ABSTRACT
The production of nanoparticles with the use of microbes is a technology that is environment friendly. This paper highlights the use of a syndicate of various micro-organisms (bacteria, actinomycetes, fungi, and algae) for the amalgamation of various metallic and metal oxide nanoparticles. When compared to physical and chemical methods, which are expensive, complicated, hazardous, and produce toxic wastes that are detrimental to the environment and the health of a variety of living beings, the synthesis of nanoparticles through the biological route is not only inexpensive, but also environmentally friendly, sustainable, and does not require a significant amount of energy to complete. This is in contrast to the fact that the physical and chemical methods are responsible for producing nanoparticles, which is an energy-intensive Nanoparticles can be biosynthesized via extracellular or intracellular pathways, depending on the particular circumstances. Capping, enzymatic reduction, and metal capture are the three steps that are involved in the process of nanoparticle production by micro-organisms. The organisms as well as the parameters that are utilised, such as temperature, pH and substrate concentration, all play a role in determining the size and form of the nanoparticles. The biogenesis of these nanoparticles takes place in a short amount of time and results in particles that have the right size and form. These nanoparticles are reliable and risk-free, and they have a wide range of applications in a variety of fields, including drug administration, cancer treatment, gene therapy, agricultural biosensors, the cosmetics sector, and many others.
INTRODUCTION
Richard Feynman gave the infamous speech “There’s a plenty of room at the bottom” at the American Institute of Technology, which is where the notion of nanotechnology was firstly presented. The word “nano” from nanotechnology is derived from the Greek word nanos, which means “dwarf ”. In order to generate desired features and functionalities and for a variety of applications, nanotechnology involves removing portions of matter’s structure at the atomic, molecular and supramolecular levels that range in size from 1 to 100 nanometres. Numerous industries, including drug delivery and other biomedical ones, have been affected by nanotechnology.1–5 Nanoparticles are manufactured by chemical, physical and biological methods. The physical methods include crushing, grinding, pyrolysis, milling, ball processing, etc. On the other hand, sol-gel process, irradiation reduction, electrolysis, ultrasonic assisted reduction, etc. For the mass fabrication of nanoparticles, physical techniques are subpar. Due to their higher energy requirements, higher input costs, and poorer yield of NPs, physical techniques are less effective for producing nanomaterials on a wide scale.6 Higher energy requirements and input costs are the main contributors to the reduced NPs yield. Because they use less energy during the reduction of metals and the creation of homologous NPs with high accuracy, chemical approaches to NP synthesis have been popular in recent years.7 These conventional techniques for homogenous NPs therefore have good accuracy. Traditional methods of NPs synthesis are time-consuming, laborious, hazardous, antiquated, expensive, inefficient, and based on the use of unsafe (cytotoxic, genotoxic, and carcinogenic) toxic chemicals, which are act as powerful environmental pollutants. Modern methods of NPs synthesis are based on the use of non-hazardous, up-to-date, cost-effective alternatives.8,9,19 Additionally, the biomedical uses of NPs made from pollutants. Additionally, due to their instability and hazardous character, the biomedical applications of NPs synthesised through chemosynthesis have been restricted. Therefore, it is crucial to create dependable, non-toxic, and environmentally friendly technologies for synthesising nanoparticles in order to increase the scope of their biomedical applications. Using microbes to create nanoparticles is one way to accomplish this. According to research, microbes are among the unique resources that have the greatest potential for manufacturing nanoparticles.10–19 With the use of microbes, the usage of pricey chemicals is removed, and the increasingly popular “green” approach uses less energy and is better for the environment than the chemical approach. The bulk of the bacteria live in environments with a variety of temperature, pH, and pressure, which lends support to the “biogenic” theory. Due to the bacterial carrier Matri, the particles produced by these procedures have better catalytic reactivity, more surface area, and improved interaction with the relevant metal salt.20,21 A wide variety of applications for the biosynthesized nanoparticles have been created, such as pharmaceutical carriers for targeted delivery, gene therapy, DNA analysis, cancer treatment, antibacterial agents, biosensors, separation science, Magnetic Resonance Imaging (MRI) and so on. This approach provides a succinct rundown of techniques for micro-organisms to produce nanoparticles, followed by a discussion of applications.
MECHANISM OF NANOPARTICLES SYNTHESIS
Numerous micro-organisms either create inorganic compounds inside or outside of the cell. But with various biological agents, the mechanism of nanoparticle synthesis differs for intra and extracellular. The intracellular technique makes use of a unique ion transport system within the microbial cell. The cell wall of the micro-organisms is crucial in the intracellular creation of nanoparticles. The microbial cultures are kept alive in the proper growth medium with favourable pH and temperature conditions, and the microbial biomass is cleaned with sterile distilled water before being centrifuged to produce the pellet of biomass.22–24 Then, an aqueous metal solution is introduced to the microbial biomass. The mixture of metals and microbial biomass is then grown under the desired incubation conditions until a particular chromatic shift is noticed. The development of NPs is indicated by the emergence of a certain hue. An appearance of a pale yellow to yellow tint denotes the synthesis of manganese and zinc nanoparticles. While a pale yellow to pinkish appearance suggests the synthesis of gold nanoparticles, a pale yellow to brownish appearance suggests the synthesis of silver nanoparticles.25 At this stage, the negatively charged cell walls of micro-organisms’ trap positively charged metal ions. Enzymes bio reduce these metal ions within the cell wall, forming nanoclusters along the way before the NPs finally diffuse out of the cell wall and into the solution.26 Therefore, the microbial cells absorb the metal ions throughout the intracellular process, and in the presence of cellular enzymes, the metal ions are changed into NPs inside the cell as shown in Figure 1.27
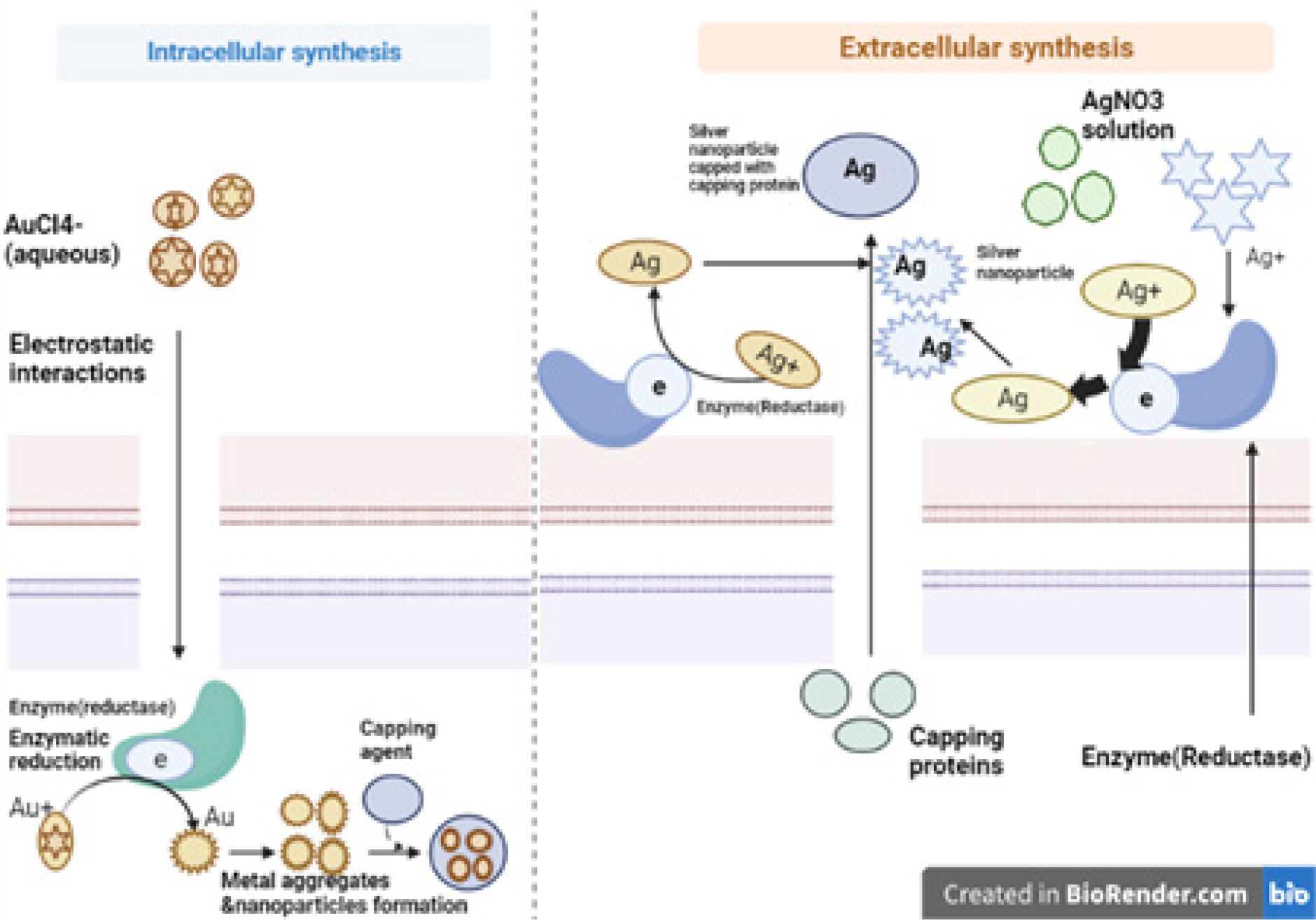
Figure 1:
Mechanism of nanoparticle synthesis by micro-organism.
The production of metal NPs by reductase enzymes, which are either found in the cell wall or released from the cell into the growth medium, occurs during the extracellular biosynthesis of nitrate reductase. The nitrate reductase converts metal ions to their metallic forms during this process.28,29 Additionally, the existence of several components in micro-organisms, such as proteins, enzymes and other biological molecules, is crucial to the process of reducing metal NPs.28 By using NADH-dependent reductases as the electron carrier, the reduction pathways appear to start by transferring an electron from NADH.30 Additionally, micro-organisms can release proteins that serve primarily as a stabilising agent and maintain colloidal stability while inhibiting metal NP clumping.28
BIOSYNTHESIS OF NANOPARTICLES
As indicated in the Figure 2, nanoparticles can be synthesized by various micro-organism.
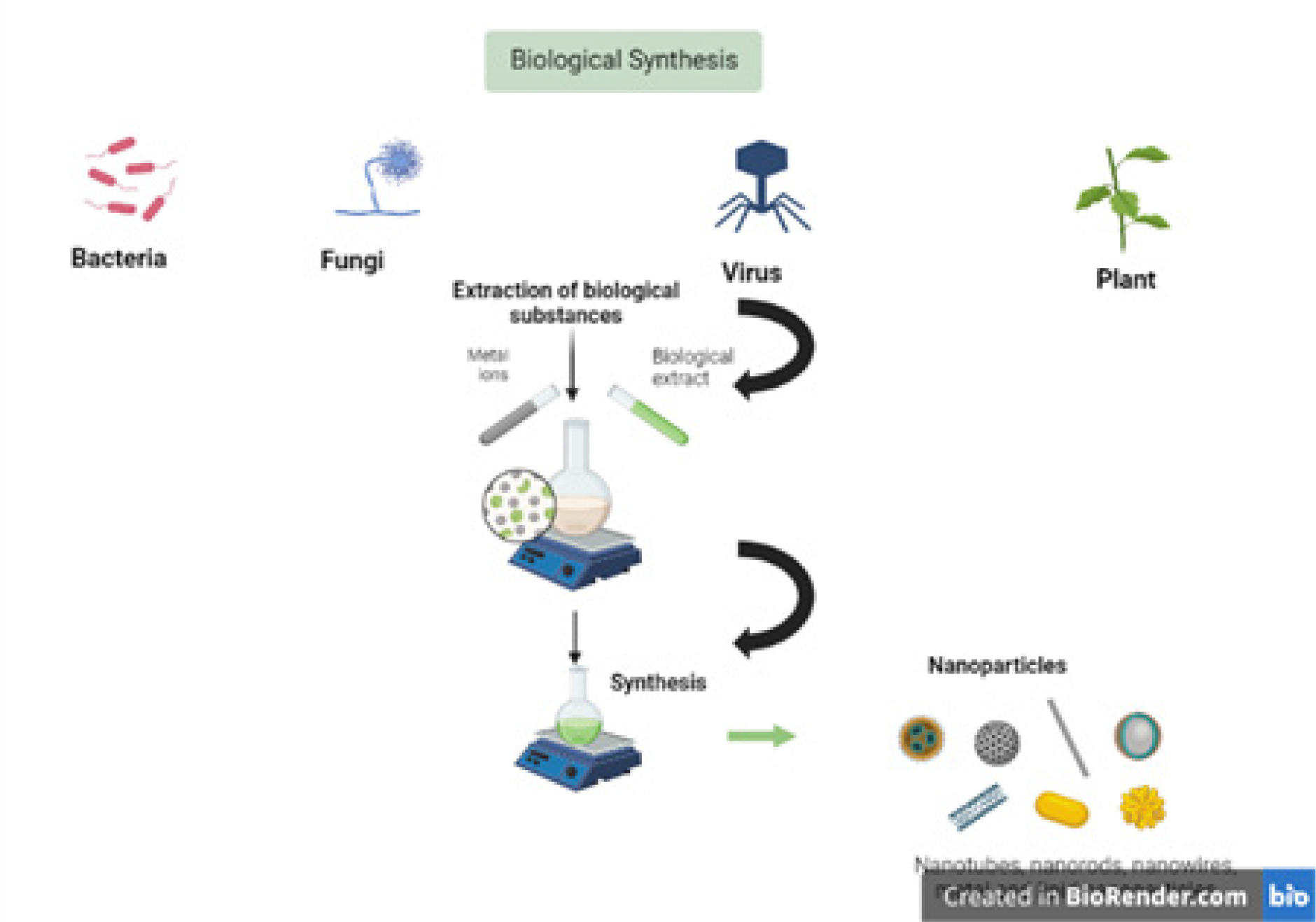
Figure 2:
Biosynthesis of nanoparticles using various micro-organism.
BIOSYNTHESIS OF NANOPARTICLES BY BACTERIA
A bio-nanofactory has been used to create a variety of metal nanoparticles, including Ag, Au, Cu, etc, as well as metal oxide nanoparticles, including Ag2O, CuO, ZnO, TiO2, MnO2, MgO, Fe2O3, etc.31 These NPs have a wide range of applications, such as the development of third generation biosensors,32 the creation of biofilms,33 the labelling and imaging of cell,34 sensoristic devices,35 and diagnostics.36 Additionally, bio fabricated NPs showed promise as anticancer,37–44 antibacterial, antioxidant,40 anticoagulant,42 and antimigration and antiproliferative agents.41 Bacteria are incredibly resilient to abiotic stressors and have the unique capacity to convert heavy metals into NPs. For example, some bacteria, such as Pseudomonas stutzeri (a facultative aerobe) and P. aeruginosa (a facultative anaerobe), can thrive and endure in environments with high quantities of metals, particularly heavy metals.45,46
It is known that bacteria can produce inorganic compounds either inside or outside of their cells. Silver nanoparticles have also been created in the periplasmic region of Bacillus species.47 Silver nanocrystals with a size of 200 nm were generated the bacteria Pseudomonas stutzeri.48,49 The deposition of the particles in vacuole-like granules in the periplasm, likely accompanied by metal efflux and metal binding, was the cause of P. stutzeri’s resistance to silver. The inhabitants of gold mines would be better equipped to withstand the poisonous effects of soluble gold and manufacture gold nanoparticles.50 The colour of the gold nanoparticles (AuNP) containing colloidal solution varied greatly when Acinetobacter sp. SW30 was incubated with different concentration of gold chloride and different cell densities, indicating variation in size and shape. Surprisingly, monodispersed spherical AuNP of size 19 nm was seen at the lowest cell density and HAuCl4 salt concentration, whereas cell number increase led to the development of polyhedral AuNP (39 nm). The extracellular creation of gold nanoparticles in a range of sizes and shapes was also discovered to be mediated by Rhodopseudomonas capsules. The strain was utilised to produce triangular plate (50-400nm) and spherical (10-50nm) AuNPs.51 It has also been shown that ZnO nanoparticle biosynthesis occurs in Lactobacillus plantarum.52 Aeromonas hydrophila, a gram-negative bacterial strain, has been investigated for the production of ZnO nanoparticles with additional antibacterial uses.53 Halomonas elongate was used to create triangular CuO nanoparticles, which demonstrated antimicrobial activity against S. aureus and E.coli.54 Streptomyces sp. (intracellular route) has been used to reduce manganese and zinc nanoparticles quickly and easily. Manganese and zinc NPs ranged in size from 10 to 20 20 nm Table 1.55
Bacteria | Nanoparticles | Size | Applications |
---|---|---|---|
Escherichia coli 56 | Ag | 5-50 nm | Antimicrobial activity |
Mycobacterium sp.57 | Au | 5-55 nm | Anticancer |
Bacillus subtilis 58 | Fe3O4 | 60-80 nm | Antimicrobial activity |
Serratia nematodiphila 59 | Zinc sulphide | 80 +(-)10 nm | Antibacterial |
Lactobacillus sporogenes 60 | ZnO | 145.70 nm | Antimicrobial activity |
Gordonia amicalis 61 | Ag | 5-25 nm | Antioxidant Scavenging activity |
Lactobacillus fermentum 62 | Iron oxide | 10-15 nm | Antimicrobial activity |
Shewanella loihica 63 | Cu | 10-16 nm | Antibacterial activity |
Lactobacillus sp.64 | TiO2 | 50-100 nm | Immobilization or refolding of enzyme |
Brevundimonas diminuta 56 | Ag | 5-50 nm | Antimicrobial activity |
Lactobacillus plantarum 52 | ZnO | 7-19 nm | Treatment of cardiovascular diseases |
Bacillus licheniformis 65 | Cadmium sulphide | 20-40 nm | Antibacterial activity |
Bacillus sp.66 | Selenium nanoparticles | 80-220 nm | Antioxidant and cytotoxic effect |
Paracoccus haeundaensis BC7417167 | Au | 20.93+(-)3.46 nm | Antioxidant activity and antiproliferative effect |
Bacteria use a variety of mechanisms for the synthesis of nanoparticles, including changes in solubility and toxicity caused by reduction or oxidation, the absence of a dedicated metal transport system, biosorption, extracellular complexation or precipitation of metals, bioaccumulation, and efflux system. Bacteria can be used to create nano mineral crystals with properties that are identical to those of chemically produced nano materials. Bacteria can also be used to control the nanoparticles’ different sizes, shapes, and compositions.
BIOSYNTHESIS OF NANOPARTICLES BY FUNGI
Application of myco-nanotechnological methods is a successful biogenic strategy for producing different metal nanoparticles. Nano synthesis can occur either extracellularly or intracellularly just like in bacteria. More proteins are secreted by fungi, which increases the productivity of nanoparticles.68 After being exposed to HAuCl4 solution, the gold nanoparticles were produced intracellularly in the biomass of Verticillium species.69 Another study used Fusarium oxysporum strains to produce extracellular silver metal nanoparticles between 20 and 50 nm.70 Kumar and their groups generated in vitro silver nanoparticles (10-25 nm) stabilized by a capping peptide utilising the nitrate reductase enzyme obtained from Fusarium oxysporum, phytochelatinin, and 4-hydroxyquinoline in the presence of reduced cofactor Nicotinamide Adenine Dinucleotide Phosphate (NADPH).71 The fungi Cladosporium sp. extracted from the medicinal plant Commiphora wightii, were used in Munawer et al 2020.’s report to extracellularly green synthesise spherical gold NPs that were 5-10 nm in size and displayed excellent antiproliferative activity against the breast cancer cell line MCF-7.72 Similar to this, Ramos et al., 2020 described the extracellular manufacture of silver NPs from Trichoderma spp., collected from seeds of plant Bertholletia excelsa (Brazil nut), and came to conclusion that the bio fabricated silver NPs showed greater antibacterial action against Gram-negative bacteria.73
Neethu et al. (2018) demonstrated the environmentally friendly synthesis of silver NPs from the fungus Penicillium polonicum, isolated from the marine green alga Chetomorpha antennina, and came to the conclusion that the biosynthesised silver NPs demonstrated strong antibacterial activity against the biofilm-forming, multidrug-resistant bacterium Acinetobacter baumanii.74 Excellent antibacterial capability was shown by ZnO nanoparticles mediated by Aspergillus niger, and Bismark brown dye deteriorated by up to 90%.76
Aspergillus nidulans has recently been used to create cobalt oxide nanoparticles.76 The switch from bacteria to fungi has the additional benefit of making downstream biomass processing and handling considerably simpler, which can help develop natural nanofactories. Compared to bacteria, fungi are more resourceful in the manufacture of nanoparticles due to their abundance of bioactive metabolites, high aggregation, and enhanced manufacturing Table 2.
Fungus | Nanoparticles | Size | Applications |
---|---|---|---|
Penicillium oxalicum 77 | Ag | 60-80 nm | Antibacterial activity |
Macrophomina phaseolina 78 | Ag | 5-40 nm | Antimicrobial properties |
Cladosporium sp.72 | Au | 5-10 nm | Photodegradation, in vitro anticancer activity and in vivo antitumour studies |
Neurospora crassa 79 | Au | 3-100 nm | Antimicrobial properties |
Aspergillus flavus 80 | TiO2 | 62-74 nm | Antimicrobial activity |
Agaricus bisporus 81 | ZnS | 2.9 nm | Antibacterial activity |
C. geniculatus 82 | ZnO | 2-6 nm | Antibacterial activity |
Coriolus versicolor 83 | CdS | 100-200 nm | Antitumour properties |
Morchella esculenta 84 | Au | 16.51 nm | Antimicrobial activity and cytotoxic activity |
Cladosporium perangustum 85 | Ag | 30-40 nm | Antioxidant, anticancer, and nano-toxicological study |
Trichoderma harzianum 86 | Au | 32-44 nm | Antibacterial activity |
Thermophilic fungus Humicola sp. 87 | CeO2 | 12-20 nm | Antibacterial |
Penicillium chrysogenum 88 | Pt | 5-40 nm | Cytotoxicity |
Penicillium janthinellum DJP06 89 | Au | 1-40 nm | Anticancer activity |
Trichoderma longibrachiatum 90 | Ag | 10 nm | Antifungal against phyto-pathogenic fungi |
Verticillium sp. 91 | Ag | 25 +(-) 12nm | Antimicrobial activity |
BIOSYNTHESIS OF NANOPARTICLES BY ACTINOMYCETES
Important traits of fungi and prokaryotes, such as bacteria, are shared by actinomycetes. Actinomycetes have been found to be crucial in the creation of metal nanoparticles. Due to their synthesis of numerous bioactive components and extracellular enzymes through their saprophytic activity, actinomycetes are regarded as superior groupings among microbial species of commercial value.92 Only a few species from the actinomycetes have been found for the production and characterisation of gold nanoparticles, including Thermomonospora, Nocardia, streptomyces, and Rhodococcus.93 An alkalothermophilic actinomycete called Thermomonospora sp. produced gold ions with a diameter of 8 nm when exposed to extremely alkaline conditions and elevated temperatures. Gold nanoparticles were created using Rhodococcus sp., an actinomycete that can withstand alkaline conditions. These gold nanoparticles, whose sizes range from 5 to 15 nm, were created intracellularly. Actinomycetes produce nanoparticles when metal ions undergo intracellular reduction on the surface of mycelia and cytoplasmic membranes.94 According to some researchers, the electrostatic interactions between Ag+ and negatively charged carboxylate groups in mycelial cell wall enzymes may be the method by which metal nanoparticles are synthesised intracellularly. Enzymes in the cell wall that cause the creation of silver nuclei reduce the silver ions; this causes the nuclei to grow by further decreasing the silver ions and accumulating Ag+ ions.95 The fact that the cells kept growing even after the nanoparticles developed indicates that the metal ions were not hazardous to the cells Table 3.
Actinomycetes | nanoparticles | Size | Application |
---|---|---|---|
Gordonia amarae 96 | Au | 15-40 nm | Application in rapid sensing of copper ions |
Gordonia amicalis 61 | Au | 5-25 nm | Antioxidant scavenging activity |
Streptomyces viridogens HM1097 | Au | 18-20 nm | Antibacterial activity |
Actinomyces sp.95 | Ag | 10-20 nm | Antibacterial activity |
Nocardiopsis sp. MBRC-198 | Ag | 45 nm | Antimicrobial activity |
Marine endophytic actinomycetes99 | Cu | Nano range size | Antibacterial efficacy |
Rhodococcus sp. (Actinomycete)94 | Au | 5-15 nm | Antimicrobial activity |
Streptomyces sp. JAR1100 | Ag | 60-70 nm | Antimicrobial activity |
Streptomyces sp. LK3101 | Ag | 5 nm | Acaricidal activity against Rhipicephalus microplus and Haemaphysalis bispinosa |
Actinomycetes102 | Ag | 5-50 nm | Antibacterial activity |
BIOSYNTHESIS OF NANOPARTICLES BY ALGAE
Algae are also increasingly being used for the production of nanoparticles. Using Cystoseira trinodis, CuO nanoparticles with dimensions of about 7 nm were produced biochemically. They were found to have enhanced antioxidant and antibacterial capabilities as well as methylene blue degradation potential.103 Rapid extracellular production of Au nanoparticles with size range of 8 to 12 nm was seen in the marine alga Sargassum wightii grevillea.104 An additional environmentally acceptable method that recovers Au from diluted hydrometallurgical solutions and leachates of electronic wastes is bio reduction using Fucus vesiculosis. Sargassum crassifolium, a macroalgae and sea grass, has been used in the production of AuNPs. Intriguingly, this study’s UV absorption spectra showed a blue shift as S. crassifolium concentration was increased, which was explained by a reduction in nanoparticle size caused by an increase in nucleation centres in the reductant.105 The creation of unique core (Au)-shell (Ag) nanoparticles has also been studied using Spirulina platensis.106
APPLICATIONS
Nanoparticles are good candidates for a variety of applications in different sectors due to their distinctive size, shape, structure and biological, physical, and chemical properties.
Biomedical
Drug delivery
Nanomaterials are an excellent tool for improving the bioavailability, stability, and bioactivity of pharmaceuticals and can be employed as vehicle for targeted drug administration. Liposomes, nanospheres, polymeric micelles, water-soluble polymers, nano-emulsions, and NP-coated natural antibodies are some of the most common drug delivery nanomaterials.118,119 The use of nanomaterials as medication delivery systems has various benefits over more conventional ones. Targeted drug administration can lower the likelihood of adverse drug reactions in patients. The effectiveness of ZnONPs bio fabricated from pyridinivorans as nanovehicles for anthraquinone was investigated by Kundu.120 ZnoNPs loaded with anthraquinone were found to be cytotoxic to HT-29 colon cancer cells in a concentration-dependent manner, leading to conclusion that they could be used for targeted drug delivery. Fe3O4 (magnetite) and Fe2O3 (maghemite), two types of magnetic nanoparticles, are known to be biocompatible. They have been actively researched for MRI, guided medication delivery, gene therapy, targeted cancer treatment (magnetic hyperthermia), stem cell sorting, and manipulatinves.121 With the addition of its uses as an antibacterial, anti-fungal, antiviral and anti-inflammatory agent, silver nanoparticles have been widely exploited as a novel therapeutics agents. According to Kalishwaralal, Bacillus licheniformis produce silver nanoparticles that may have anti-angiogenic. In addition to more conventional delivery methods, gold nanoparticles have shown promise as a drug and gene delivery scaffold.39 They have special qualities that should makes new delivery methods possible, such as low inherent toxicity, high surface area, stability, and function tunability. Due to their small size, nanoparticle drug carriers are able to pass across the blood-brain barrier and skin’s tight epithelial junctions, which frequently limit the delivery of drugs to their intended target location. Due to their high surface area to volume ratio, nanocarriers display better pharmacokinetics and biodistribution of medical medicines, hence limiting toxicity by preferentially accumulating at the target site.122 It is hoped that targeted drug delivery using nanoparticles will result in a large reduction in the dosage of anticancer medications with improved specificity, increased efficacy, and minimal side effects.
Algae | Nanoparticle | Size | Application |
---|---|---|---|
Nostac linckia 107 | Ag | 5-60 nm | Antibacterial |
Amphiroa rigida 108 | Ag | 25 nm | Antibacterial, cytotoxicity, and larvicidal efficiency |
Galaxaura elongate 109 | Au | 3.85-77 nm | Antibacterial |
Cystoseira baccata110 | Au | 8.4 nm | Anticancer |
Spirulina platensis 111 | Pd | 10-20 nm | Adsorbent |
Sargassum wightii 112 | ZrO2 | 18 nm | Antibacterial |
Chlorella pyrenoidosa 113 | CdSe QD | 4-5 nm | Imatinib sensing |
Neochloris oleoabundans 114 | Ag | 40 nm | Antibacterial |
Leptolyngbya 115 | Ag | 5-50 nm | Antibacterial, anticancer |
Ulva armoricana sp. 116 | Ag | 33 nm | Bactericidal activity |
Chlorella ellipsoidea 117 | Ag | 220.8 +(-) 31.3 nm | Photophysical, catalytic, and antibacterial activity |
Biosensors
Nanoparticles can be employed in biosensor applications and have fascinating electrical and optical features. According to Zheng et al., yeast-produced Au-Ag alloy nanoparticles were used to create a sensitive electrochemical vanillin sensor.123 Electrochemical tests showed that the vanillin sensor based on glassy carbon electrode modified with Au-Ag alloy nanoparticles could increase the electrochemical response of vanillin by at least five times. Vanillin’s oxidation peak current increased linearly with concentration at the sensor under ideal operating circumstances, with a low detection limit of 40 nM. This vanillin sensor was successfully used to measure the amount of vanillin in samples of vanilla bean and vanilla tea, indicating that it might be useful in vanillin monitoring systems.
Agriculture
The use of nanoparticles in agriculture is a new field that has largely remained theoretical. This technology enables the delivery of insecticides encased in nanomaterials for measured and controlled release, the consistency of biopesticides, the slow release of nanomaterial-assisted fertilisers, bioinoculants, and the incorporation of essential micronutrients for organised use in addition to the field applications of agrochemicals.
Nano-fertilizers
A significant problem in the agriculture industry is the steadily declining soil fertility brought on by the excessive use of artificial fertilisers. Research is currently being done to develop nanocomposites that will deliver all the necessary nutrients nano-fertilizers in the right amounts through intelligent delivery systems. With the aforementioned in mind, chemical fertilisers are encapsulated within nanoparticles, which aids in coordinating fertiliser discharge with crop uptake and transportation, preventing unintended nutrient losses. Furthermore, the foliar application of nanoparticles as chemical fertilisers has been linked to a notable increase in yields.124 In addition to this, research has demonstrated that nanosilica particles are able to produce films at the level of the cell wall. These are absorbed by plant roots., which can considerably improve plants’ tolerance to biotic and abiotic challenges and, as a result boost crop yield.
Nano-pesticide
The active ingredients can be successfully shielded from the harsh environment using the nano-encapsulation technique. This strategy also advances persistence, a successful nanotechnology strategy utilised to advance the insecticidal value.
Insecticides, fungicides and nematodes can be nano-encapsulated to create a mixture that will effectively manage pests while preventing or minimising the formation of residues in the soil. NPs can be found in nanop-esticides as micelles, particles, nano-polymers (an organic component), and metal oxides (inorganic constituent). Numerous research has described how metallic NPs can be employed as efficient pesticides against a wide range of insects and pests that cause productivity losses in agriculture around the world.125–128 For instance, Wang showed that a new Oil-Water (O/W) based nano-emulsion may be employed to carry the insecticide Beta-Pypermethrin successfully (beta-PP).129 The outcomes of this study indicate that beta-PP coated on nano-emulsion dispersed more effectively than ordinary beta-PP did, which resulted in a reduction in the total amount of pesticide that was applied. In another study, conducted by Goswami, the researchers discovered that certain Nanoparticles (NPs), such as aluminium oxide, silver, zinc oxide, and titanium oxide NPs, have the ability to prevent silkworms from contracting illnesses caused by baculoviruses such as Bombyx mori nuclear polyhedrosis virus and Sitophilus oryzae.126
Food Industry
Nanoparticle use in the food industry. In the food business, NPs can be applied to two crucial processes: food packaging and food processing.130,131 Nanomaterials are used in packaging materials to stop contamination and maintain food item freshness.132 The capacity of silver NPs to break through the bacterial cells’ protective biofilm gives them tolerance to stress. Silver NPs can therefore be used in the cleaning and disinfection steps of food packaging.133
CONCLUSION
Especially in the fields of electronics, energy, and medical science, nanotechnology is a rapidly evolving and emerging branch of study. Over the past ten years, there have been many significant advancements in the field of micro-organism-produced nanoparticles and their applications. Microorganism-based nanoparticle production is an environmentally beneficial method. Many prokaryotic and eukaryotic groups of micro-organisms have the ability to synthesise nanoparticles, and they have a tightly controlled cellular system. As a result, they could be a useful alternative to common chemical and physical approaches that release numerous hazardous chemicals and radiations into the environment during nanoparticle synthesis. Though, in comparison to physical and chemical methods, the creation of nanoparticles utilising micro-organisms is a somewhat sluggish process but its capacity to control size and form would present a significant advantage over chemical and physical methods. The majority of the bacteria live in environments with a variety of temperature, pH, and pressure, which lends support to the biogenic theory. Due to the bacterial carrier matrix, the particles produced by these procedures have increased specific surface area, and enhanced interaction between the enzyme and the relevant metal salt. The use of biologically synthesised nanoparticles in various fields like agriculture, food industry, biomedical, etc are safe, nontoxic, and environmentally acceptable.
References
- Chopra H, Dey PS, Das D, Bhattacharya T, Shah M, Mubin S, et al. Curcumin nanoparticles as promising therapeutic agents for drug targets. Molecules. 2021;26(16):4998 [PubMed] | [CrossRef] | [Google Scholar]
- Chopra H, Bibi S, Islam F, Ahmad SU, Olawale OA, Alhumaydhi FA, et al. Emerging trends in the delivery of resveratrol by nanostructures: applications of nanotechnology in life sciences. J Nanomater. 2022;2022:1-17. [CrossRef] | [Google Scholar]
- Singla RK, Sai CS, Chopra H, Behzad S, Bansal H, Goyal R, et al. Natural products for the management of castration-resistant prostate cancer: special focus on nanoparticles based studies. Front Cell Dev Biol. 2021;9:745177 [PubMed] | [CrossRef] | [Google Scholar]
- Chopra H, Gandhi S, Gautam RK, Kamal MA. Bacterial nanocellulose based wound dressings: current and future prospects. Curr Pharm Des. 2022;28(7):570-80. [PubMed] | [CrossRef] | [Google Scholar]
- Bhattacharya T, Soares GABE, Chopra H, Rahman MM, Hasan Z, Swain SS, et al. Applications of phyto-nanotechnology for the treatment of neurodegenerative disorders. Materials (Basel). 2022;15(3):804 [PubMed] | [CrossRef] | [Google Scholar]
- Shedbalkar U, Singh R, Wadhwani S, Gaidhani S, Chopade BA. Microbial synthesis of gold nanoparticles: current status and future prospects. Adv Colloid Interface Sci. 2014;209:40-8. [PubMed] | [CrossRef] | [Google Scholar]
- Albanese A, Tang PS, Chan WC. The effect of nanoparticle size, shape, and surface chemistry on biological systems. Annu Rev Biomed Eng. 2012;14:1-16. [PubMed] | [CrossRef] | [Google Scholar]
- Arshad A, Iqbal J, Mansoor Q, Ahmed I. Graphene/SiO2 nanocomposites: the enhancement of photocatalytic and biomedical activity of SiO2 nanoparticles by graphene. J Appl Phys. 2017;121(24):244901 [CrossRef] | [Google Scholar]
- Nath D, Banerjee P. Green nanotechnology–a new hope for medical biology. Environ Toxicol Pharmacol. 2013;36(3):997-1014. [PubMed] | [CrossRef] | [Google Scholar]
- Shafey AME. Green synthesis of metal and metal oxide nanoparticles from plant leaf extracts and their applications: a review. Green Process Synth. 2020;9(1):304-39. [CrossRef] | [Google Scholar]
- Lahiri D, Nag M, Sheikh HI, Sarkar T, Edinur HA, Pati S, et al. Microbiologically-synthesized nanoparticles and their role in silencing the biofilm signaling cascade. Front Microbiol. 2021;12:636588 [PubMed] | [CrossRef] | [Google Scholar]
- Cuong HN, Pansambal S, Ghotekar S, Oza R, Thanh Hai NT, Viet NM, et al. New frontiers in the plant extract mediated biosynthesis of Copper Oxide (CuO) nanoparticles and their potential applications: a review. Environ Res. 2022;203:111858 [PubMed] | [CrossRef] | [Google Scholar]
- [PubMed] | [CrossRef] | [Google Scholar]
- Ettadili FE, Aghris S, Laghrib F, Farahi A, Saqrane S, Bakasse M, et al. Recent advances in the nanoparticles synthesis using plant extract: applications and future recommendations. J Mol Struct. 2022;1248:131538 [CrossRef] | [Google Scholar]
- Lomelí-Rosales DA, Zamudio-Ojeda A, Reyes-Maldonado OK, López-Reyes ME, Basulto-Padilla GC, Lopez-Naranjo EJ, et al. Green synthesis of gold and silver nanoparticles using leaf extract of Capsicum chinense plant. Molecules. 2022;27(5):1692 [PubMed] | [CrossRef] | [Google Scholar]
- Majeed M, Hakeem KR, Rehman RU. Synergistic effect of plant extract coupled silver nanoparticles in various therapeutic applications-present insights and bottlenecks. Chemosphere. 2022;288(2):132527 [PubMed] | [CrossRef] | [Google Scholar]
- Mustapha T, Misni N, Ithnin NR, Daskum AM, Unyah NZ. A review on plants and micro-organisms mediated synthesis of silver nanoparticles, role of plants metabolites and applications. Int J Environ Res Public Health. 2022;19(2):674 [PubMed] | [CrossRef] | [Google Scholar]
- Najafi S, Razavi SM, Khoshkam M, Asadi A. Green synthesized of sulfur nanoparticles and its application on lettuce plants metabolic profiling. BioNanoScience. 2022;12(1):116-27. [CrossRef] | [Google Scholar]
- Kharisov BI, Kharissova OV, Ortiz Méndez U, De La Fuente IG. Decoration of carbon nanotubes with metal nanoparticles: recent trends. Synth React Inorg Metal-Organic Nano-Metal Chem.. 2016;46(1):55-76. [CrossRef] | [Google Scholar]
- Bhattacharya R, Mukherjee P. Biological properties of ”naked” metal nanoparticles. Adv Drug Deliv Rev. 2008;60(11):1289-306. [PubMed] | [CrossRef] | [Google Scholar]
- [PubMed] | [CrossRef] | [Google Scholar]
- Shah M, Fawcett D, Sharma S, Tripathy SK, Poinern GEJ. Green synthesis of metallic nanoparticles via biological entities. Materials (Basel). 2015;8(11):7278-308. [PubMed] | [CrossRef] | [Google Scholar]
- Castro L, Blázquez ML, Muñoz FG, González FG, Ballester A. Mechanism and applications of metal nanoparticles prepared by bio-mediated process. Rev Adv Sci Engng. 2014;3(3):199-216. [CrossRef] | [Google Scholar]
- Fernández-Llamosas H, Castro L, Blázquez ML, Díaz E, Carmona M. Speeding up bioproduction of selenium nanoparticles by using Vibrio natriegens as microbial factory. Sci Rep. 2017;7(1):16046 [PubMed] | [CrossRef] | [Google Scholar]
- Waghmare SR, Mulla MN, Marathe SR, Sonawane KD. Ecofriendly production of silver nanoparticles using Candida utilis and its mechanistic action against pathogenic micro-organisms. 3 Biotech. 2015;5(1):33-8. [PubMed] | [CrossRef] | [Google Scholar]
- Marooufpour N, Alizadeh M, Hatami M, Asgari Lajayer B. Biological synthesis of nanoparticles by different groups of bacteria. In: Microbial Nanobionics. Vol. 1, State-of-the-Art. 2019:63-85. [CrossRef] | [Google Scholar]
- Kalabegishvili TL, Kirkesali EI, Rcheulishvili AN, Ginturi EN, Murusidze IG, Pataraya DT, et al. Synthesis of gold nanoparticles by some strains of . Mater Sci Eng A.. 2012;2(2):164-73. [CrossRef] | [Google Scholar]
- Mohd Yusof H, Mohamad R, Zaidan UH, Abdul Rahman NA. Microbial synthesis of zinc oxide nanoparticles and their potential application as an antimicrobial agent and a feed supplement in animal industry: a review. J Anim Sci Biotechnol.. 2019;10:1-22. [CrossRef] | [Google Scholar]
- Hulkoti NI, Taranath TC. Biosynthesis of nanoparticles using microbes—a review. Colloids Surf B Biointerfaces. 2014;121:474-83. [PubMed] | [CrossRef] | [Google Scholar]
- Jain N, Bhargava A, Majumdar S, Tarafdar JC, Panwar J. Extracellular biosynthesis and characterization of silver nanoparticles using NJP08: a mechanism perspective. Nanoscale. 2011;3(2):635-41. [PubMed] | [CrossRef] | [Google Scholar]
- Grasso G, Zane D, Dragone R. Microbial nanotechnology: challenges and prospects for green biocatalytic synthesis of nanoscale materials for sensoristic and biomedical applications. Nanomaterials (Basel). 2019;10(1):11 [PubMed] | [CrossRef] | [Google Scholar]
- Du L, Jiang H, Liu X, Wang E. Biosynthesis of gold nanoparticles assisted by DH5α and its application on direct electrochemistry of hemoglobin. Electrochem Commun. 2007;9(5):1165-70. [CrossRef] | [Google Scholar]
- Suresh AK, Pelletier DA, Wang W, Broich ML, Moon JW, Gu B, et al. Biofabrication of discrete spherical gold nanoparticles using the metal-reducing bacterium . Acta Biomater. 2011;7(5):2148-52. [PubMed] | [CrossRef] | [Google Scholar]
- Bao H, Lu Z, Cui X, Qiao Y, Guo J, Anderson JM, et al. Extracellular microbial synthesis of biocompatible CdTe quantum dots. Acta Biomater. 2010;6(9):3534-41. [PubMed] | [CrossRef] | [Google Scholar]
- Wang T, Yang L, Zhang B, Liu J. Extracellular biosynthesis and transformation of selenium nanoparticles and application in H2O2 biosensor. Colloids Surf B Biointerfaces. 2010;80(1):94-102. [PubMed] | [CrossRef] | [Google Scholar]
- Ramya S, Shanmugasundaram T, Balagurunathan R. Biomedical potential of actinobacterially synthesized selenium nanoparticles with special reference to anti-biofilm, antioxidant, wound healing, cytotoxic and anti-viral activities. J Trace Elem Med Biol. 2015;32:30-9. [PubMed] | [CrossRef] | [Google Scholar]
- Al-Dhabi NA, Mohammed Ghilan AK, Arasu MV. Characterization of silver nanomaterials derived from marine sp. al-dhabi-87 and its application against multidrug resistant and extended-spectrum beta-lactamase clinical pathogens. Nanomaterials (Basel). 2018;8(5):279 [PubMed] | [CrossRef] | [Google Scholar]
- Roychoudhury P, Gopal PK, Paul S, Pal R. Cyanobacteria assisted biosynthesis of silver nanoparticles—a potential antileukemic agent. J Appl Phycol. 2016;28(6):3387-94. [CrossRef] | [Google Scholar]
- Kalishwaralal K, Banumathi E, Ram Kumar Pandian SRK, Deepak V, Muniyandi J, Eom SH, et al. Silver nanoparticles inhibit VEGF induced cell proliferation and migration in bovine retinal endothelial cells. Colloids Surf B Biointerfaces. 2009;73(1):51-7. [PubMed] | [CrossRef] | [Google Scholar]
- Shao W, Liu H, Liu X, Sun H, Wang S, Zhang R, et al. pH-responsive release behavior and anti-bacterial activity of bacterial cellulose-silver nanocomposites. Int J Biol Macromol. 2015;76:209-17. [PubMed] | [CrossRef] | [Google Scholar]
- Torres SK, Campos VL, León CG, Rodríguez-Llamazares SM, Rojas SM, González M, et al. Biosynthesis of selenium nanoparticles by Pantoea agglomerans and their antioxidant activity. J Nanopart Res. 2012;14(11):1-9. [CrossRef] | [Google Scholar]
- Kalishwaralal K, Deepak V, Ram Kumar Pandian SR, Kottaisamy M, BarathManiKanth S, Kartikeyan B, et al. Biosynthesis of silver and gold nanoparticles using Brevibacterium casei. Colloids Surf B Biointerfaces. 2010;77(2):257-62. [PubMed] | [CrossRef] | [Google Scholar]
- Ahmad MS, Yasser MM, Sholkamy EN, Ali AM, Mehanni MM. Anticancer activity of biostabilized selenium nanorods synthesized by Streptomyces bikiniensis strain Ess_amA-1. Int J Nanomedicine. 2015;10:3389-401. [PubMed] | [CrossRef] | [Google Scholar]
- Gurunathan S, Han JW, Eppakayala V, Kim JH. Green synthesis of graphene and its cytotoxic effects in human breast cancer cells. Int J Nanomedicine. 2013;8:1015-27. [PubMed] | [CrossRef] | [Google Scholar]
- Bridges K, Kidson A, Lowbury EJ, Wilkins MD. Gentamicin- and silver-resistant pseudomonas in a burns unit. Br Med J. 1979;1(6161):446-9. [PubMed] | [CrossRef] | [Google Scholar]
- Haefeli C, Franklin CH, Hardy K. Plasmid-determined silver resistance in Pseudomonas stutzeri isolated from a silver mine. J Bacteriol. 1984;158(1):389-92. [PubMed] | [CrossRef] | [Google Scholar]
- Pugazhenthiran N, Anandan S, Kathiravan G, Udaya Prakash NK, Crawford S, Ashokkumar M, et al. Microbial synthesis of silver nanoparticles by Bacillus sp. J Nanopart Res. 2009;11(7):1811-5. [CrossRef] | [Google Scholar]
- Joerger R, Klaus T, Granqvist CG. Biologically produced silver–carbon composite materials for optically functional thin‐film coatings. Adv Mater. 2000;12(6):407-9. [CrossRef] | [Google Scholar]
- Klaus T, Joerger R, Olsson E, Granqvist CG. Silver-based crystalline nanoparticles, microbially fabricated. Proc Natl Acad Sci U S A. 1999;96(24):13611-4. [PubMed] | [CrossRef] | [Google Scholar]
- Srinath BS, Namratha K, Byrappa K. Eco-friendly synthesis of gold nanoparticles by and their environmental applications. Adv Sci Lett.. 2018;24(8):5942-6. [CrossRef] | [Google Scholar]
- He S, Guo Z, Zhang Y, Zhang S, Wang J, Gu N, et al. Biosynthesis of gold nanoparticles using the bacteria . Mater Lett. 2007;61(18):3984-7. [CrossRef] | [Google Scholar]
- Selvarajan E, Mohanasrinivasan VJ. Biosynthesis and characterization of ZnO nanoparticles using Lactobacillus plantarum VITES07. Mater Lett. 2013;112:180-2. [CrossRef] | [Google Scholar]
- Jayaseelan C, Rahuman AA, Kirthi AV, Marimuthu S, Santhoshkumar T, Bagavan A, et al. Novel microbial route to synthesize ZnO nanoparticles using Aeromonas hydrophila and their activity against pathogenic bacteria and fungi. Spectrochim Acta A Mol Biomol Spectrosc. 2012;90:78-84. [PubMed] | [CrossRef] | [Google Scholar]
- Rad M, Taran M, Alavi M. Effect of incubation time, CuSO4 and glucose concentrations on biosynthesis of Copper Oxide (CuO) nanoparticles with rectangular shape and antibacterial activity: Taguchi method approach. Nano Biomed Eng. 2018;10(1):25-33. [CrossRef] | [Google Scholar]
- Waghmare SS, Deshmukh AM, Kulkarni SW, Oswaldo LA. Biosynthesis and characterization of manganese and zinc nanoparticles. Univers J Environ Res Technol. 2011;1(1) [CrossRef] | [Google Scholar]
- Saeed S, Iqbal A, Ashraf MA. Bacterial-mediated synthesis of silver nanoparticles and their significant effect against pathogens. Environ Sci Pollut Res Int. 2020;27(30):37347-56. [PubMed] | [CrossRef] | [Google Scholar]
- Camas M, Sazak Camas A, Kyeremeh K. Extracellular synthesis and characterization of gold nanoparticles using sp. BRS2A-AR2 isolated from the aerial roots of the Ghanaian mangrove plant, . Indian J Microbiol. 2018;58(2):214-21. [PubMed] | [CrossRef] | [Google Scholar]
- Sundaram PA, Augustine R, Kannan M. Extracellular biosynthesis of iron oxide nanoparticles by strains isolated from rhizosphere soil. Biotechnol Bioprocess Eng. 2012;17(4):835-40. [CrossRef] | [Google Scholar]
- Malarkodi C, Annadurai G. A novel biological approach on extracellular synthesis and characterization of semiconductor zinc sulfide nanoparticles. Appl Nanosci. 2013;3(5):389-95. [CrossRef] | [Google Scholar]
- Mishra M, Paliwal JS, Singh SK, Selvarajan E, Subathradevi C, Mohanasrinivasan V, et al. Studies on the inhibitory activity of biologically synthesized and characterized zinc oxide nanoparticles using lactobacillus sporogens against . J Pure Appl Microbiol. 2013;7(2):1263-8. [CrossRef] | [Google Scholar]
- Sowani H, Mohite P, Munot H, Shouche Y, Bapat T, Kumar AR, et al. Green synthesis of gold and silver nanoparticles by an actinomycete Gordonia amicalis HS-11: mechanistic aspects and biological application. Process Biochem. 2016;51(3):374-83. [CrossRef] | [Google Scholar]
- Park KY, Jeong JK, Lee YE, Daily JW. Health benefits of kimchi (Korean fermented vegetables) as a probiotic food. J Med Food.. 2014;17(1):6-20. [PubMed] | [CrossRef] | [Google Scholar]
- Lv Q, Zhang B, Xing X, Zhao Y, Cai R, Wang W, et al. Biosynthesis of copper nanoparticles using Shewanella loihica PV-4 with antibacterial activity: novel approach and mechanisms investigation. J Hazard Mater. 2018;347:141-9. [PubMed] | [CrossRef] | [Google Scholar]
- Ahmad R, Khatoon N, Sardar M. Biosynthesis, characterization and application of TIO. [PubMed] | [CrossRef] | [Google Scholar]
- Shivashankarappa A, Sanjay KR. Study on biological synthesis of cadmium sulfide nanoparticles by Bacillus licheniformis and its antimicrobial properties against food borne pathogens. Nanosci Nanotechnol Res. 2015;3(1):6-15. [PubMed] | [CrossRef] | [Google Scholar]
- Forootanfar H, Adeli-Sardou M, Nikkhoo M, Mehrabani M, Amir-Heidari B, Shahverdi AR, et al. Antioxidant and cytotoxic effect of biologically synthesized selenium nanoparticles in comparison to selenium dioxide. J Trace Elem Med Biol. 2014;28(1):75-9. [PubMed] | [CrossRef] | [Google Scholar]
- Patil MP, Kang MJ, Niyonizigiye I, Singh A, Kim JO, Seo YB, et al. Extracellular synthesis of gold nanoparticles using the marine bacterium Paracoccus haeundaensis BC74171T and evaluation of their antioxidant activity and antiproliferative effect on normal and cancer cell lines. Colloids Surf B Biointerfaces. 2019;183:110455 [PubMed] | [CrossRef] | [Google Scholar]
- Mohanpuria P, Rana NK, Yadav SK. Biosynthesis of nanoparticles: technological concepts and future applications. J Nanopart Res. 2008;10(3):507-17. [CrossRef] | [Google Scholar]
- Mukherjee P, Ahmad A, Mandal D, Senapati S, Sainkar SR, Khan MI, et al. Bioreduction of AuCl4− Ions by the Fungus, sp. and Surface Trapping of the Gold Nanoparticles Formed D.M. and S.S. thank the Council of Scientific and Industrial Research (CSIR), Government of India, for financial assistance. Angew Chem Int Ed. 2001;40(19):3585 [CrossRef] | [Google Scholar]
- Ahmad A, Mukherjee P, Senapati S, Mandal D, Khan MI, Kumar R, et al. Extracellular biosynthesis of silver nanoparticles using the fungus . Colloids Surf B Biointerfaces. 2003;28(4):313-8. [CrossRef] | [Google Scholar]
- Anil Kumar S, Abyaneh MK, Gosavi SW, Kulkarni SK, Pasricha R, Ahmad A, et al. Nitrate reductase-mediated synthesis of silver nanoparticles from AgNO 3. Biotechnol Lett. 2007;29(3):439-45. [PubMed] | [CrossRef] | [Google Scholar]
- Munawer U, Raghavendra VB, Ningaraju S, Krishna KL, Ghosh AR, Melappa G, et al. Biofabrication of gold nanoparticles mediated by the endophytic Cladosporium species: photodegradation, anticancer activity and antitumor studies. Int J Pharm. 2020;588:119729 [PubMed] | [CrossRef] | [Google Scholar]
- Ramos MM, Morais dos S E, da S, Sena I, Lima AL, de Oliveira FR, de Freitas CM, et al. Silver nanoparticle from whole cells of the fungi spp. isolated from Brazilian Amazon. Biotechnology letters. 2020;42:833-43. [PubMed] | [CrossRef] | [Google Scholar]
- Neethu S, Midhun SJ, Sunil MA, Soumya S, Radhakrishnan EK, Jyothis M, et al. Efficient visible light induced synthesis of silver nanoparticles by ARA 10 isolated from Chetomorpha antennina and its antibacterial efficacy against serovar Typhimurium. J Photochem Photobiol B.. 2018;180:175-85. [PubMed] | [CrossRef] | [Google Scholar]
- Kalpana VN, Kataru BAS, Sravani N, Vigneshwari T, Panneerselvam A, Devi Rajeswari VD, et al. Biosynthesis of zinc oxide nanoparticles using culture filtrates of : antimicrobial textiles and dye degradation studies. OpenNano. 2018;3:48-55. [CrossRef] | [Google Scholar]
- Vijayanandan AS, Balakrishnan RM. Biosynthesis of cobalt oxide nanoparticles using endophytic fungus . J Environ Manage. 2018;218:442-50. [PubMed] | [CrossRef] | [Google Scholar]
- Feroze N, Arshad B, Younas M, Afridi MI, Saqib S, Ayaz A, et al. Fungal mediated synthesis of silver nanoparticles and evaluation of antibacterial activity. Microsc Res Tech. 2020;83(1):72-80. [PubMed] | [CrossRef] | [Google Scholar]
- Chowdhury S, Basu A, Kundu S. Green synthesis of protein capped silver nanoparticles from phytopathogenic fungus (Tassi) Goid with antimicrobial properties against multidrug-resistant bacteria. Nanoscale Res Lett. 2014;9(1):365 [PubMed] | [CrossRef] | [Google Scholar]
- Castro-Longoria E, Vilchis-Nestor AR, Avalos-Borja M. Biosynthesis of silver, gold and bimetallic nanoparticles using the filamentous fungus . Colloids Surf B Biointerfaces. 2011;83(1):42-8. [PubMed] | [CrossRef] | [Google Scholar]
- Rajakumar G, Rahuman AA, Roopan SM, Khanna VG, Elango G, Kamaraj C, et al. Fungus-mediated biosynthesis and characterization of TiO2 nanoparticles and their activity against pathogenic bacteria. Spectrochim Acta A Mol Biomol Spectrosc. 2012;91:23-9. [PubMed] | [CrossRef] | [Google Scholar]
- Senapati US, Jha DK, Sarkar D. Structural, optical, thermal and electrical properties of fungus guided biosynthesized zinc sulphide nanoparticles. Res J Chem Sci.. 2015;2231:606X [PubMed] | [CrossRef] | [Google Scholar]
- Kadam VV, Ettiyappan JP, Mohan Balakrishnan RM. Mechanistic insight into the endophytic fungus mediated synthesis of protein capped ZnO nanoparticles. Mater Sci Eng B.. 2019;243:214-21. [CrossRef] | [Google Scholar]
- Sanghi R, Verma P. A facile green extracellular biosynthesis of CdS nanoparticles by immobilized fungus. Chem Eng J. 2009;155(3):886-91. [CrossRef] | [Google Scholar]
- Acay H. Utilization of Morchella esculenta-mediated green synthesis golden nanoparticles in biomedicine applications. Prep Biochem Biotechnol. 2021;51(2):127-36. [PubMed] | [CrossRef] | [Google Scholar]
- Govindappa M, Lavanya M, Aishwarya P, Pai K, Lunked P, Hemashekhar B, et al. Synthesis and characterization of endophytic fungi, Cladosporium perangustum mediated silver nanoparticles and their antioxidant, anticancer and nano-toxicological study. BioNanoScience. 2020;10(4):928-41. [CrossRef] | [Google Scholar]
- Tripathi RM, Shrivastav BR, Shrivastav A. Antibacterial and catalytic activity of biogenic gold nanoparticles synthesised by . IET Nanobiotechnology. 2018;12(4):509-13. [PubMed] | [CrossRef] | [Google Scholar]
- Khan SA, Ahmad A. Fungus mediated synthesis of biomedically important cerium oxide nanoparticles. Mater Res Bull. 2013;48(10):4134-8. [CrossRef] | [Google Scholar]
- Subramaniyan SA, Sheet S, Vinothkannan M, Yoo DJ, Lee YS, Belal SA, et al. One-pot facile synthesis of Pt nanoparticles using cultural filtrate of microgravity simulated grown and their activity on bacteria and cancer cells. J Nanosci Nanotechnol. 2018;18(5):3110-25. [PubMed] | [CrossRef] | [Google Scholar]
- Pareek V, Bhargava A, Panwar J. Biomimetic approach for multifarious synthesis of nanoparticles using metal tolerant fungi: a mechanistic perspective. Mater Sci Eng B. 2020;262:114771 [CrossRef] | [Google Scholar]
- Elamawi RM, Al-Harbi RE, Hendi AA. Biosynthesis and characterization of silver nanoparticles using Trichoderma longibrachiatum and their effect on phytopathogenic fungi. Egypt J Biol Pest Control. 2018;28(1):1 [CrossRef] | [Google Scholar]
- Mukherjee P, Ahmad A, Mandal D, Senapati S, Sainkar SR, Khan MI, et al. Fungus-mediated synthesis of silver nanoparticles and their immobilization in the mycelial matrix: a novel biological approach to nanoparticle synthesis. Nano Lett. 2001;1(10):515-9. [CrossRef] | [Google Scholar]
- Kumar SA, Peter YA, Nadeau JL. Facile biosynthesis, separation and conjugation of gold nanoparticles to doxorubicin. Nanotechnology. 2008;19(49):495101 [PubMed] | [CrossRef] | [Google Scholar]
- El-Batal AI, Mona S, Al-Tamie M. Biosynthesis of gold nanoparticles using marine and their antimicrobial, antioxidant and antitumor () activities. J Chem Pharm Res.. 2015;7(7):1020-36. [PubMed] | [CrossRef] | [Google Scholar]
- Ahmad A, Senapati S, Khan MI, Kumar R, Ramani R, Srinivas V, et al. Intracellular synthesis of gold nanoparticles by a novel alkalotolerant actinomycete, . Nanotechnology. 2003;14(7):824-8. [CrossRef] | [Google Scholar]
- Abdeen S, Geo S, Praseetha PK, Dhanya RP. Biosynthesis of silver nanoparticles from Actinomycetes for therapeutic applications. [CrossRef] | [Google Scholar]
- Bennur T, Khan Z, Kshirsagar R, Javdekar V, Zinjarde S. Biogenic gold nanoparticles from the Actinomycete Gordonia amarae: application in rapid sensing of copper ions. Sens Actuators B. 2016;233:684-90. [CrossRef] | [Google Scholar]
- Balagurunathan R, Radhakrishnan M, Rajendran RB, Velmurugan D. Biosynthesis of gold nanoparticles by actinomycete Streptomyces viridogens strain. ;HM10 [CrossRef] | [Google Scholar]
- Manivasagan P, Venkatesan J, Senthilkumar K, Sivakumar K, Kim SK. Biosynthesis, antimicrobial and cytotoxic effect of silver nanoparticles using a novel Nocardiopsis sp. MBRC-1. BioMed Res Int. 2013;2013:287638 [PubMed] | [CrossRef] | [Google Scholar]
- Rasool U, Hemalatha SJ. Marine endophytic actinomycetes assisted synthesis of Copper Nanoparticles (CuNPs): characterization and antibacterial efficacy against human pathogens. Mater Lett. 2017;194:176-80. [CrossRef] | [Google Scholar]
- Chauhan R, Kumar A, Abraham J. A biological approach to the synthesis of silver nanoparticles with Streptomyces sp JAR1 and its antimicrobial activity. Sci Pharm. 2013;81(2):607-21. [PubMed] | [CrossRef] | [Google Scholar]
- Karthik L, Kumar G, Kirthi AV, Rahuman AA, Bhaskara Rao KV. Streptomyces sp. LK3 mediated synthesis of silver nanoparticles and its biomedical application. Bioprocess Biosyst Eng. 2014;37(2):261-7. [PubMed] | [CrossRef] | [Google Scholar]
- Narasimha G, Alzohairy M, Khadri H, Mallikarjuna K. Extracellular synthesis, characterization and antibacterial activity of silver nanoparticles by Actinomycetes isolative. [PubMed] | [CrossRef] | [Google Scholar]
- Gu H, Chen X, Chen F, Zhou X, Parsaee Z. Ultrasound-assisted biosynthesis of CuO-NPs using brown alga : characterization, photocatalytic AOP, DPPH scavenging and antibacterial investigations. Ultrason Sonochem. 2018;41:109-19. [PubMed] | [CrossRef] | [Google Scholar]
- Singaravelu G, Arockiamary JS, Kumar VG, Govindaraju K. A novel extracellular synthesis of monodisperse gold nanoparticles using marine alga, Greville. Colloids Surf B Biointerfaces. 2007;57(1):97-101. [PubMed] | [CrossRef] | [Google Scholar]
- [PubMed] | [CrossRef] | [Google Scholar]
- Govindaraju K, Basha SK, Kumar VG, Singaravelu G. Silver, gold and bimetallic nanoparticles production using single-cell protein () Geitler. J Mater Sci.. 2008;43(15):5115-22. [CrossRef] | [Google Scholar]
- Vanlalveni C, Rajkumari K, Biswas A, Adhikari PP, Lalfakzuala R, Rokhum L, et al. Green synthesis of silver nanoparticles using Nostoc linckia and its antimicrobial activity: a novel biological approach. BioNanoScience. 2018;8(2):624-31. [CrossRef] | [Google Scholar]
- Gopu M, Kumar P, Selvankumar T, Senthilkumar B, Sudhakar C, Govarthanan M, et al. Green biomimetic silver nanoparticles utilizing the red algae and its potent antibacterial, cytotoxicity and larvicidal efficiency. Bioprocess Biosyst Eng. 2021;44(2):217-23. [PubMed] | [CrossRef] | [Google Scholar]
- Abdel-Raouf N, Al-Enazi NM, Ibraheem IBM. Green biosynthesis of gold nanoparticles using and characterization of their antibacterial activity. Arab J Chem.. 2017;10:S3029-39. [CrossRef] | [Google Scholar]
- González-Ballesteros N, Prado-López S, Rodríguez-González JB, Lastra M, Rodríguez-Argüelles MC. Green synthesis of gold nanoparticles using brown algae : its activity in colon cancer cells. Colloids Surf B Biointerfaces. 2017;153:190-8. [PubMed] | [CrossRef] | [Google Scholar]
- Sayadi MH, Salmani N, Heidari A, Rezaei MR. Bio-Synthesis of palladium nanoparticle using alga extract and its application as adsorbent. Surf Interfaces. 2018;10:136-43. [CrossRef] | [Google Scholar]
- Kumaresan M, Vijai Anand K, Govindaraju K, Tamilselvan S, Ganesh Kumar V. Seaweed Sargassum wightii mediated preparation of zirconia (ZrO2) nanoparticles and their antibacterial activity against gram positive and gram negative bacteria. Microb Pathog. 2018;124:311-5. [PubMed] | [CrossRef] | [Google Scholar]
- Zhang Z, Chen J, Yang Q, Lan K, Yan Z, Chen J, et al. Eco-friendly intracellular microalgae synthesis of fluorescent CdSe QDs as a sensitive nanoprobe for determination of imatinib. Sens Actuators B. 2018;263:625-33. [CrossRef] | [Google Scholar]
- Bao Z, Lan CQ. Mechanism of light-dependent biosynthesis of silver nanoparticles mediated by cell extract of Neochloris oleoabundans. Colloids Surf B Biointerfaces. 2018;170:251-7. [PubMed] | [CrossRef] | [Google Scholar]
- Zada S, Ahmad A, Khan S, Yu X, Chang K, Iqbal A, et al. Biogenic synthesis of silver nanoparticles using extracts of Leptolyngbya JSC-1 that induce apoptosis in HeLa cell line and exterminate pathogenic bacteria. Artif Cells Nanomed Biotechnol. 2018;46(S3):471-80. [PubMed] | [CrossRef] | [Google Scholar]
- Massironi A, Morelli A, Grassi L, Puppi D, Braccini S, Maisetta G, et al. Ulvan as novel reducing and stabilizing agent from renewable algal biomass: application to green synthesis of silver nanoparticles. Carbohydr Polym. 2019;203:310-21. [PubMed] | [CrossRef] | [Google Scholar]
- Borah D, Das N, Das N, Bhattacharjee A, Sarmah P, Ghosh K, et al. Alga‐mediated facile green synthesis of silver nanoparticles: photophysical, catalytic and antibacterial activity. Appl Organomet Chem. 2020;34(5):e5597 [CrossRef] | [Google Scholar]
- [CrossRef] | [Google Scholar]
- Omlor AJ, Nguyen J, Bals R, Dinh QT. Nanotechnology in respiratory medicine. Respir Res. 2015;16(1):64 [PubMed] | [CrossRef] | [Google Scholar]
- Kundu D, Hazra C, Chatterjee A, Chaudhari A, Mishra S. Extracellular biosynthesis of zinc oxide nanoparticles using NT2: multifunctional textile finishing, biosafety evaluation and drug delivery in colon carcinoma. J Photochem Photobiol B. 2014;140:194-204. [PubMed] | [CrossRef] | [Google Scholar]
- Fan TX, Chow SK, Zhang D. Biomorphic mineralization: from biology to materials. Prog Mater Sci. 2009;54(5):542-659. [CrossRef] | [Google Scholar]
- Vaidyanathan R, Kalishwaralal K, Gopalram S, Gurunathan S. Nanosilver-The burgeoning therapeutic molecule and its green synthesis (Retraction of vol 27, pg 924, 2009). Biotechnol Adv.. 2010;28(6):940 [PubMed] | [CrossRef] | [Google Scholar]
- Zheng D, Hu C, Gan T, Dang X, Hu S. Preparation and application of a novel vanillin sensor based on biosynthesis of Au–Ag alloy nanoparticles. Sens Actuators B. 2010;148(1):247-52. [CrossRef] | [Google Scholar]
- Tarafdar JC. Perspectives of nanotechnological applications for crop production. NAAS News. 2012;12:8-11. [CrossRef] | [Google Scholar]
- Rai M, Ingle A. Role of nanotechnology in agriculture with special reference to management of insect pests. Appl Microbiol Biotechnol. 2012;94(2):287-93. [PubMed] | [CrossRef] | [Google Scholar]
- Goswami A, Roy I, Sengupta S, Debnath N. Novel applications of solid and liquid formulations of nanoparticles against insect pests and pathogens. Thin Solid Films. 2010;519(3):1252-7. [CrossRef] | [Google Scholar]
- Athanassiou CG, Kavallieratos NG, Benelli G, Losic D, Usha Rani P, Desneux N, et al. Nanoparticles for pest control: current status and future perspectives. J Pest Sci. 2018;91(1):1-15. [CrossRef] | [Google Scholar]
- Rawtani D, Khatri N, Tyagi S, Pandey G. Nanotechnology-based recent approaches for sensing and remediation of pesticides. J Environ Manage. 2018;206:749-62. [PubMed] | [CrossRef] | [Google Scholar]
- Wang L, Li X, Zhang G, Dong J, Eastoe J. Oil-in-water nanoemulsions for pesticide formulations. J Colloid Interface Sci. 2007;314(1):230-5. [PubMed] | [CrossRef] | [Google Scholar]
- Shukla K. Nanotechnology and emerging trends in dairy foods: the inside story to food additives and ingredients. Int J Nanosci Nanotechnol. 2012;1(1):41-58. [PubMed] | [CrossRef] | [Google Scholar]
- Espitia PJP, Soares NdFF, Coimbra JS, de Andrade NJ, Cruz RS, Medeiros EAA, et al. Zinc oxide nanoparticles: synthesis, antimicrobial activity and food packaging applications. Food Bioprocess Technol. 2012;5(5):1447-64. [CrossRef] | [Google Scholar]
- Salem SS, Fouda A. Green synthesis of metallic nanoparticles and their prospective biotechnological applications: an overview. Biol Trace Elem Res. 2021;199(1):344-70. [PubMed] | [CrossRef] | [Google Scholar]
- Huang J, Lin L, Sun D, Chen H, Yang D, Li Q, et al. Bio-inspired synthesis of metal nanomaterials and applications. Chem Soc Rev. 2015;44(17):6330-74. [PubMed] | [CrossRef] | [Google Scholar]