ABSTRACT
The overwhelming demand for plastics results in overproduction of plastics especially single-use or non-biodegradable plastics. Consequently, this has given rise to environmental concerns due to the addition of plastic waste in the environment where they may harm human, animals, and ecosystem. Due to this, the adoption of alternative sustainable packaging materials has gained more attention among the researchers and industries in developing biodegradable films in order to reduce plastic pollution. Chitosan is utilized for the formation of biodegradable films. Chitosan that is derived from chitin, is the second most abundant biopolymer after cellulose. It is known as a versatile polymer for many applications due to its excellent properties such as biodegradability, non-toxicity, antibacterial effects, and hydrophilicity. However, inherent drawbacks such as high sensitivity to humidity and low mechanical properties become the major limitation to its industrial application, particularly in food packaging applications. In this study, extensive review of the scientific literature addressing chitosan-based films, their properties, and strategies to enhance its physicochemical properties was conducted. The objective of this study is to provide a comprehensive literature review of the last 10 years (2020-2021) addressing chitosan-based films and strategies implemented for their performance to improve food packaging applications.
INTRODUCTION
Each year, more than 350 million tons of plastics are manufactured and approximately 8 million tons of plastic litter end up in the world’s oceans.1,2 According to Marcus Eriksen and his colleagues, there was more than 5 trillion plastic debris, weighing more than 260,000 tons in the ocean surface due to plastic pollution in 2014.3 Marine animals are greatly affected by plastic pollution as they can ingest or become entangled within the plastic debris in the ocean which ultimately can lead to injury and death. Plastic pollution does not only affect marine life, it also threatens human health, quality and safety of food.4 Therefore, various measures are taken to resolve this issue, including the development of biodegradable plastics. One of the largest applications for plastics are packaging, particularly food packaging which are widely used to preserve and protect foods in order to bring satisfaction to consumer desires and industry demands specially to improve food safety and food quality with optimal cost.5,6 Nevertheless, food packaging industries are now pushed towards the development and manufacture of biodegradable plastic films made from eco-friendly materials due to uncontrollable amounts of plastic pollution in the environment, high cost of petroleum and fossil reserves depletion.7,8 Eco-friendly materials such as bioplastics can be defined as biodegradable plastics or plastic that can be obtained from biobased materials (renewable resources).
Biodegradable natural polymers have been investigated extensively as alternatives to synthetic petroleum-based polymers due to unique advantages compared to conventional non-biodegradable plastics, reducing the dependence on fossil resource, mitigating greenhouse emissions and carbon footprint.7,9 Polysaccharides, lipid, proteins, and their derivatives are the basic material to form biodegradable plastics. Among polysaccharides, chitosan has gained attention from industry for its applications in food packaging due to its unique properties such as biodegradability, biocompatibility, non-toxicity, good film-forming properties, antimicrobial properties, antioxidant properties, high reactivity and chemical stability.10–12 Chitosan is a versatile biopolymer obtained from the skeleton of shellfish which is used in many industrial applications and in food packaging.13 However, inherent drawbacks of chitosan which are causing restrictions for its industrial applications include its low thermal and mechanical stability as well as sensitivity to humidity.14 Therefore, blends chitosan with other biopolymers could overcome these drawbacks. Kiing Sie Cheong et al. developed biodegradable plastics by using sago starch as a biopolymer and the bioplastics formed possess good compatibility and miscibility.15 Another study by Shirai et al. demonstrated the production of biodegradable films by using starch and poly (lactic acid).16 Biodegradable food packaging has been synthesized through the blending of mahua oil-based polyurethane and chitosan.17 Bioplastics made from blending chitosan and yellow pumpkin starch have been synthesized successfully in which the film took 30 days to totally biodegrade.18 The objective of the present paper is to provide a comprehensive literature review of the last 10 years addressing chitosan-based films and strategies implemented for their potential to improve food packaging applications.
MATERIALS AND METHODS
This review article was based upon searches of the National Center for Biotechnology (NCBI), MEDLINE, Google Scholar, and Science Direct databases. The search keywords to select relevant studies were biodegradable film sheet and chitosan, chitosan and food packaging, and plastic pollution. In particular, we reviewed fully documented articles in English only which were published in the year between 2010 to 2021. Non-English papers and articles with irrelevant title were excluded. Those that were published before 2010 were also excluded. Figure 1 summarizes the process of article selection.
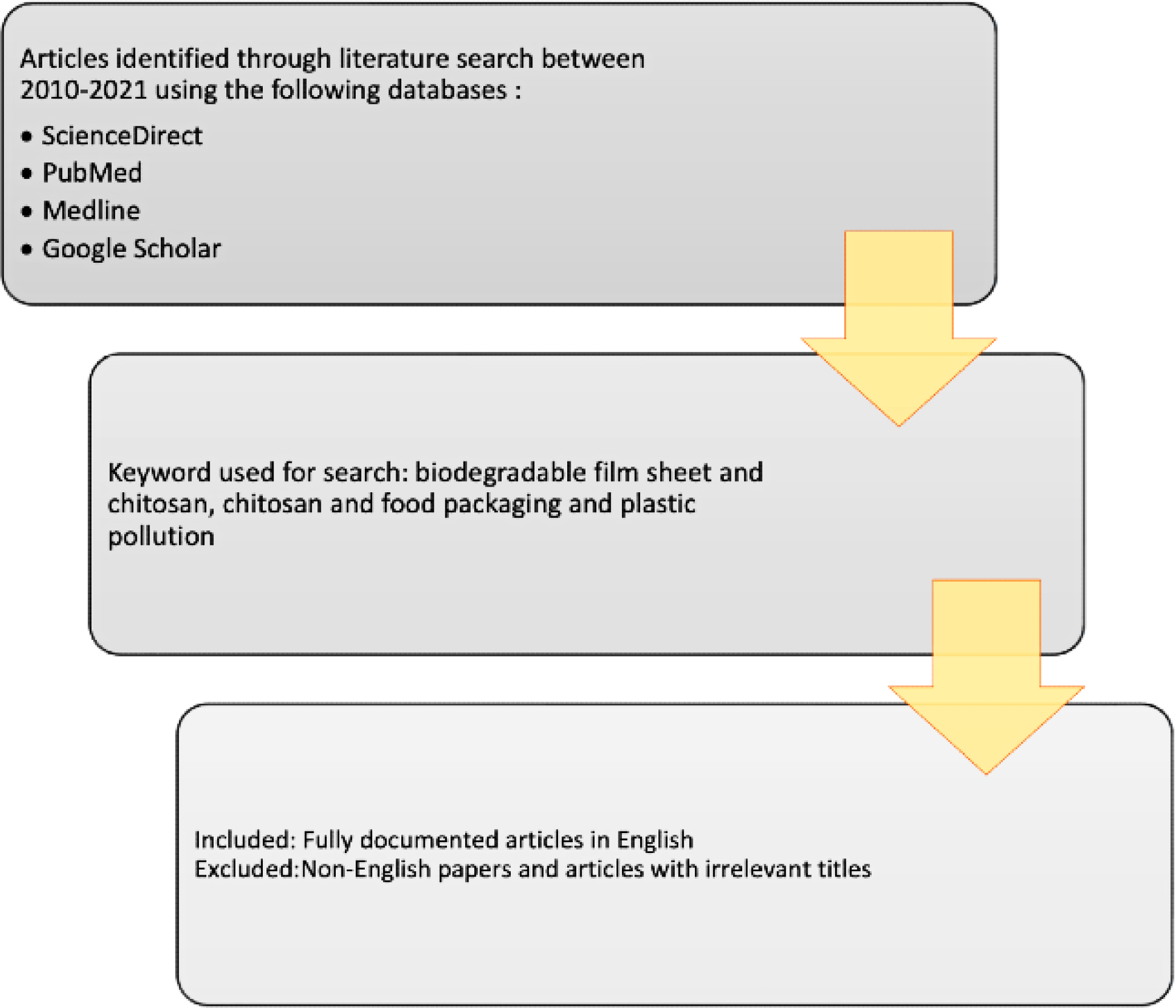
Figure 1.
Flow chart of article selection process.
FEATURES AND POTENTIAL OF CHITOSAN
Chitosan is one of the most abundant natural polymers. It is a de-N-acetylated form of chitin that can be obtained from the shells of shrimps or crabs. Chitin can also be acquired from terrestrial arthropods (spiders, scorpions, cockroaches and beetles), micro-organisms, and Mollusca such as squid.19 Chitosan has been widely utilized in various applications especially in food packaging due to its properties such as it being natural and biodegradable.20 In addition, chitosan used in the food industry can act as an antimicrobial agent, used as a thickener or emulsifying agent, and integrator. It can also be applied to form an edible film and coating for foods.19,21,22 A recent study has emphasized the mechanical, thermal and permeable properties of films governed by the molecular weight of chitosan.23 Furthermore, a study reported that chitosan can be degraded in soil without causing soil pollution. Additionally, chitosan films have also shown a high percentage of weight loss and the polymer network of the film can be easily penetrated by soil moisture, making it susceptible to hydrolysis by microorganisms in soil.17
Mechanical Properties of Chitosan
Tensile strength, elongation at break, and young’s modulus or elastic modulus are the parameters in evaluating the mechanical properties of chitosan based films. A linear relationship is observed between tensile strength and the molecular weight of the films as well as the degree of deacetylation of chitosan. Plus, longer storage of chitosan with acetic acid films at room temperature has enhanced the tensile strength of the films due to decreased of the free volume of the polymer and the time- dependent conformational changes of chitosan molecules.24,25 Furthermore, the type and nature of acids incorporated in chitosan films will significantly affect their mechanical strength. It has been described that chitosan films produced using acetic acid were observed to exhibit a higher tensile strength compared to films produced using citric, lactic or malic acid solutions. The use of glycerol as a plasticizer can also enhance the mechanical properties of chitosan films.24,26,27 A study by Vlacha et al.28 compared the efficiency of plasticization of glycerol with oleic acid that had been incorporated in chitosan/clay nanocomposites as a plasticizer. It was found that glycerol acted as better plasticizer than oleic acid in terms of mechanical strength. It has also been reported that the addition of small quantities of ethanol results in enhanced plasticization of chitosan/oil film with an increased in strain at break as ethanol allows a direct interaction to occur between chitosan and olive and corn oil.29 Mohd Isa and Mohamed,30 reported that low concentrations of chitosan exhibited higher tensile strength while chitosan of more than 5% concentration had lower tensile strength in chitosan/ polyethylene-based films. This occurred as there are reduced interactions between chitosan polymer chains and polyethylene which result in a less compact network. Similarly, the elongation at break was observed to be highest in 2% chitosan while 5% and 10% chitosan in chitosan/polyethylene-based films were found to be very brittle. On the contrary, the Young’s modulus was highest in 10% chitosan and lowest in 2% chitosan.
Antimicrobial Properties of Chitosan
Antimicrobial activity of chitosan against Gram-positive and Gram-negative bacteria as well as fungi has drawn attention for potential use as a natural preservative for food.31 Several factors affect the antimicrobial properties of chitosan including the pH of the media, type of pathogen, concentration, and molecular weight of chitosan.32,33 The state of chitosan (film or solution), degree of acetylation, and molecular mass are also found to affect the antimicrobial activity of chitosan.34 Chitosan has been reported to be effective against molds and yeast, such as Candida lambica, Fusarium oxysporum, Rhizoctonia solani through its fungistatic effect. Chitosan can also act as an antifungal agent by causing spore germination and tube elongation.35 The mechanism of chitosan’s = antimicrobial activity is due to the interaction between microbial negative cell membrane constituents (e.g. proteins, amino acids, phosphoryl groups of phospholipid) and NH3+ of glucosamine in the backbone of chitosan. Consequently, this interaction will interfere with the energy metabolism, membrane permeability and integrity, nutrient transport of the microorganisms which can lead to cell death.14,36 Other studies suggest that the chelating activity of chitosan allows it to bind to essential trace metals and spores, preventing toxin production and inhibits microbial growth.33,37
As cited by Leceta et al.,38 chitosan- based coatings delayed the spoilage of baby carrots and maintained the quality of the products. Meanwhile, blended films of chitosan and brewer’s spent grain protein showed bacterial inhibition against Escherichia coli, Staphylococcus aureus, Salmonella typhimurium, and Listeria monocytogenes.39 The incorporation of red grape seed extract and Zizipohora clinopodioides essential oil into chitosan/gelatin- based films enhanced the antibacterial activity against Pseudomonas spp. Pseudomonas fluorescens, Listeria monocytogenes, Shewanella putrafaciens, Enterobacteriaceae species, and lactic acid bacteria which improved the shelf life of minced trout fillet.40 Caro et al.34 reported that chitosan/ tripolyphosphate nanoparticles with thymol films showed a potent antimicrobial effect against Salmonella typhimurium, Escherichia coli, and Enterobacter aerogenes. The films also exhibited good antimicrobial activity against Pseudomonas aeruginosa, Listeria innocua, and Staphylococcus aureus. Another study outlined the linear relationship between chitosan concentration and antimicrobial activity in which 100/0 wt% of chitosan/TEMPO-cellulose nanofibers films exhibited complete inhibition against all Salmonella enterica, Escherichia coli and Listeria monocytogenes compared to that of films with 85/15 wt% and 75/25 wt% chitosan/TEMPO-cellulose nanofibers.41
Antioxidant Properties of Chitosan
Antioxidant properties are one of the major properties of active packaging, which is important to extend the shelf life of food products. Chitosan has been reported to possess antioxidant properties. Chitosan gains its scavenging ability as its monomers can maintain two hydroxyl group and one amino which react with free radicals.42,43 A study reported that molecular weight of chitosan will affect its antioxidant activity. High molecular weight chitosan was found to be non-effective against superoxide and hydroxyl radicals compared to that of low molecular weight chitosan because shorter chains of low molecular weight chitosan are less likely to develop intramolecular hydroxyl bonds and therefore, more activated amino and hydroxyl groups are available to carry out the radical scavenging activity.44 Li et al.45 reported that the addition of clove oil into chitosan/starch-based films caused a 10-fold increase in antioxidant activity. The 2-2Diphenyl-1-Picrylhydrazyl (DDPH) radical scavenging activity of the chitosan/starch films was only 1.78% but the value increase to 52.2% in the presence of 12% clove oil and 3% of nano titanium dioxide. Another study showed that the antioxidant activity for 75/25 wt% and 100/0 wt% of chitosan/ TEMPO cellulose nanofibers (CNFs) films towards DPPH radical scavenging was 2.1 and 8.2 (mg Trolox activity/g sample) respectively. Hence, the study concluded that antioxidant activities have linear relationship with the concentration of chitosan.41 In a study by Kan, et al.,46 chitosan/gelatin-based films demonstrated low DPPH radical scavenging activity which was 3.89% but the incorporation of the extract of hawthorn fruits increase the value to 84.4%. which could be due to the release of polyphenols from the matrix as polyphenols have potent antioxidant activity due to their ability to donate hydrogen atoms to react with free radicals.
FABRICATION METHODS OF CHITOSAN-BASED FILMS
Direct Casting Method
The Direct casting method is the simplest and widely use method for the development of biopolymer films. In this method, the steps start by dissolving the chitosan in a solution of a weak acid followed by the addition of other active compound (example: plasticizers, cross-linkers, fillers, etc). Then, blend with other polymers together if required. Stir the mixture to get a homogenous and viscous solution. Remove air bubbles and insoluble particles by filtration or centrifugation. Next, the solution was poured on leveled and flat surface of definite shape and size and dry the solution under specific conditions for a certain time. Peel off the film formed.47 However, casting methods require specialized equipment for drying such as hot air oven, vacuum oven, etc. Casted films have been applied to many food products as they ensure food safety by enhancing shelf life and maintain the quality. However, care must be taken when performing this method as little variations in the formulation can greatly affect the properties of the films.12,48
Melt-extrusion Methods
This method is another widely developed method in producing chitosan-based active packaging films. The steps in this method according to Goskonda and Upadrashta, 1993 and Steckel and Mindermann-Nogly, 2004 as cited by Priyadarshi and Rhim13 involve the formulation of materials with several compounds and mixing or blending of the material. The third step involves the blend material extrusion under specified condition. Next, the extrudates will be cut into pellets using a pelletizer before they are dried. The pellets are then extruded into flat sheets using a twin-screw extruder attached with a flat die or a blown film extruder with an annular die can also be used to produce a blown film. Generally, chitosan films made through extrusion process demonstrate good thermal stability, mechanical and antimicrobial properties. However, it is important to consider the processing temperature and the interaction among polymers to gain the best performance of the films.11,49–51
CHITOSAN FILMS
Pure Chitosan-based Films
Pure chitosan films have been developed by few researchers without the addition of other polymers but still include additives such as plasticizers, dilute acids or emulsifiers. Chitosan that is dissolved in dilute acid solutions have been successfully developed for preservation of various food products including vegetables, fruits, meat and fish.52 Despite the food packaging characteristic shown by chitosan films, their applications in food packaging is still unsatisfying due to low mechanical and thermal stability and high-water sensitivity which could lead to shorter shelf life of the foods14,53 Table 1 depicts the properties of chitosan films.
M w | MC (%) | T (A 600 /T) | WVP (x10 13 gcm -1 s -1 Pa -1 ) | OP (cm 3 µm m -2 day -1 kPa -1 ) | TS (MPa) | EB (%) |
---|---|---|---|---|---|---|
LMw | 19.43 | 0.760 | 9.21 | 7.70 | 55.83 | 4.58 |
HMw | 15.70 | 0.754 | 8.07 | 6.65 | 61.82 | 4.59 |
Blended Chitosan Films
A blend film is a combination of at least two compatible polymers or copolymers that are often developed in order to improve the physical properties of the films.54,55 The objective of polymer blending is to develop composite films in a simple and cost-effective way which would combine the properties of both components, possibly improving their attributes and minimizing drawbacks.56,57 Several properties such as physical, mechanical, barrier, and optical properties will determine the success of the polymer blending. Hence, an understanding these features is an essential part in developing blend films. Various published studies have been done regarding chitosan blended with other polymers due to the growing interest towards chitosan for food packaging applications. In this study, chitosan blends are classified into two groups, chitosan and natural biopolymers blends and chitosan and synthetic polymers blends.
Chitosan and Natural Biopolymers Blends
A blend of chitosan with other natural biopolymers such as polysaccharides and proteins can enhance the functional properties of chitosan-based films.36,54,58 Chitosan is compatible with these polymers due to its ability to associate through electrostatic interactions as is possesses highly polar groups such as OH, NH/NH2, C=O, in its backbone. This will allow the formation of intermolecular hydrogen bonds with the corresponding functional groups of other natural biopolymers.59
Chitosan and Polysaccharides blend films
A study reported that chitosan can be blended with polysaccharides such as starch, pectin, xanthan gum, carrageenan, xylan alginate, cellulose and its derivatives.60 Polysaccharides are not only increase hydrophilicity of other polymers, they also enhance tensile strength and elongation at break beside possessing antioxidant properties which make them suitable to be used for active packaging.61 Costa et al.62 developed chitosan/ cellulose nanocrystal (CNC) films by solvent casting which are used for meat packaging. In their study, chitosan films with the incorporation of CNC showed that the tensile strength increase to 8.93, 13.0 and 25.3 MPa compared to that of chitosan films without CNC (7.98 MPa) as the concentration of CNC increased. Nevertheless, the elongation at break which was evaluated to observe elasticity of films, was relatively unchanged with CNC incorporation into chitosan. The Young’s modulus of chitosan with CNC increased to 26.1, 40.2 and 92.7 MPa when the concentration of CNC is 5, 10 and 50 wt % respectively indicating enhanced films’ stiffness. Meanwhile, the chitosan films with 10 and 25% wt of CNC showed that the films were completely bactericidal and fungicidal against gram-positive and negative bacteria sand Candida albicans respectively. In addition, chitosan CNC film had better efficiency in delaying meat spoilage compared to chitosan films alone, demonstrating lower TVB-n value.
Another study reported biodegradable food packaging with purple yam starch and chitosan using the casting method. The yam starch/chitosan (YS/CS) films presented homogenous surfaces due to the absence of starch granules as there is a complete solubilization of chitosan and starch in the filmogenic solutions. The higher concentration of chitosan caused an increased in water vapor permeability of the films but reduced the films’ moisture content. The researchers also evaluated the ability of YS/CS films to preserve fruits. Apples demonstrated lower weight loss when they were coated with YS/CS films compared to that of control apples without coating. It was found that lower weight loss was observed as the concentration of chitosan increased. This study also observed a better internal visual appearance of the apples coated with yam starch and 0.5 wt % of chitosan in accordance with lower weight loss observed in the apples.63 In a study by Kamdem, DP and his colleagues, chitosan-based films with the incorporation of different concentration of xylan and carvacrol was developed for food packaging application. The addition of 25 wt % of xylan to the chitosan films resulted in maximum increase in tensile strength of the films from 60.9 MPa to 86.2 MPa. The study also reported a linear relationship between concentration of xylan and elongation at break of chitosan films where the maximum value (4.5%) was observed in chitosan films with 8 wt. % xylan. Moreover, incorporation of xylan reduced the degradation rate of the films. The films of chitosan with 0 to 25 wt. % xylan and carvacrol did not show significant inhibition to both Listeria innocua and Escherichia coli.64
Active edible coatings and films had been developed by casting method based on blends of cassava starch/chitosan (CS/CH) with the incorporation of pitanga (Eugenia uniflora L.) leaf extract (PE) and/ or natamycin. PE was evaluated as an antifungal and antioxidant agent for the films. In the study, insoluble particles were observed in the films upon incorporation of PE and/or natamycin. However, addition of natamycin rendered the films opaque with significantly greater value while PE only increased opacity slightly. Consequently, the films incorporated with PE and/ or natamycin were observed to be good barriers to UV light compared to that of films without additives. The mechanical properties of films were evaluated through four properties. Firstly, the tensile strength of CS/CH films was increased slightly upon addition of natamycin but no changes was found when PE was added due to the limitation by starch on the interaction between chitosan and phenol found in PE.65 Similarly, the elastic modulus was also increased with the addition of natamycin, indicating loss of film flexibility and stretch ability while the puncture force and deformation decreased in CS/CH films with PE and natamycin. Radical scavenging of the films with additives increased due to enhanced antioxidant activity of natamycin incorporation in the films. With regard to antifungal activity, CS/CH films that only contain natamycin was reported to demonstrate inhibition against Aspergillus flavus and Aspergillus parasiticus, which is beneficial to prevent fungal contamination in food.66
Xu et al. (2019) developed edible plasticized hemicelluloses/ chitosan films. Chitosan and hemicellulose show good miscibility due to the formation of abundant hydrogen bonds between chitosan and hemicellulose.67 However, they also incorporated cellulose nanofiber (CNF) to improve the flexibility and mechanical strength of the films. The resulting films with 5-20% CNF exhibited a significant increase in tensile strength. Conversely, tensile strain at break had decreased and the highest tensile strength and tensile strain at break were observed when 5 % CNF and 20-30% plasticizer were added. Nevertheless, the higher tensile strength observed in sorbitol-plasticized films showed slightly lower water vapor permeability due to the crystalline structure of CNF that inhibit the water vapor, resulting in lower permeability.68 The oxygen permeability of hemicellulose/ chitosan films was below 38.9 cm3 um/m2∙day∙kPa, indicating that the material was good oxygen barrier for food packaging purposes and thereby enhance the quality of the food.69
Chitosan and Protein blend films
Proteins have been used in the development of biodegradable films and the sources or protein can be animal such as collagen, gelatin, casein, etc. or plant sources such as corn zein, soy protein, whey gluten, quinoa protein, etc. Its high abundance, good mechanical properties and good gas barrier properties contribute to the development of protein-based films.70 However, blends of protein like gelatin with chitosan demonstrate good filmogenic property due to the compatibility of both biopolymers to form very homogenous systems.71 This is because both polymers are able to associate through hydrogen and electrostatic bonding as chitosan is positively charged while gelatin’s functional group are negatively charged.72
A study has developed blends of gelatin and chitosan biodegradable films with the incorporation of eugenol and ginger essential oil as antioxidants using a casting method. The resulting films with the incorporation of eugenol and ginger essential oil were more opaque than the gelatin/chitosan only films and roughness of films was also increased which led to discontinuities in film structure. Even though the films without antioxidants showed good UV barrier, the total barrier was still not achieved and the study showed that the addition of antioxidants significantly enhanced the UV/visible light barrier properties which is suitable for the packaging of foods prone to oxidation such as nuts and dairy products.71 High resistance of films was observed in pure chitosan films with or without antioxidants while gelatin/chitosan films presented a lower resistance or tensile strength. Regarding the elongation at break, it was observed that both antioxidants caused an increase in elongation at break values for pure chitosan and gelatin/chitosan films. Despite the high-water vapor permeability observed in all films regardless of antioxidants presence, a significant increase of antioxidant activity was observed in films contain antioxidant agents, especially with eugenol.59
Yadav et al.73 have developed eco-friendly chitosan-gelatin films incorporated with quercetin-starch using casting method. Their study showed that chitosan-gelatin films with quercetin-starch has caused a slightly increase absorption in UV-vis region. Since high absorption in UV region promote filtration of high energy UV rays, therefore low UV ray exposure will be transmitted to foods. Regarding the structure of the films, chitosan-gelatin films had smooth and even surface but the films became irregular in shape and uneven rough structure when quercetin-starch was added. In the meantime, decreased in water solubility of quercetin-starch incorporated chitosan-gelatin films was observed, indicating that the films had high resistance which could be due miscibility of amino groups in chitosan and gelatin with phenol group in quercetin-starch.74 Likewise, quercetin-starch and gelatin increased the tensile strength of chitosan films to 17.11 MPa and 16.10 MPa respectively but they decrease the elongation at break of the chitosan films. Factors that contribute to high tensile strength are most probably due the compatibility and greater molecular interactions between chitosan and gelatin or quercetin-starch.26 The water vapor permeability and oxygen permeability were observed to be lowest in quercetin-starch incorporated chitosan/gelatin films compared to that of pure chitosan films and chitosan-gelatin films. The antimicrobial activity of the resulting films showed that the zone of inhibition of quercetins-starch incorporated chitosan-gelatin film increased against Bacillus subtilis and Escherichia coli, suggesting the potential of quercetin-starch as an antimicrobial agent.73
Uranga, et al.75 developed citric acid-incorporated fish gelatin/ chitosan films using casting method. The resulting films were rough but flexible. The gelatin films with 10% and 20% citric acid demonstrated a decrease in swelling rate and swelling values similar to that of gelatin films with chitosan. However, the lowest swelling values were observed when 20% citric acid incorporated in the gelatin films without the addition of chitosan. Chitosan lowered the swelling values as it promoted the interactions with polar groups within the components in the formulation, resulting to less interactions of less polar groups with water molecules. In addition, the films demonstrated good UV barrier properties when citric acid and chitosan were added due to the presence of carboxyl and hydroxyl auxochrome groups of citric acid and chitosan and the tyrosine and phenylalanine in gelatin.76,77 Regarding the mechanical properties, citric acid caused a decrease in tensile strength but an increase in elongation at break. However, when chitosan was added to 20% citric acid-incorporated gelatin films, both tensile strength and elongation increased in accordance to the concentration of chitosan. The films’ antibacterial activity was evaluated against Escherichia coli. There was only slight reduction of E. coli growth in films with citric acid but a significant reduction was seen when chitosan was added to the gelatin film with 20% citric acid regardless of its content.75
Bhuimbar et al.78 used chitosan to develop antibacterial active food packaging films with the addition of acid soluble collagen obtained from skin black ruff fish and 5% pomegranate peel extract. The collagen/chitosan films appeared to be smooth, shiny and transparent. However, when pomegranate peel extract was incorporated, the films became translucent and yellow in color. The use of 3% and 5% pomegranate peel extract resulted in a decrease in film solubility. The author also claimed that the films possessed good mechanical strength as the films were easy to peel without cracking. Regarding the antimicrobial properties of the films, the collagen/chitosan blended films did not exhibit any antimicrobial activity. Nevertheless, when 5% pomegranate peel extract was added, the resulting films showed effectiveness against Bacillus subtilis, Salmonella typhi, and Escherichia coli.
Chitosan and Synthetic Polymers blend films
Blending of chitosan with synthetic polymers such as polyvinyl alcohol (PVA), polyvinyl pyrrolidone (PVP) or polylactic acid (PLA) has been widely studied for their benefits on mechanical, physical, and biological properties of the blended films. Low cost of production, chemical resistance as well as mechanical properties are the factors affecting the success of synthetic polymers as biodegradable materials.79 Chitosan is most likely to be miscible with several synthetic polymers by forming intermolecular hydrogen bonds between amine and hydroxyl groups of chitosan and hydroxyl groups of synthetic polymers.80 Annu et al.81 developed chitosan/PVA based films with the incorporation of natural extract of Ocimum tenuiflorum using the solvent casting method. The resulting films were thin, homogenous, and uniform. Regarding the optical properties, chitosan/PVA films incorporated with natural extract demonstrated low transmission in UV light region, indicating that the films possessed an excellent UV barrier due to the presence of polyphenol, carbonyl compounds, and benzene ring that enhance n→π* absorption.82 The antioxidant activity of the films was determined through DPPH assay. Pure chitosan with the incorporation of natural extract showed greater scavenging activity (54.0%) as compared to chitosan/PVA films (27.42%). Despite the enhanced UV barrier properties and antioxidant activity, the study did not evaluate the films’ mechanical strength and shelf-life properties.
Nwabor et al.83 developed chitosan/PVA containing Eucalyptus leaf extract via solvent casting and particulate leaching. The resulting films of 1.75:0.25 chitosan-PVA/Ag nanoparticles demonstrated higher water vapor permeability which could expose food products to water and can lead to speedy deterioration and spoilage of packaged foods. However, a greater proportion of Ag nanoparticles (1:1 and 1.5:0.5 chitosan-PVA/Ag nanoparticles) had decreased the water vapor permeability due to reduced motility of the polymer chains when Ag nanoparticles exists as a discontinuous state in the film matrix which will disrupt the diffusion of water vapor.84 The tensile strength and elongation at break of the chitosan-PVA/Ag nanoparticles was enhanced but the effects of Ag nanoparticles on tensile strength and elongation at break were not significant. The contact angle measurement revealed that all the films were hydrophilic with contact angles less than 90° but the incorporation of Ag nanoparticles increasing the contact angles due to an increase in the hydrophobicity of the chitosan-PVA/Ag nanoparticles films. Regarding the antimicrobial activity of the films, pure chitosan and PVA films did not present any antimicrobial effects but when Ag nanoparticles were incorporated, there were 99.9% reduction in CFU/mL observed against Listeria monocytogenes, Staphylococcus aureus and Escherichia coli. The results of the DPPH and ABTS demonstrated that both chitosan/PVA and chitosan-PVA/Ag nanoparticles films possessed antioxidant activity with highest activity was observed in 1:1 chitosan-PVA/ Ag nanoparticles films. The study also evaluates the shelf life of chicken sausage wrapped with the films. the results showed that 1:1 film did not show any sign of spoilage and remained visually intact while 1.5:0.5 film presented coloration and growth of fungi after storage for 15 days. However, pure chitosan/PVA films started showing spoilage signs after 7 days. This indicated that chitosan-PVA/Ag nanoparticles films could serve as food packaging that could extend the shelf life of food products.
A study to develop films to keep the freshness of fish was conducted by Vadivel et al.85 This study used blends of polymers: chitosan (S), PVA, xylan (CC) and hydroxyapatite (nHA) as well as curcumin (Cur) as an antioxidant for film development. The resulting films of PVA/CS/CC had a fractured layer close to the film surface which was due to the existence of intermolecular hydrogen bond and orientation and arrangement of PVA molecules in the composite systems which are related to the presence of semi-crystalline nature. However, the surface of PVA/ CS/CC/nHA films were compact and smooth. The biodegradation evaluation of films in vitro showed that lower degradation by enzyme were observed in PVA/CS/CC/nHA scaffolds while increased resistance to biodegradation was observed when Cur was incorporated. Besides, the antimicrobial studies revealed that the PVA/CS/CC/nHA with Cur films has higher zone of inhibition towards Escherichia coli, Staphylococcus aureus, Aspergillus niger, and Aspergillus flavus compared to that of PVA/CS/CC films. The DPPH radical scavenging assay results showed that the DPPH solution turned from purple to yellow upon the insertion of PVA/ CS/CC/nHA with Cur films, indicating the radical scavenging ability of the films. Fathima et al.86 studied on the development of composite films using polylactic acid/nano-chitosan (PLA/ NCS) with PVA as plasticizer and polyethylene glycol as a cross linking agent. The surface morphology of resulting films of PLA/NCS reveal a rough film surface which could potentially provide adherence to bacterial cell walls and hence, enhance the antimicrobial activity of films. Regarding mechanical properties, the tensile strength decreases as concentration of NCS increases and the lowest value was observed in 2% PLA/NCS films (161.37 kg/cm3) while higher values were observed in 0.5% PLA/NCS films (346.78 kg/cm3). Likewise, a decrease in elongation was detected as the concentration of NCS increased which occurred due to weak interfacial adhesion between PLA and NCS. The oxygen transmission rate was lowest in 0.5% PLA/NCS films (282 ml/m2/day) and highest in 2% PLA/NCS films (2134 ml/ m2/day). In contrast, the water vapor transmission rate was highest in 0.5% PLA/NCS films (7.12 g/m2/24h) and lowest in 2% PLA/NCS films (5.15 g/m2/24h). The PLA/NCS films exhibited antimicrobial activity with greater inhibition against Listeria monocytogenes (gram-positive bacteria) than Escherichia coli (gram-negative bacteria) owing to bacteriostatic and bactericidal properties of chitosan.
Haghighi et al.87 developed antimicrobial films for food packaging applications using polymer blends of chitosan (CS) and PVA with the incorporation of ethyl lauroyl arginate (LAE). The resulting films were homogenous and smooth but aggregation on the film surfaces were observed when the concentration of LAE increased up to 10%. Sponge shape and irregular structure were also observed in films containing 5% and 10% LAE. These results were obtained as functional groups of LAE disturbed the interaction between the polymer chains.88 Meanwhile, CS/PVA films without LAE showed highest tensile strength (42.5 MPa), elongation at break (54.2%) and elastic modulus (1570 MPa). However, when 10% LAE was added, the tensile strength and elongation at break reduced to 15.70 MPa and 14.31% respectively, indicating that such films were less stretchable and less resistant than CS/PVA. This is due to inability of LAE to create a continuous and cohesive matrix. The reduction in mechanical properties were attributed to limited cohesion forces in the film matrix due to the competitive interaction between the functional groups of LAE and CS/PVA leading to weak intermolecular hydrogen bonding. UV barrier analysis demonstrated that reduced UV light transmittance was observed in films with increasing LAE concentration. Exceeding the critical concentration of LAE can result in the formation of aggregates that are large enough to scatter light and thus disrupt transmission.89 Water content and water vapor permeability increases upon incorporation of LAE into the films. Regarding the antimicrobial evaluation, pure CS/PVA films did not demonstrate any bacterial inhibition activity against food pathogens; Campylobacter jejuni, Listeria monocytogenes, and Salmonella typhimurium despite the antimicrobial property possessed by chitosan which could be due to its limitation to diffuse into the agar medium.90 Nevertheless, incorporated 1% LAE CS/PVA films showed inhibition zone against C. jejuni only.
CONCLUSION
Conventional plastic packaging has labeled the food industry as a source of pollution with a number of social and environmental concerns due to insufficient waste management systems and non-biodegradability. Thus, biodegradable and biobased materials have received considerable attention to overcome these problems recently. Chitosan adoption as packaging material shows benefits in mitigating these environmental concerns, despite some downside in terms of barrier and mechanical properties as well as the production costs. Blended films of chitosan with other synthetic or natural polymers such as polyvinyl alcohol, carboxymethyl cellulose, methylcellulose, contribute towards the enhancement of various physico-chemical properties including its mechanical and barrier properties. The antimicrobial and antioxidant properties of chitosan-based films could contribute towards developing a functional food packaging against microbial and oxidative degradation. This approach may prove promising for future innovative of food packaging design as it will replace the existing plastic packaging materials with those that can provide equal or better properties than synthetic polymers.
References
- Ritchie H, Roser M. Plastic pollution: Our World in data; 2018. (Available from: https://ourworldindata.org/plastic-pollution [cited 24/11/2022] [Google Scholar]
- Tanhua T, Gutekunst SB, Biastoch A. A near-synoptic survey of ocean microplastic concentration along an around-the-world sailing race. PLOS ONE. 2020;15(12):e0243203 [PubMed] | [CrossRef] | [Google Scholar]
- Eriksen M, Lebreton LCM, Carson HS, Thiel M, Moore CJ, Borerro JC, et al. Plastic pollution in the world’s oceans: More than 5 trillion plastic pieces weighing over 250,000 tons afloat at sea. PLOS ONE. 2014;9(12):e111913 [PubMed] | [CrossRef] | [Google Scholar]
- Marine plastics. International Union for Conservation of Nature; 2018 [cited Nov 20 2020]. Available from: https://www.iucn.org/resources/issues-briefs/marine-plastics#:~:text=Plastic%20pollution%20is%20the%20most,and%20contributes%20to%20 climate%20change [PubMed] | [CrossRef] | [Google Scholar]
- Cui H, Surendhiran D, Li C, Lin L. Biodegradable zein active film containing chitosan nanoparticle encapsulated with pomegranate peel extract for food packaging. Food Packaging Shelf Life. 2020:24 [CrossRef] | [Google Scholar]
- Yam KL, Lee DS. Emerging food packaging technologies: An overview. Emerg Food Packaging Technol Principle Pract. 2012:1-9. [CrossRef] | [Google Scholar]
- Arikan EB, Ozsoy HD. A review: Investigation of Bioplastics. J Civ Eng Archit. 2015;9(22):188-92. [CrossRef] | [Google Scholar]
- Philp JC, Bartsev A, Ritchie RJ, Baucher MA, Guy K. Bioplastics science from a policy vantage point. N Biotechnol. 2013;30(6):635-46. [PubMed] | [CrossRef] | [Google Scholar]
- Kumar S, Thakur K. Bioplastics. Classification, production and their potential food applications. J Hill Agric. 2017;8(2) [PubMed] | [CrossRef] | [Google Scholar]
- Dutta J, Tripathi S, Dutta PK. Progress in antimicrobial activities of chitin, chitosan and its oligosaccharides: A systematic study needs for food applications. Food Sci Technol Int. 2012;18(1):3-34. [PubMed] | [CrossRef] | [Google Scholar]
- Lago MA, Sendón R, De Quirós ARB, Sanches-Silva A, Costa HS, Sánchez-Machado DI, et al. Preparation and characterization of antimicrobial films based on chitosan for active food packaging applications. Food Bioprocess Technol. 2014;7(10):2932-41. [CrossRef] | [Google Scholar]
- Mujtaba M, Morsi RE, Kerch G, Elsabee MZ, Kaya M, Labidi J, et al. Current advancements in chitosan-based film production for food technology; A review. Int J Biol Macromol. 2019;121:889-904. [PubMed] | [CrossRef] | [Google Scholar]
- Priyadarshi R, Rhim JW. Chitosan-based biodegradable functional films for food packaging applications. Innov Food Sci Emerg Technol. 2020:62 [CrossRef] | [Google Scholar]
- Elsabee MZ, Abdou ES. Chitosan based edible films and coatings: A review. Mater Sci Eng C Mater Biol Appl. 2013;33(4):1819-41. [PubMed] | [CrossRef] | [Google Scholar]
- Cheong KS, Balasubramaniam JR, Hung YP, Chuong WS, Amartalingam R. Development of biodegradable plastic composite blends based on sago derived starch and natural rubber. Pertanika J Sci Technol. 2010;18(2):411-20. [PubMed] | [CrossRef] | [Google Scholar]
- Shirai MA, Grossmann MV, Mali S, Yamashita F, Garcia PS, Müller CM, et al. Development of biodegradable flexible films of starch and poly(lactic acid) plasticized with adipate or citrate esters. Carbohydr Polym. 2013;92(1):19-22. [PubMed] | [CrossRef] | [Google Scholar]
- K SS, M PI, G RR. Mahua oil-based polyurethane/chitosan/Nano ZnO composite films for biodegradable food packaging applications. Int J Biol Macromol. 2019;124:163-74. [PubMed] | [CrossRef] | [Google Scholar]
- Hasan M, Rahmayani RFI, Munandar Array. Bioplastic from chitosan and yellow pumpkin starch with castor oil as plasticizer. IOP Conf Ser.: Mater Sci Eng. 2018;333(1) [CrossRef] | [Google Scholar]
- Zargar V, Asghari M, Dashti A. A Review on Chitin and Chitosan Polymers: Structure, Chemistry, Solubility, Derivatives, and Applications. Chem Bio Eng Reviews. 2015;2(3):204-26. [CrossRef] | [Google Scholar]
- Pereda M, Amica G, Marcovich NE. Development and characterization of edible chitosan/olive oil emulsion films. Carbohydr Polym. 2012;87(2):1318-25. [CrossRef] | [Google Scholar]
- Dutta PK, Tripathi S, Mehrotra GK, Dutta J. Perspectives for chitosan based antimicrobial films in food applications. Food Chem. 2009;114(4):1173-82. [CrossRef] | [Google Scholar]
- Zhang H, Li R, Liu W. Effects of chitin and its derivative chitosan on postharvest decay of fruits: a review. Int J Mol Sci. 2011;12(2):917-34. [PubMed] | [CrossRef] | [Google Scholar]
- Liu X, Xu Y, Zhan X, Xie W, Yang X, Cui SW, et al. Development and properties of new kojic acid and chitosan composite biodegradable films for active packaging materials. Int J Biol Macromol. 2020;144:483-90. [PubMed] | [CrossRef] | [Google Scholar]
- Kerch G, Korkhov V. Effect of storage time and temperature on structure, mechanical and barrier properties of chitosan-based films. Eur Food Res Technol. 2011;232(1):17-22. [CrossRef] | [Google Scholar]
- Zhong Y, Li Y. Effects of storage conditions and acid solvent types on structural, mechanical and physical properties of kudzu starch ()-chitosan composite films. Starch/Stärke. 2011;63(9):579-86. [CrossRef] | [Google Scholar]
- Leceta I, Guerrero P, De la Caba K. Functional properties of chitosan-based films. Carbohydr Polym. 2013;93(1):339-46. [PubMed] | [CrossRef] | [Google Scholar]
- Dayarian S, Zamani A, Moheb A, Masoomi M. Physico-mechanical properties of films of chitosan, carboxymethyl chitosan, and their blends. J Polym Environ. 2014;22(3):409-16. [CrossRef] | [Google Scholar]
- Vlacha M, Giannakas A, Katapodis P, Stamatis H, Ladavos A, Barkoula NM, et al. On the efficiency of oleic acid as plasticizer of chitosan/clay nanocomposites and its role on thermo-mechanical, barrier and antimicrobial properties-comparison with glycerol. Food Hydrocoll. 2016;57:10-9. [CrossRef] | [Google Scholar]
- Giannakas A, Patsaoura A, Barkoula NM, Ladavos A. A novel solution blending method for using olive oil and corn oil as plasticizers in chitosan based organoclay nanocomposites. Carbohydr Polym. 2017;157:550-7. [PubMed] | [CrossRef] | [Google Scholar]
- Mohd Isa SA, Mohamed R. Physical and mechanical propertied of chitosan and polyethylene blend. Int J Mech Prod Eng. 2015;3(10):51-5. [PubMed] | [CrossRef] | [Google Scholar]
- Van den Broek LAM, Knoop RJI, Kappen FHJ, Boeriu CG. Chitosan films and blends for packaging material. Carbohydr Polym. 2015;116:237-42. [PubMed] | [CrossRef] | [Google Scholar]
- Hosseinnejad M, Jafari SM. Evaluation of different factors affecting antimicrobial properties of chitosan. Int J Biol Macromol. 2016;85:467-75. [CrossRef] | [Google Scholar]
- Caro N, Medina E, Díaz-Dosque M, López L, Abugoch L, Tapia C, et al. Novel active packaging based on films of chitosan and chitosan/quinoa protein printed with chitosan-tripolyphosphate-thymol nanoparticles via thermal ink-jet printing. Food Hydrocoll. 2016;52:520-32. [CrossRef] | [Google Scholar]
- Qin Y, Li P, Guo Z. Cationic chitosan derivatives as potential antifungals: A review of structural optimization and applications. Carbohydr Polym. 2020:236-116002. [PubMed] | [CrossRef] | [Google Scholar]
- Mousavi Khaneghah A, Hashemi SMB, Limbo S. Antimicrobial agents and packaging systems in antimicrobial active food packaging: An overview of approaches and interactions. Food Bioprod Process. 2018;111:1-19. [CrossRef] | [Google Scholar]
- Vodnar DC, Pop OL, Dulf FV, Socaciu C. Antimicrobial efficiency of edible films in food industry. Notular Botanicae Horti Agrobotanici Cluj-Napoca. 2015;43(2):302-12. [CrossRef] | [Google Scholar]
- Leceta I, Peñalba M, Arana P, Guerrero P, De La Caba K. Ageing of chitosan films: Effect of storage time on structure and optical, barrier and mechanical properties. Eur Polym J. 2015;66:170-9. [CrossRef] | [Google Scholar]
- Lee JH, Lee JH, Yang HJ, Song KB. Preparation and characterization of brewer’s spent grain protein-chitosan composite films. J Food Sci Technol. 2015;52(11):7549-55. [CrossRef] | [Google Scholar]
- Kakaei S, Shahbazi Y. Effect of chitosan-gelatin film incorporated with ethanolic red grape seed extract and essential oil on survival of Listeria monocytogenes and chemical, microbial and sensory properties of minced trout fillet. LWT Food Sci Technol. 2016;72:432-8. [CrossRef] | [Google Scholar]
- Soni B, Mahmoud B, Chang S, El-Giar EM, Hassan EB. Physicochemical, antimicrobial and antioxidant properties of chitosan/TEMPO biocomposite packaging films. Food Packaging Shelf Life. 2018;17:73-9. [CrossRef] | [Google Scholar]
- Wei L, Tan W, Wang G, Li Q, Dong F, Guo Z, et al. The antioxidant and antifungal activity of chitosan derivatives bearing Schiff bases and quaternary ammonium salts. Carbohydr Polym. 2019;226:115256 [PubMed] | [CrossRef] | [Google Scholar]
- Castro Marín A, Culcasi M, Cassien M, Stocker P, Thétiot-Laurent S, Robillard B, et al. Chitosan as an antioxidant alternative to sulphites in oenology: EPR investigation of inhibitory mechanisms. Food Chem. 2019;285:67-76. [PubMed] | [CrossRef] | [Google Scholar]
- Sun T, Zhou D, Mao F, Zhu Y. Preparation of low-molecular-weight carboxymethyl chitosan and their superoxide anion scavenging activity. Eur Polym J. 2007;43(2):652-6. [CrossRef] | [Google Scholar]
- Li W, Zheng K, Chen H, Feng S, Wang W, Qin C, et al. Influence of Nano titanium dioxide and clove oil on chitosan-starch film characteristics. Polymers. 2019;11(9):1418 [PubMed] | [CrossRef] | [Google Scholar]
- Kan J, Liu J, Yong H, Liu Y, Qin Y, Liu J, et al. Development of active packaging based on chitosan-gelatin blend films functionalized with Chinese hawthorn () fruit extract. Int J Biol Macromol. 2019;140:384-92. [PubMed] | [CrossRef] | [Google Scholar]
- Shankar S, Rhim JW. Preparation of sulfur nanoparticle-incorporated antimicrobial chitosan films. Food Hydrocoll. 2018;82:116-23. [CrossRef] | [Google Scholar]
- Zhang Y, Liu BL, Wang LJ, Deng YH, Zhou SY, Feng JW, et al. Preparation, structure and properties of acid aqueous solution plasticized thermoplastic chitosan. Polymers. 2019;11(5) [PubMed] | [CrossRef] | [Google Scholar]
- Alix S, Mahieu A, Terrie C, Soulestin J, Gerault E, Feuilloley MGJ, et al. Active pseudo-multilayered films from polycaprolactone and starch-based matrix for food-packaging applications. Eur Polym J. 2013;49(6):1234-42. [CrossRef] | [Google Scholar]
- Woranuch S, Yoksan R. Eugenol-loaded chitosan nanoparticles: II. Application in bio-based plastics for active packaging. Carbohydr Polym. 2013;96(2):586-92. [PubMed] | [CrossRef] | [Google Scholar]
- Woranuch S, Yoksan R, Akashi M. Ferulic acid-coupled chitosan: Thermal stability and utilization as an antioxidant for biodegradable active packaging film. Carbohydr Polym. 2015;115:744-51. [PubMed] | [CrossRef] | [Google Scholar]
- Ali A, Zahid N, Manickam S, Siddiqui Y, Alderson PG. Double layer coatings: A new technique for maintaining physico-chemical characteristics and antioxidant properties of dragon fruit during storage. Food Bioprocess Technol. 2014;7(8):2366-74. [CrossRef] | [Google Scholar]
- Al-Tayyar NA, Youssef AM, Al-Hindi R. Antimicrobial food packaging based on sustainable bio-based materials for reducing foodborne pathogens: A review. Food Chem. 2020;310:125915 [PubMed] | [CrossRef] | [Google Scholar]
- Cazón P, Vázquez M. Mechanical and barrier properties of chitosan combined with other components as food packaging film. Environ Chem Lett. 2020;18(2):257-67. [CrossRef] | [Google Scholar]
- . Polymers and polymeric composite: A reference series. 2018:1-38. Ineditors [CrossRef] | [Google Scholar]
- . Array. 2015:1-6. Ineditors [CrossRef] | [Google Scholar]
- Unger M, Sedlmair J, Siesler HW, Hirschmugl C. 3D FT-IR imaging spectroscopy of phase-separation in a poly(3-hydroxybutyrate)/poly(L-lactic acid) blend. Vib Spectrosc. 2014;75:162-72. [CrossRef] | [Google Scholar]
- Aider M. Chitosan application for active bio-based films production and potential in the food industry: Review. LWT Food Sci Technol. 2010;43(6):837-42. [CrossRef] | [Google Scholar]
- Bonilla J, Poloni T, Lourenço RV, Sobral PJA. Antioxidant potential of eugenol and ginger essential oils with gelatin/chitosan films. Food Biosci. 2018;23:107-14. [CrossRef] | [Google Scholar]
- Wang H, Qian J, Ding F. Emerging chitosan-based films for food packaging applications. J Agric Food Chem. 2018;66(2):395-413. [PubMed] | [CrossRef] | [Google Scholar]
- Carina D, Sharma S, Jaiswal AK, Jaiswal S. Seaweeds polysaccharides in active food packaging: A review of recent progress. Trends Food Sci Technol. 2021;110:559-72. [CrossRef] | [Google Scholar]
- Costa SM, Ferreira DP, Teixeira P, Ballesteros LF, Teixeira JA, Fangueiro R, et al. Active natural-based films for food packaging applications: The combined effect of chitosan and nanocellulose. Int J Biol Macromol. 2021;177:241-51. [PubMed] | [CrossRef] | [Google Scholar]
- Martins da Costa JC, Lima Miki KS, Da Silva Ramos A, Teixeira-Costa BE. Development of biodegradable films based on purple yam starch/chitosan for food application. Heliyon. 2020;6(4):e03718 [PubMed] | [CrossRef] | [Google Scholar]
- Kamdem DP, Shen Z, Nabinejad O, Shu Z. Development of biodegradable composite chitosan-based films incorporated with xylan and carvacrol for food packaging application. Food Packaging Shelf Life. 2019:21 [CrossRef] | [Google Scholar]
- Talón E, Trifkovic KT, Nedovic VA, Bugarski BM, Vargas M, Chiralt A, et al. Antioxidant edible films based on chitosan and starch containing polyphenols from thyme extracts. Carbohydr Polym. 2017;157:1153-61. [PubMed] | [CrossRef] | [Google Scholar]
- Sirisha Nallan Chakravartula S, Lourenço RV, Balestra F, Quinta Barbosa Bittante AM, Sobral PJdA, Dalla Rosa M, et al. Influence of pitanga ( L.) leaf extract and/or natamycin on properties of cassava starch/chitosan active films. Food Packaging Shelf Life. 2020:24 [CrossRef] | [Google Scholar]
- Gabrielii I, Gatenholm P. Preparation and properties of hydrogels based on hemicellulose. J Appl Polym Sci. 1998;69(8):1661-7. [CrossRef] | [Google Scholar]
- Ibn Yaich A, Edlund U, Albertsson AC. Transfer of Biomatrix/Wood cell interactions to hemicellulose-based materials to control water interaction. Chem Rev. 2017;117(12):8177-207. [PubMed] | [CrossRef] | [Google Scholar]
- Xu J, Xia R, Zheng L, Yuan T, Sun R. Plasticized hemicelluloses/chitosan-based edible films reinforced by cellulose nanofiber with enhanced mechanical properties. Carbohydr Polym. 2019;224:115164 [PubMed] | [CrossRef] | [Google Scholar]
- Arfat YA, Ahmed J, Hiremath N, Auras R, Joseph A. Thermo-mechanical, rheological, structural and antimicrobial properties of bionanocomposite films based on fish skin gelatin and silver-copper nanoparticles. Food Hydrocoll. 2017;62:191-202. [CrossRef] | [Google Scholar]
- Bonilla J, Sobral PJA. Investigation of the physicochemical, antimicrobial and antioxidant properties of gelatin-chitosan edible film mixed with plant ethanolic extracts. Food Biosci. 2016;16:17-25. [CrossRef] | [Google Scholar]
- Benbettaïeb N, Chambin O, Assifaoui A, Al-Assaf S, Karbowiak T, Debeaufort F, et al. Release of coumarin incorporated into chitosan-gelatin irradiated films. Food Hydrocoll. 2016;56:266-76. [CrossRef] | [Google Scholar]
- Yadav S, Mehrotra GK, Bhartiya P, Singh A, Dutta PK. Preparation, physicochemical and biological evaluation of quercetin-based chitosan-gelatin film for food packaging. Carbohydr Polym. 2020;227:115348 [PubMed] | [CrossRef] | [Google Scholar]
- Piyada K, Waranyou S, Thawien W. Mechanical, thermal and structural properties of rice starch films reinforced with rice starch nanocrystals. Int Food Res. 2013;20:439-49. [PubMed] | [CrossRef] | [Google Scholar]
- Uranga J, Puertas AI, Etxabide A, Dueñas MT, Guerrero P, De la Caba K, et al. Citric acid-incorporated fish gelatin/chitosan composite films. Food Hydrocoll. 2019;86:95-103. [CrossRef] | [Google Scholar]
- Nagarajan M, Benjakul S, Prodpran T, Songtipya P. Properties and characteristics of nanocomposite films from tilapia skin gelatin incorporated with ethanolic extract from coconut husk. J Food Sci Technol. 2015;52(12):7669-82. [PubMed] | [CrossRef] | [Google Scholar]
- Jadhav JP, Phugare SS. Nonconventional textile waste water treatment. 2012 [PubMed] | [CrossRef] | [Google Scholar]
- Bhuimbar MV, Bhagwat PK, Dandge PB. Extraction and characterization of acid soluble collagen from fish waste: Development of collagen-chitosan blend as food packaging film. J Environ Chem Eng. 2019;7(2) [CrossRef] | [Google Scholar]
- El Bourakadi KE, Merghoub N, Fardioui M, Mekhzoum MEM, Kadmiri IM, Essassi EM, et al. Chitosan/polyvinyl alcohol/thiabendazoluim-montmorillonite bio-nanocomposite films: mechanical, morphological and antimicrobial properties. Composites Part B: Engineering. 2019;172:103-10. [CrossRef] | [Google Scholar]
- Bonilla J, Fortunati E, Atarés L, Chiralt A, Kenny JM. Physical, structural and antimicrobial properties of poly vinyl alcohol-chitosan biodegradable films. Food Hydrocoll. 2014;35:463-70. [CrossRef] | [Google Scholar]
- Annu AA, Ali A, Ahmed S. Eco-friendly natural extract loaded antioxidative chitosan/polyvinyl alcohol based active films for food packaging. Heliyon. 2021;7(3):e06550 [PubMed] | [CrossRef] | [Google Scholar]
- Dammak I, De Carvalho RA, Trindade CSF, Lourenço RV, Do Amaral Sobral PJ. Properties of active gelatin films incorporated with rutin-loaded nanoemulsions. Int J Biol Macromol. 2017;98:39-49. [PubMed] | [CrossRef] | [Google Scholar]
- Nwabor OF, Singh S, Paosen S, Vongkamjan K, Voravuthikunchai SP. Enhancement of food shelf life with polyvinyl alcohol-chitosan nanocomposite films from bioactive Eucalyptus leaf extracts. Food Biosci. 2020:36 [CrossRef] | [Google Scholar]
- Shankar S, Rhim JW, Won K. Preparation of poly(lactide)/lignin/silver nanoparticles composite films with UV light barrier and antibacterial properties. Int J Biol Macromol. 2018;107:1724-31. [CrossRef] | [Google Scholar]
- Vadivel M, Sankarganesh M, Raja JD, Rajesh J, Mohanasundaram D, Alagar M, et al. Bioactive constituents and bio-waste derived chitosan / xylan based biodegradable hybrid nanocomposite for sensitive detection of fish freshness. Food Packaging Shelf Life. 2019:22 [CrossRef] | [Google Scholar]
- Fathima PE, Panda SK, Ashraf PM, Varghese TO, Bindu J. Polylactic acid/chitosan films for packaging of Indian white prawn (). Int J Biol Macromol. 2018;117:1002-10. [PubMed] | [CrossRef] | [Google Scholar]
- Haghighi H, Leugoue SK, Pfeifer F, Siesler HW, Licciardello F, Fava P, et al. Development of antimicrobial films based on chitosan-polyvinyl alcohol blend enriched with ethyl lauroyl arginate (LAE) for food packaging applications. Food Hydrocoll. 2020:100 [CrossRef] | [Google Scholar]
- Gaikwad KK, Lee SM, Lee JS, Lee YS. Development of antimicrobial polyolefin films containing lauroyl arginate and their use in the packaging of strawberries. Food Measure. 2017;11(4):1706-16. [CrossRef] | [Google Scholar]
- Bonnaud M, Weiss J, McClements DJ. Interaction of a food-grade cationic surfactant () with food-grade biopolymers (pectin, carrageenan, xanthan, alginate, dextran, and chitosan). J Agric Food Chem. 2010;58(17):9770-7. [PubMed] | [CrossRef] | [Google Scholar]
- Leceta I, Guerrero P, Ibarburu I, Dueñas MT, De la Caba K. Characterization and antimicrobial analysis of chitosan-based films. J Food Eng. 2013;116(4):889-99. [CrossRef] | [Google Scholar]