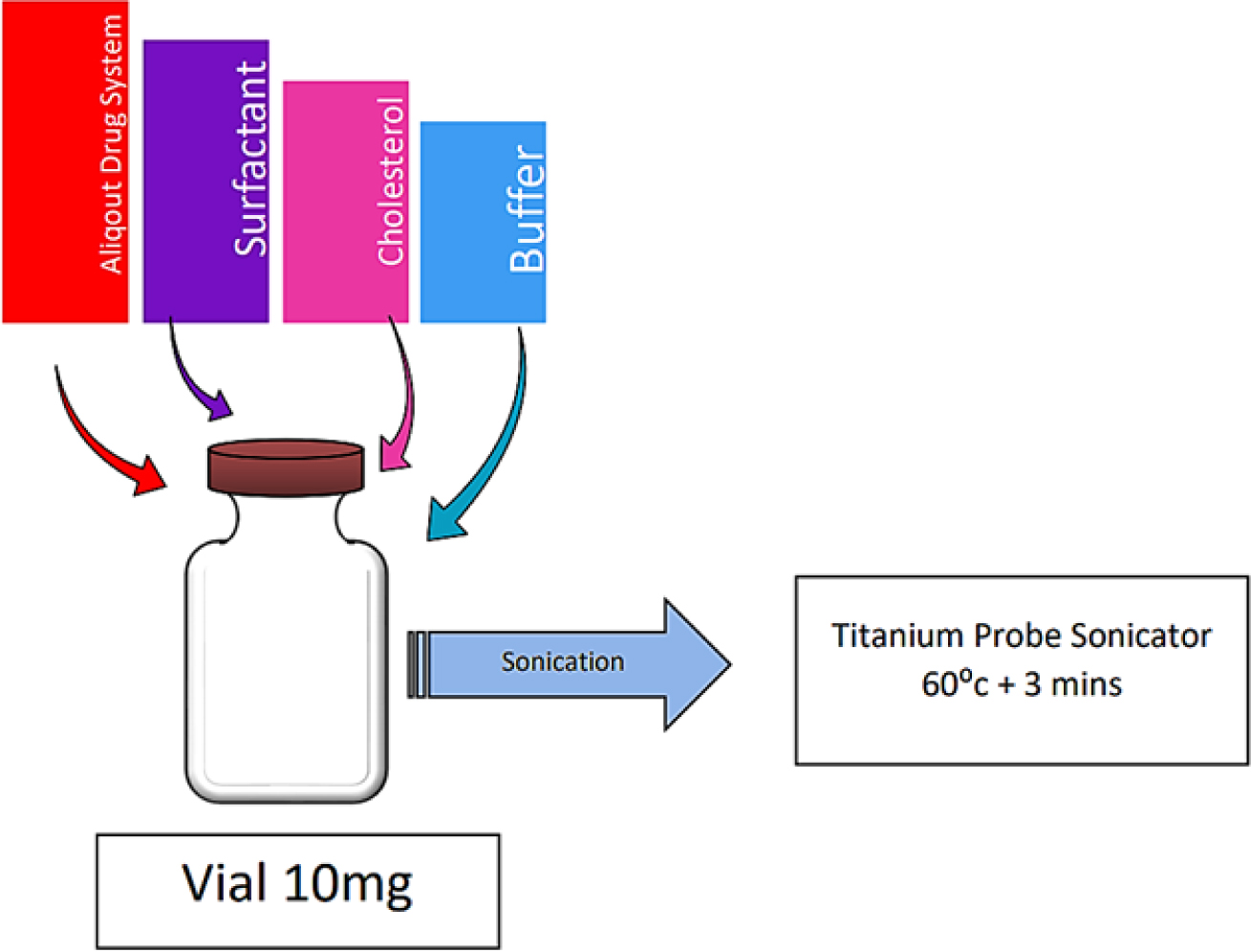
Figure 9.
Sonication Method.
Characterization Techniques
Fourier-Transform Infrared Spectroscopy (FTIR)
A disc is created by compressing each sample with 2-3 mg of dry potassium bromide and testing it in the 4000-500 cm-1 range at 25±2°C.12
Differential Scanning Calorimetry
The optimized drug-loaded novasomes are converted into a dry powder through lyophilization. Samples weighing 4 mg were examined at a heating rate of 10°C per min in sealed aluminum pans, with an inert nitrogen flow of 25 mL/min applied over a temperature range of 30 to 500°C.13
Permeation Studies
The nasal mucosa of freshly sliced sheep was treated with novasomes on its mucosal surface. The 100 mL of bicarbonate Ringer solution, kept at the pH of 7.4, is placed in the beaker and swirled at 150 rpm and 37°C. The UV-visible spectrophotometer is used to calculate absorbance data.13
Mucoadhesive Studies
The nasal mucosa of the sheep sheet is placed on a glass slide, onto which the nasal mucosa is bonded using cyanoacrylate adhesive. The novasomes (10 mg apiece) were pressed with the fingers to adhere to the membrane of the nasal mucosa. A USP disintegration device type II is applied to a mucosa. The 50 rpm simulated nasal fluid with a pH of 7.4 at 37°C.14
Zeta Potential and Particle Size Analysis
The Zeta-sizer 2000 is used to identify the zeta potential and particle size of drug-carried novasomes. The novasome formulations are diluted before analysis at room temperature, and the mean value is recorded. Zeta potential is determined using photon correlation spectroscopy in 10 mm cells at 25°C with Malvern Zetasizer Nano. The zeta potential of the drug-carried novasome vesicles is monitored by electrophoretic mobility in an electric field before ZP measurements.14
Determination of Entrapment Efficiency (EE%)
Place 1 mL of the dispersion into the upper chamber of a centrifuge tube fitted with an ultrafilter. Centrifuge the mixture at 6000 rpm for 30 min and analyze using a spectrophotometer. The following equation is used to compute the EE%

Electron Microscopy
A drop of the diluted novasome dispersion was placed onto a carbon-coated copper grid, and a round cell was applied to the carbon substrate to absorb for 1 min. Next, a drop of 1% (w/v) phosphotungstic acid was added. After air drying at room temperature, the negatively stained sample was imaged at 80 kV.15
In vitro Drug Release
The drug release study was conducted using a Dissolution Apparatus II and a cellulose dialysis membrane pre-soaked in PBS (pH 7.4). Glass tubes containing a fixed amount of novasomes or drug were sealed with the cellulose membrane and attached to a rotating shaft. These tubes were then immersed in 500 mL of PBS, maintained at 37°C, and stirred continuously at 50 rpm. Samples were collected at intervals ranging from 0.5 to 24 hr, and drug release was measured using a UV-visible spectrophotometer.16
Release Kinetics
Various statistical models, such as the zero-order model, Higuchi model, Hixon-Crowell model, first-order model, and Korsmeyer-Peppas model, are employed to analyze the formulations’ release kinetics.16
Cell Viability Test
The cytotoxicity of novasomes is assessed using the MCF-7 cell line. Cells are cultured in 96-well plates with DMEM, FBS, and a passage number of 20. After 24 hr, the growth medium is replaced with a serum-free medium. MTT solution is added, and cells are incubated for an hour. Formazan crystals are dissolved with DMSO, and absorbance is measured.17
Forced Swimming Test
The study involved rats given a standard antidepressant orally and nasally, and a control group given deionized water. After an hour, rats were placed in a glass beaker containing tap water at 23°C. The water was replaced for each trial, and the rats’ activity was recorded. Immobility was used to assess depressive-like behavior, as rats exhibited minimal movements to keep their heads above the water.17
Tail Suspension Test
The study involved administering a reference drug orally to a reference group, while the test group received the sample via nasal route. A control group received 1 mL of deionized water via a nasal spray. After an hour, rats were suspended 50 cm above the floor with adhesive tape. Video recordings were taken, and immobility was observed for 6 min out of a 10-min session.18
Determination of Minimum Inhibitory Concentration (MIC) using the XTT reduction technique
The Minimum Inhibitory Concentration (MIC) for Candida albicans was determined using a micro-well dilution assay. The inoculum concentration was adjusted to 106 CFU/mL. In a 96-well plate, suspension, unmedicated novasomes, and drug-loaded novasomes were diluted threefold with DMSO. Each well received 50 μL of diluted solutions, 10 μL of inoculum, and 40 μL of brain heart infusion medium. The plates were incubated at 37°C for 24 hr, then added 40 μL of tetrazolium salt solution and incubated for an additional hour. The reduction of XTT was measured and the percentage of inhibition was calculated.19

Pharmacokinetic study
In the pharmacokinetic study, twelve rats were allocated into three groups of four. After administering intraperitoneal anesthesia, groups B and C received 100 μL of Total Base Number (TBN) solution in PBS (pH 7.4) and an optimized novasome suspension, respectively, via intraperitoneal injection. Group A was administered 1 mL of oral TBN solution in PBS (pH 7.4) at a concentration of 200 μg/mL. Following the intraperitoneal injections, 50 μL of 0.9% saline was used to rinse the Microsprayer® tubing and syringe. Blood samples (500 μL) were drawn from the retro-orbital venous plexus of each rat at 0.5, 1, 2, 4, 8, and 24 hr. The samples were centrifuged at 3000 rpm for 15 min to isolate the plasma, which was then stored at -20°C until further analysis. Plasma drug concentrations were determined using Liquid Chromatography-Mass Spectrometry (LC-MS/MS), with data analysis performed using Shimadzu Controller Version Analyst 1.19
Statistical analysis
Pharmacokinetic data were evaluated using one-way ANOVA, with posthoc comparisons conducted via the Tukey test. The data were processed with SPSS 22 and presented as mean±SD. Statistical analysis was carried out using GraphPad Prism 8.0.1 software. ANOVA, along with Tukey’s or Dunnett’s posthoc tests, was used to determine the significance of differences between formulations. A p-value of less than 0.05 was deemed statistically significant. Data analysis was further conducted with SPSS version 25.0 to assess differences between treatments.20
Stability study of Novasomes
The study tests the stability of novasomes by storing them at 4°C for three months and observing their attributes every 0, 30, 60, and 90 days. Samples are evaluated for physical traits at pre-arranged intervals, and mean values of entrapment efficiency, zeta potential, and particle size are computed and presented.20
Novasomes storage condition
Three portions of optimal samples are separated and put into three vials (15 mL each). One sample is kept at ambient temperature and exposed to sunshine, while the other 2 flasks are wrapped with aluminum foil and refrigerated at -4°C. Zeta potential, particle size, and in vitro drug release analyses of novasomes are conducted both at the time of analysis and at 1, 3, and 6 months later.21
Applications
Ocular drug delivery
The human eye, a delicate organ with complex anatomy, is susceptible to various ophthalmic conditions, requiring appropriate treatment with Novasomes.22
Enhanced Brain Targeting
Novasomes enhance brain targeting in intranasal preparations, such as nasal administration of zolmitriptan for migraine treatment. They also serve as carriers for topical thyroid hormone, accelerating wound healing in rats, and acting as adjuvants for immunization.22
Nasal drug delivery
Nasal delivery is expected to increase the drug’s bioavailability by bypassing pre-systemic metabolism. The medication’s rapid absorption due to the high vasculature and presence of microvilli in the nasal cavity is anticipated.22
Nanoparticle delivery
The novasomes have extensive applications in nanoparticle drug delivery. It offers a greater payload capacity compared to liposomes, enhances product stability, and extends the product’s shelf life. Additionally, it applies to a range of products.23
Enhanced Stability
Novasomes aid in formulation, expand action site, ensure chemical stability, cost-effectiveness, large availability, and can carry nutrients, flavor oils, and active components
Enhanced Penetration
Numerous vaccines using novasomes have received approval. In the current work, Brij® V was incorporated as an additional penetration enhancer into the structure of novasomes to produce Transnovasomes (TNs).23
Enhanced Immunogenicity
Novasomes, used in various fields like personal care, cosmetics, food, dermatology, and chemicals, improve immunogenicity and protection. They have been used in smallpox vaccines, Avian
Rheovirus, and Newcastle Disease vaccines. They also improve the topical dispersion of minoxidil.24
Treatment of tinea corporis
Transnovasomes containing fenticonazole nitrate are used to treat tinea corporis. Novasomes serve as an excellent vesicular carrier system for terconazole in the treatment of fungal skin infections.24
CONCLUSION
In conclusion, advancements in pharmaceutical technology have led to the development of novasomes, an innovative approach to drug delivery. The novasome formulation, designed for optimal size and entrapment efficiency, shows promise for transdermal and nasal delivery systems. Novasomes improve drug release control and encapsulation, addressing the limitations of traditional liposomal systems. These nanoparticles enhance drug formulations and targeted delivery, contributing to personalized medicine and efficient drug distribution. Novasomes offer high capacity, stability across a wide pH range and temperature, and effective encapsulation of active ingredients. They are characterized by their unique multi-bilayered structure and ability to maintain stability and efficacy. The technology provides numerous advantages, including improved formulation, stability, and scalability, and offers potential for broader applications in pharmaceuticals, cosmetics, and agriculture. Continued research into novasomes could significantly improve drug delivery systems, extending product shelf life and enhancing overall efficacy and safety.
Cite this article:
Mustafa MB, Rasheed N, Ayesha M, Ain Q, Mughal M, et al., Novasomes: A Versatile Drug Delivery System for Enhanced Therapeutic Efficacy. J Young Pharm. 2025;17(1):36-45.
ACKNOWLEDGEMENT
We want to extend our sincere gratitude to the Deanship of the Faculty of Pharmaceutical Sciences at Lahore University of Biological and Applied Sciences (UBAS) for providing the necessary resources and support that made this research possible. Their commitment to fostering academic excellence has been invaluable.
ABBREVIATIONS
DSC | Differential scanning calorimetry |
---|---|
FTIR | Fourier- transform infrared spectroscopy |
PBS | Phosphate Buffer Solution |
EE | Entrapment Efficiency |
MTT | 3-(4,5-Dimethylthiazol-2-yl)-2,5- Diphenyltetrazolium Bromide |
FBS | Fetal bovine serum |
DMEM | Dulbeccos Modified Eagle Medium |
DMSO | Dimethyl sulfoxide |
XTT | 2,3-bis- (2-methoxy-4-nitro-5- sulfophenyl)-(2H)-tetrazolium-5- carboxanilide |
TBN | Total Base Number |
MIC | Minimum Inhibitory Concentration |
TNs | Transnovasomes |
References
- Singh A, Malviya R, Sharma PK. Novasome-A breakthrough in pharmaceutical technology a review article. Adv Biol Res (Rennes). 2011;5(4):184-9. [Google Scholar]
- Elkomy MH, El Menshawe SF, Kharshoum RM, Abdeltwab AM, Hussein RR, Hamad DS, et al. Innovative pulmonary targeting of terbutaline sulfate-laded novasomes for non-invasive tackling of asthma: statistical optimization and comparative / evaluation. Drug Deliv. 2022;29(1):2058-71. [PubMed] | [CrossRef] | [Google Scholar]
- Waghmare S, Patil A, Patil P. Novasome: advance in liposome and niosome. J Pharm Innov. 2016;5(5):34-8. [PubMed] | [CrossRef] | [Google Scholar]
- Abd-Elal RM, Shamma RN, Rashed HM, Bendas ER. Trans-nasal zolmitriptan novasomes: preparation, optimization and evaluation of brain targeting efficiency. Drug Deliv. 2016;23(9):3374-86. [PubMed] | [CrossRef] | [Google Scholar]
- Paudwal G, Banjare N, Gupta PN. Nanovesicles for nasal drug delivery. Applications of Nanovesicular drug delivery. 2022:81-101. [PubMed] | [CrossRef] | [Google Scholar]
- Tawfik MA, Mohamed MI, Tadros MI, El-Helaly SN. Low-frequency sonophoresis as an active approach to potentiate the transdermal delivery of agomelatine-loaded novasomes: design, optimization, and pharmacokinetic profiling in rabbits. AAPS PharmSciTech. 2021;22(8):261 [PubMed] | [CrossRef] | [Google Scholar]
- Aslam Abdul Rahiman CA, Krishnan K, Sreelekshmi AS, Arjun KK, Nair SC. Novasome: A pioneering advancement in vesicular drug delivery. Int J Appl Pharm. 2021;13(1):59-64. [PubMed] | [CrossRef] | [Google Scholar]
- Abdelbari MA, El-Gazar AA, Abdelbary AA, Elshafeey AH, Mosallam S. Investigating the potential of novasomes in improving the trans-tympanic delivery of niflumic acid for effective treatment of acute otitis media. J Drug Deliv Sci Technol. 2024;98:105912 [CrossRef] | [Google Scholar]
- Agarwal S, Kumari PV. Advances in novasome technology-a review. Int J Appl Pharm. 2013;5(1):1-4. [CrossRef] | [Google Scholar]
- Fareed NY, Kassab HJ. A comparative study of oral diacerein and transdermal diacerein as Novasomal gel in a model of MIA induced osteoarthritis in rats. Pharmacia. 2023;70(4):1363-71. [CrossRef] | [Google Scholar]
- Aboud HM, Hussein AK, Zayan AZ, Makram TS, Sarhan MO, El-Sharawy DM, et al. Tailoring of Selenium-Plated Novasomes for Fine-Tuning pharmacokinetic and Tumor Uptake of quercetin: Optimization and Radio biodistribution Assessment in Ehrlich Tumor-Bearing Mice. Pharmaceutics. 2022;14(4) [CrossRef] | [Google Scholar]
- Din FU, Aman W, Ullah I, Qureshi OS, Mustapha O, Shafique S, et al. Effective use of nanocarriers as drug delivery systems for the treatment of selected tumors. Int J Nanomedicine. 2017;12:7291-309. [PubMed] | [CrossRef] | [Google Scholar]
- Ahmed S, Amin MM, El-Korany SM, Sayed S. Corneal targeted fenticonazole nitrate-loaded novasomes for the management of ocular candidiasis: preparation, characterization, and assessments. Drug Deliv. 2022;29(1):2428-41. [PubMed] | [CrossRef] | [Google Scholar]
- Gulshan S, Shah S, Shah PA, Irfan M, Saadullah M, Abbas G, et al. Development and pharmacokinetic evaluation of novasomes for the trans-nasal delivery of fluvoxamine using arachidonic acid-carboxymethyl chitosan conjugate. Pharmaceutics. 2023;15(9) [PubMed] | [CrossRef] | [Google Scholar]
- Fatima I, Rasul A, Shah S, Saadullah M, Islam N, Khames A, et al. Novasomes as nano-vesicular carriers to enhance topical delivery of fluconazole: A new approach to treat fungal infections. Molecules. 2022;27(9):2936 [PubMed] | [CrossRef] | [Google Scholar]
- Albash R, Ragaie MH, Hassab MA, El-Haggar R, Eldehna WM, Al-Rashood ST, et al. Fenticonazole nitrate loaded trans-novasomes for effective management of tinea corporis: design characterization, in silico study, and exploratory clinical appraisal. Drug Deliv. 2022;29(1):1100-11. [PubMed] | [CrossRef] | [Google Scholar]
- Rukari TG, Pingale PL, Upasani CD. optimizing novasomes: impact of oleic acid and co-surfactant ratio on posaconazole delivery: and pharmacokinetic study. JOAPR. 2024;12(3):88-98. [PubMed] | [CrossRef] | [Google Scholar]
- Gupta RK, Varanelli CL, Griffin P, Wallach DF, Siber GR. Adjuvant properties of non-phospholipid liposomes (Novasomes®) in experimental animals for human vaccine antigens. Vaccine. 1996;14(3):219-25. [PubMed] | [CrossRef] | [Google Scholar]
- Mosallam S, Ragaie MH, Moftah NH, Elshafeey AH, Abdelbary AA. Use of novasomes as a vesicular carrier for improving the topical delivery of terconazole: in vitro characterization, in vivo assessment and exploratory clinical experimentation. Int J Nanomedicine. 2021;16:119-32. [PubMed] | [CrossRef] | [Google Scholar]
- ElShagea HN, Makar RR, Salama AH, Elkasabgy NA, Salama A, Basalious EB, et al. Terpene-augmented novasomal gels for the sustainment of rasagiline mesylate delivery; A new approach for treating Parkinson’s disease induced by rotenone in rats. J Drug Deliv Sci Technol. 2024;92:105369 [CrossRef] | [Google Scholar]
- Ali AA, Hassan AH, Eissa EM, Aboud HM. Response surface optimization of ultra-elastic nanovesicles loaded with deflazacort tailored for transdermal delivery: accentuated bioavailability and anti-inflammatory efficacy. Int J Nanomedicine. 2021;16:591-607. [PubMed] | [CrossRef] | [Google Scholar]
- Deshmukh PK, Deshmukh PK, Jain SN, Patil PO, Pardeshi CV. Vesicular carriers for direct nose-to-brain drug delivery. Direct nose-to-brain drug delivery. 2021:209-23. [PubMed] | [CrossRef] | [Google Scholar]
- Zhu H, Yang C, Ma K. Nanovesicles for transdermal drug delivery. Appl Nanovesicular Drug Deliv. 2022:103-14. [PubMed] | [CrossRef] | [Google Scholar]
- Fatima I, Rasul A, Shah S, Saadullah M, Islam N, Khames A, et al. Novasomes as nano-vesicular carriers to enhance topical delivery of fluconazole: A new approach to treat fungal infections. Molecules. 2022;27(9):2936 [PubMed] | [CrossRef] | [Google Scholar]