ABSTRACT
Zinc Oxide Nanoparticles (ZnO NPs) have garnered interest for their potential anti-convulsant properties, offering a promising approach to epilepsy treatment. Their unique physicochemical traits, such as a large surface area and inherent antioxidant capacities, make ZnO NPs potent inhibitors of oxidative stress, a key factor in epileptogenesis. Experimental models have shown that ZnO NPs can reduce Reactive Oxygen Species (ROS) levels and protect against brain injury. By influencing neurotransmitter release, including glutamate and GABA, ZnO NPs help maintain the excitatory-inhibitory balance in the brain, potentially reducing seizure likelihood. Their nanoscale size facilitates effective blood-brain barrier crossing, enhancing the bioavailability of traditional anti-convulsant medications and boosting their therapeutic effects. ZnO NPs offer neuroprotection by regulating calcium influx and preserving neuronal membranes, defending neurons against excitotoxicity, common in seizures. Additionally, they enhance drug delivery by acting as carriers, increasing the bioavailability of standard anti-epileptic drugs and potentially improving treatment outcomes while minimizing side effects. In conclusion, ZnO NPs antioxidant properties, neurotransmission modulation, and drug delivery capabilities position them as a promising adjunct therapy for epilepsy. Further research is essential to maximize their therapeutic potential and ensure safety.
INTRODUCTION
Epilepsy is a long-term brain condition characterized by a persistent propensity to have seizures, unrelated to any direct injury to the central nervous system, as well as the neurobiologic, cognitive, psychological in nature and social effects of seizure recurrences.1 The work of neuroscientists in the middle of the nineteenth century the most significant of whom was John Hughlings Jackson in London is where current knowledge of epilepsy 1st emerged. Hughlings Jackson recognized partial epileptic seizures, confined at their source in separate regions of the cerebral cortex, at a time when epilepsy was used to describe a condition related to generalized seizures, which were thought to be caused by disruptions in the medulla oblongata. Jackson also established a scientific method for examining epileptic phenomena.2 Although seizures is usually thought to be a result of brain disorders like trauma to the brain, intracranial tumors, cerebrovascular illnesses, neurodegenerative illnesses, and genetic diseases or genetically associated with epileptic syndromes, approximately 20% of all seizures conditions are cryptogenic with an unknown origins, leading to numerous diagnostic and medicinal challenges.3 Antiepileptic drugs work on a variety of molecular targets to control the excitation of neurons in a way that prevents epilepsy-related activity without interfering with normal neuron-to-neuron communication. The operation of ion channels is essential for signaling. The principal inhibitory neurotransmitter, GABA, may be modified in neurons or transporters by anticonvulsant medications like benzodiazepines and barbiturates. By chance, the 1st anticonvulsant drugs were discovered, such as Phenytoin (PHT), Phenobarbital (PB), valproate, benzodiazepines, Primidone (PRD) and ethosuximide, which were later classified as the 1st generation of anticonvulsant drugs. Since 1990, the 2nd generation of medications Vigabatrin (VBG), Tiagabine (TGB), Levetiracetam (LEV), has been available for clinical use.4
Numerous fields, including medicine and physiology, promise to benefit significantly from advances in nanotechnology and nanoengineering. These compounds and devices may be designed for communication with tissues and cells at the molecular level with a great deal of functional specificity for applications in medicine and physiology. This allows for a level of connection between technologies and biological mechanisms that was previously unachievable.5 Nanomedicine encompasses the use of nanoscale structures for diagnoses, prevention, and treatment of diseases thus helping in improving the human health. Nanomedicine aimed at combining modern nanotechnology with classical molecular techniques and biotechnology to create innovative treatment for disease cure and repair of tissues, novel drug delivery systems, immediate and ultrasensitive diagnostics devices like biosensors, surgical aids, biopharmaceutics, implantable biomaterials, etc.,6 whereas Zinc Nanoparticles has been shown to slow the progression of neurodegenerative disorders and shield the blood-brain barrier from oxidative damage. Such as Zinc is used in the treatment of epilepsy due to its role in modulating neurotransmitter function and reducing oxidative stress in the brain. It acts as a cofactor for various enzymes and proteins involved in neuronal function, potentially stabilizing neural activity. Zinc supplementation has been shown to enhance the effectiveness of some antiepileptic drugs. Additionally, zinc can help mitigate the side effects associated with long-term use of these medications.7
NANOPARTICLES IN BIOMEDICAL RESEARCH
Nanotechnology represents one of the path changing discoveries of modern era. It encompasses numerous modalities based on the concepts of nanoscience in which products and procedures are made attainable by employing particles of nanoscale size range of 1-100 nm. So far, Nanoparticles have been produced from metal and non-metal, polymeric substances and bio ceramics. Amongst these numerous sources of NPs, metal oxide NPs is the primary one created on an industrial scale. Principal metal oxide NPs includes zinc, iron, silver oxide, titanium etc. Many biomedical uses including drug delivery, phototherapy and bio-sensing have been anticipated for them.8
Nanoparticles easily penetrate cells yet the mechanics involved are not fully understood. The nanoparticle influx happens by endocytosis; the particles are introduced and dispersed across the lipid bilayer in the cell membrane. Furthermore, these nanoparticles were proven to be able to penetrate the cells even after attachment to proteins such as antigens.9 The mononuclear phagocytic system can swiftly absorb NPs bigger than 200 nm, which can then be concentrated in the liver and spleen. In summary, small-size NPs are typically more beneficial for extended circulation and distribution in a variety of tissues. Additionally significant NPs properties regulating their biological in vivo courses include surface charge and hydrophobicity. Hydrophobic nanomaterials can increase NPs penetration whereas hydrophilic ones can inhibit the reticular endothelial system response.10
Engineered NPs, a product of nanotechnology, have changed the field of medicine and given doctors new hope for treating illnesses for which there are still no effective treatments. Some of the inorganic Engineered NPs, including carbon-based NPs, such as copper NPs, copper oxide nanoparticles, and aluminium NPs, where aluminium NPs and copper NPs have been created, described, and tested for biological activity. The advancement of DNA nanotechnologies and the production of a wide range of various DNA-based NPs has also had a considerable impact on the domains in healthcare and biological processes, such as the delivery of drugs and the development of biological sensors and biochips as well.
Liposomes, liquid crystals, cyclodextrins, micelles, dendrimers, polymerosomes, hydrogels, and nanoparticles are among the most widely used drug delivery mechanisms. Amongst all types of noble metal-based nanoparticles, gold nanoparticles possess a significant ability for use as potential drug delivery vehicles. Gold nanoparticles are available in a range of shapes (spherical, cage-like and rod-shape etc.,) and sizes (1-100 nm).11
Nanoparticles can be employed to improve picture contrast and sensitivity when imaging in vitro. Utilizing nanoparticles by dye-doping, or modulating their ability to absorb a particular dye, is one method. The pattern of distribution of superparamagnetic nanoparticles of iron oxide in biological tissues is the only focus of the new topographic imaging method known as magnetic particle imaging, which also offers great sensitivity, spatial resolution, and contrast. It can be utilized for stem cell labelling and tracking, vascular imaging, and cancer targeting.12
ZINC OXIDE NANOPARTICLES
Zinc has been a potent reducing substance and a comparatively active metal. Zinc’s easy oxidation and production of zinc oxide in response to its potential for reduction is very advantageous for the production of ZnO NPs.
PREPARATION METHODS OF ZINC OXIDE NANOPARTICLES
Sol-gel method
The sol-gel process involves forming a colloidal mixture that transforms into a gel and solid materials through hydrolysis, water retention, and polymerization steps as shown in Figure 1. Common precursors are metal alkoxide or chlorides in organic or aqueous media, typically alcohol. Zinc acetate hydrate in alcohol is a frequently used precursor for zinc nanoparticles. Factors such as the type of alkyl group, solvent, species proportions, temperature, and water-to-alkoxide ratio affect the creation of ZnO NPs. Created by Spanhel and Meulenkamp, the sol-gel method offers advantages like faster initiation and development and the ability to create nano powders in various forms. However, its disadvantage is the high cost of materials used.
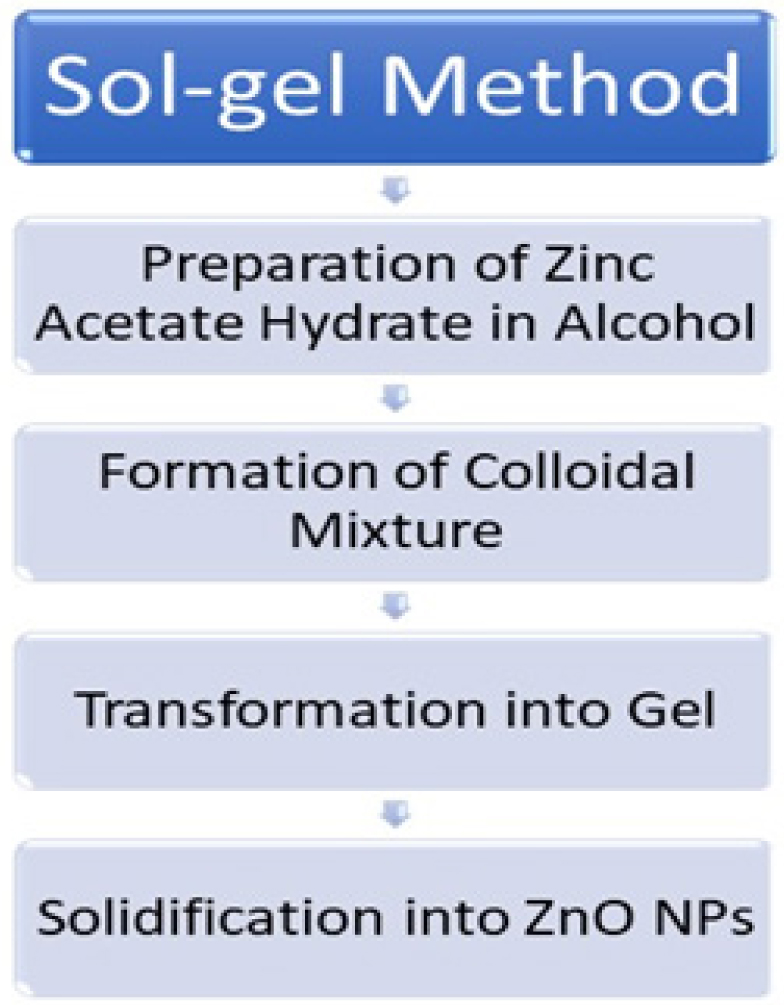
Figure 1.
Preparation of Zinc Using Sol-gel method.
Property | Potential Benefits |
---|---|
Antioxidant | Reduces oxidative stress. |
Antibacterial | Prevents infections. |
Anti-diabetic | Regulates blood sugar levels. |
Anticancer | Inhibits cancer cell growth. |
Neurotransmitter Modulation | Reduces anxiety and seizures. |
Precipitation
The precipitation method effectively produces various zinc oxide nanostructures. This method involves reacting Zinc Salts like Zn(NO3)2, ZnSO4.7H2O, and Zn(CH3COO)2 with basic solutions such as lithium hydroxide, ammonium hydroxide, and sodium hydroxide. The synthesis starts with the reaction between zinc and hydroxide ions, followed by aggregation. Typically, a stable colloidal suspension of ZnO NPs is formed in an alcohol mixture from Zn(OH)2 derived from aqueous solutions. By adjusting factors like solution concentration, pH, washout medium, and calcination temperature, it is possible to create ZnO NPs with various morphologies as mentioned in Figure 2.13
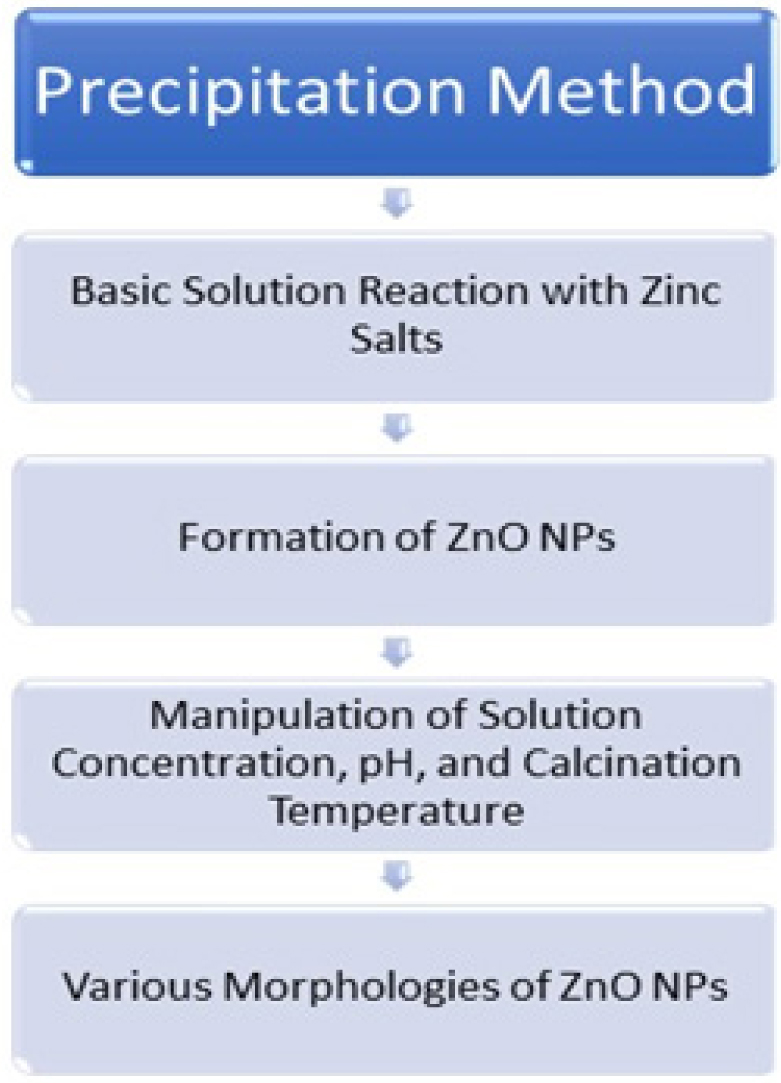
Figure 2.
Preparation of Zinc Using Precipitation method.
Characterization
The human body depends on zinc which is important for a variety of processes, including the functioning of genes, enzymatic activity, synthesis of proteins, cognition, memory, and immunological responses.14 Zinc (Zn) and its Oxide (ZnO) rank among the most intriguing and promising metallic nanostructures. The surface charge, dimension, shape and alterations of nanoparticles, as well as the sample’s purity, are all utilized to characterize them and anticipate their final attributes.
Spectroscopy and Microscopy methods
Thermal Analysis
Using a Mettler-3000 thermal analyzer, (An instrument used for thermal analysis, which involves studying the properties of materials as they change with temperature) thermogravimetric analysis was conducted on a 2 mg sample heated at a rate of 10°C/min in a controlled environment. Additionally, differential thermal analysis was performed over a similar temperature range using a Q200 Differential Scanning Calorimeter (It is an analytical instrument used to measure the heat flow associated with material transitions as a function of temperature and time. It helps in determining thermal properties such as melting points, glass transition temperatures, crystallization, and thermal stability of materials).15
MECHANISMS OF CONVULSIONS AND EPILEPSY
Seizures can happen when networkings are harmed by anatomical, viral, or metabolic issues or when there is inaccurate synchronized neuronal firing in a specific area of the brain or throughout the entire brain. Children’s seizures are most frequently brought on by genetic, perinatal, and cortical development issues. Without a hereditary predisposition to epilepsy, brain tumors, traumatic brain damage, and meningitis/encephalitis are typical causes of seizures in people.16
One may imagine that during a seizure, the brain’s normal ratio of Inhibition (I) to Excitation (E) is thrown off. This E/I unbalance can result from a shift in the way the brain works, including changes in genes, subcellular signalling cascades, and intricate neuronal networks. The E/I balance can be impacted by acquired as well as genetic factors. In addition to defective GABA receptor sub components in Angelman syndrome, aberrant ionic channel activity in benign familial newborn epilepsy, and faulty synaptic connections in cortical dysplasia, there are numerous other genetic diseases that can result in epilepsy. Acquired cerebral insults can alter a circuit’s functionality similarly to how traumatic brain damage can alter the hippocampus network’s structure.17
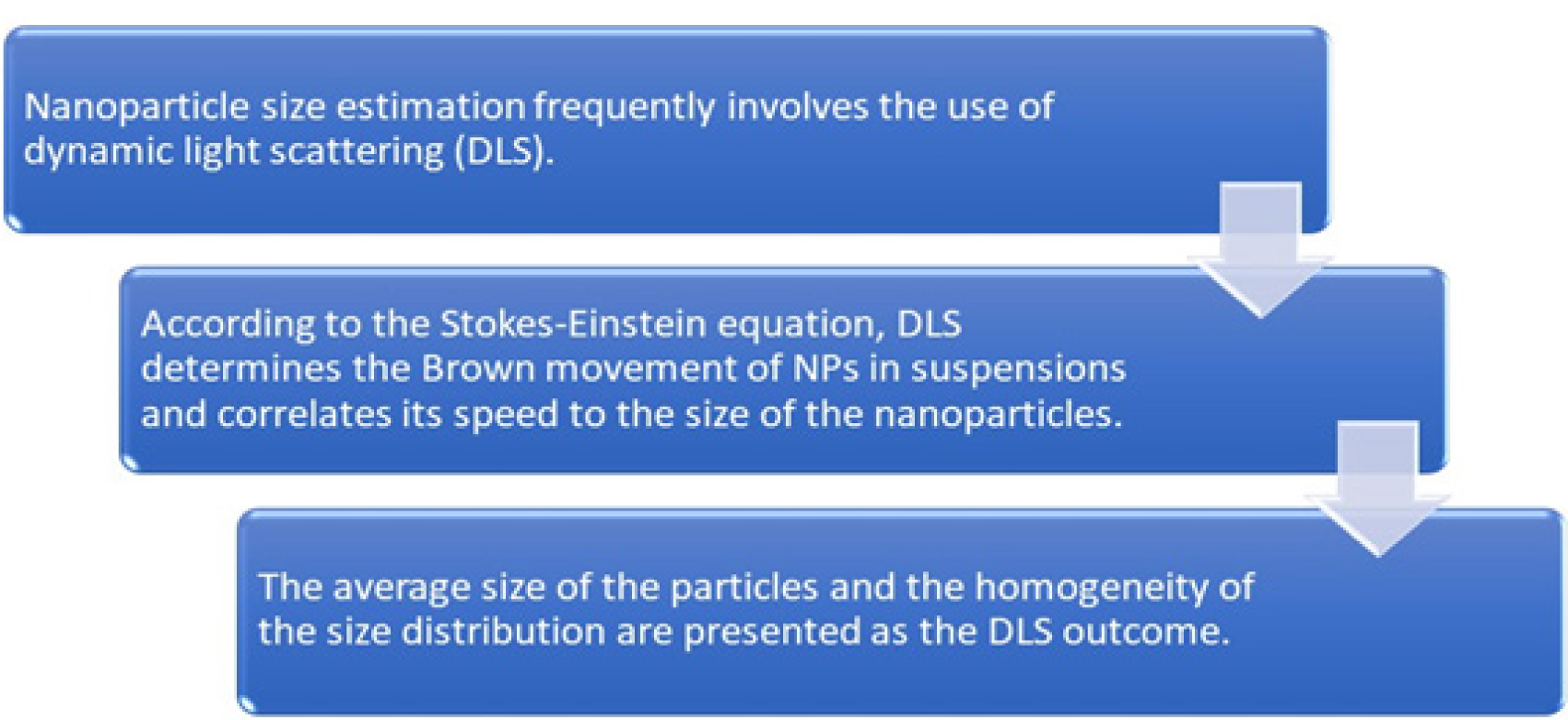
The primary neurotransmitters involved in the pathology of this equilibrium are glutamate and Gamma-Aminobutyric Acid (GABA), and aberrant changes in these molecules have the potential to cause irreparable neuronal damage. The autonomic nervous system’s primary stimulant, acetylcholine, regulates signal transmission across the cholinergic and nicotinic receptors, respectively. A growing body of research suggests that the pathogenesis of epilepsy may be profoundly influenced by the malfunctioning of the nicotinic Acetylcholine receptors, that are abundantly expressed in hippocampus and cortical neurons. Serotonin, melatonin norepinephrine, and histamine can all function as both hormones and neurotransmitters, and the dopamine-epinephrine-norepinephrine cycle stimulates these pathways. According to recent studies, nitric oxide stimulates neuronal transmission to mediate cognitive and memory-related activities.18
Action potentials are generated and propagated by voltage-gated sodium channels. These specialized proteins assist passage down an electrochemical gradient, bringing the membrane closer to the sodium equilibrium potential by momentarily boosting membrane permeability to sodium ions. Voltage-gated sodium channels play a crucial role in central nervous system in controlling excitability of neurons and consequently network activity. They are uniformly expressed in all the compartments of central nervous system, with a higher density at the Ranvier nodes and axon beginning segments.19
POTENTIAL OF ZINC OXIDE NANOPARTICLES AS ANTI-CONVULSANT
The variety and adaptability of Nanoparticles may help to more effectively advance therapeutic and diagnostic methods for the treatment of different neurological illnesses. Through a variety of mechanisms, such as the improvement of drug delivery through the Blood Brain Barrier, promotion of pharmacokinetics and drug biological distribution, provision of more effective drugs, and advancement of targeted drug delivery systems.20 Nanoparticle-based therapies could achieve the greatest therapeutic effects for various brain diseases. For the supply of a diverse range of innovative medications, several nano formulations have been investigated as prospective drug carriers to aid in the treatment of various acute or chronic neurological illnesses, such as ischemic stroke, epilepsy.21 It’s crucial to identify brain degenerative alterations as early as possible, quickly, and accurately in a number of neurological illnesses, including epilepsy. The distinctive qualities of different Nanoparticles, such as their high avidity, multiplexity, and manipulation potential, improve the sensitivity, accuracy, specificity, resolution, and contrast of tools for diagnosis for diverse neurological illnesses.22
Zinc has been shown to slow the progression of neurodegenerative disorders and shield the blood-brain barrier from oxidative damage.14 Zinc is necessary for living, and lacking it is associated with a number of harmful disorders, such as immune system impairment, reduced skin as well as bone development and repair, and dysfunction of cognitive.23 Zinc may have furthermore, distinct signalling functions inside the central nervous system, according to finding that the metal is present in synaptic vesicles. Importantly, sensory experience can quickly alter the location and composition of this vesicular or synaptic zinc pool, indicating that neuronal Zinc signalling is subject to experience dependent plasticity. Both the extracellular as well as intracellular labile zinc concentrations are kept at nanomolar or subnanomolar levels while the cell is at rest. Extracellular zinc levels briefly rise after synaptic zinc is released, modifying neurotransmission. In addition, activity can cause significant temporary increases in intracellular zinc levels that start a variety of cellular signals.24 To avoid neuronal death that results from zinc dysregulation, these fluctuations in Zn concentration within as well as outside of the cells are strictly regulated during physiological settings.
According to several researches, ZnO NP have a diverse range of biological functions against many diseases, notably neurological problems. They have antioxidant, antibacterial, anti-diabetic, and anticancer properties. In the striatum, auditory brainstem, cerebral cortex, and hippocampal synaptic vesicles of glutamatergic neuron populations. Zinc is a metal that is required for neuromodulation in biology. One part of the brain that is important for memory and learning is the hippocampus. N-methyl-D-aspartate, sometimes known as NMDA, is involved with both seizures and depression. The presynaptic area’s release of zinc and glutamate limits the function of NMDA receptors in a number of ways. It decreases glutamate release via presynaptic neurons on the hippocampus by increasing GABA synthesis and exocytosis.
GABA and NMDA receptors are modulated by zinc buildup in synaptic vesicles as well as its release through glutamatergic neurons to postsynaptic neurons. Both receptors are regarded as crucial elements in the development of anxious behaviours. Anxiety is induced by the over secretion of glutamate, which results in NMDA hyperactivation. Zinc treatment may reduce anxiety. In addition, in the case of anxiety, zinc can increase GABA secretion in the hippocampus, which inhibits presynaptic glutamate release. Therefore, it might be hypothesized that administering ZnO NPs may have controlled those mentioned pathways, improving memory and learning while reducing anxiety and seizures.25
Zinc oxide has several beneficial features and holds great potential in a number of medical fields. Table 1 represents the potential benefits and their medical properties.26
MECHANISM OF ACTION OF ZINC OXIDE NANOPARTICLES IN NEURODEGENERATIVE DISEASE
According to several researches, ZnO NP have a diverse range of biological applications against many diseases, notably neurodegenerative disorders. In the striatum, auditory brainstem, cerebral cortex, and hippocampal synaptic vesicles of glutamatergic neuron populations, Zinc is a neuromodulator metal that is essential for life. One part of the brain that is important for learning and retention of information in the hippocampus. N-methyl-D-aspartate, sometimes known as NMDA, is involved in both epileptic and depressive disorders. The presynaptic area’s release of zinc and glutamate limits the function of NMDA receptors in a number of ways. It decreases glutamate release via presynaptic neurons within the hippocampus by increasing GABA synthesis and exocytosis.25 which reduces the neuronal firing and reduces the occurrence of seizures.
SAFETY AND BIOCOMPATIBILITY
Cancer treatment
ZnO NPs are suggested as effective cancer therapeutic agents. Cancer cells are more sensitive to ZnO NPs toxicity compared to normal cells. At physiological pH, ZnO NPs have a significant positive charge, which interacts with the negatively charged molecules on cancer cell surfaces. This interaction helps prevent cell proliferation at tumor sites. Additionally, cancer cells exhibit increased toxicity and apoptosis when exposed to ZnO NPs due to their heightened sensitivity to Reactive Oxygen Species (ROS).27
Bioimaging
ZnO NPs are a dependable choice when looking for a practical and affordable instrument for bioimaging. In the near UV, blue, and green areas, ZnO NPs exhibit inherent fluorescence characteristics with excitonic emission. ZnO NPs show high stability and less photobleaching than the other fluorescent probes.28 By functionalizing ZnO NPs with the right receptor molecules, they can be used to image bacteria and cancer cells.29
Gene delivery
Gene therapy holds great promise for treating life-threatening conditions like cancer and neurodegeneration. ZnO NPs are being studied as gene delivery agents. One major challenge in gene therapy is creating a vector that securely transports DNA to the target area. Modified ZnO NPs, shaped like tetrapods similar to phage vectors, can efficiently deliver plasmid DNA into cells, addressing this challenge.30,31
Neurobiological application
ZnO NPs are used to detect neurotransmitters in the brain. Zhao et al. demonstrated the use of luminous ZnO NPs capped with (3-aminopropyl) triethoxysilane for detecting dopamine. ZnO NP-based sensors are proposed for identifying both acetylcholine and dopamine. Additionally, Zinc (Zn2+) is administered into the brain using ZnO NP nano formulations for treating depression and anxiety disorders; Figure 4 highlights various other biomedical applications.32
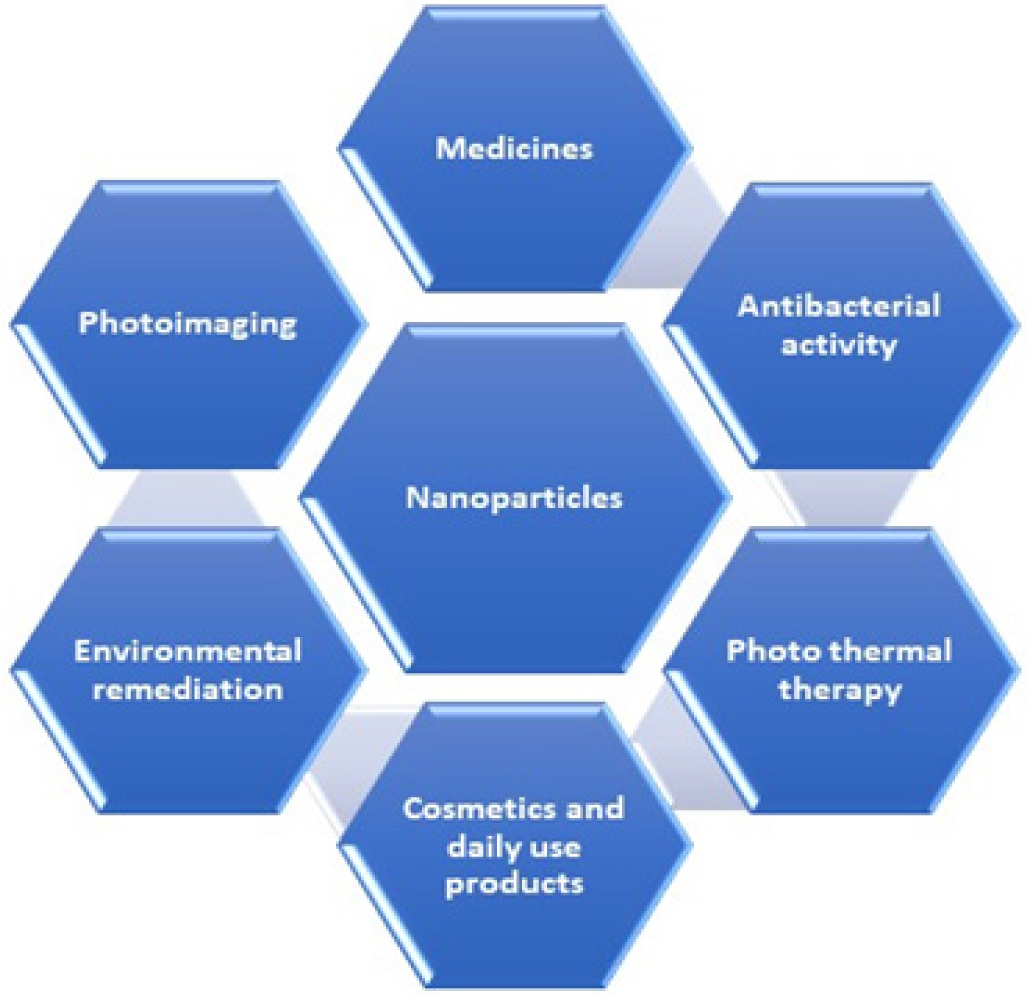
Figure 4.
Biomedical Applications of zinc oxide Nanoparticles.
ZnO NPs have been extensively investigated to determine their effects on a variety of cell lines. The toxicity experiments of ZnO NPs under in vitro conditions are demonstrated in Table 2, which illustrates cytotoxicity, genotoxicity, and inflammation at varying concentrations and incubation periods. These discoveries offer valuable insights into the potential effects of ZnO NPs on cellular health.
Sl. No. | Cell Lines | Incubation | Concentration | Effects | References |
---|---|---|---|---|---|
1 | Epidermal cell line of human | 24 hr | 0.8 as well as 14 lg/ mL. | Cytotoxicity found in (14 lg/mL, 24 hr). | Subramaniam et al.,33 |
2 | Human heparinized blood samples | 72 hr | 500 parts per million. | Genotoxicity found in (500 parts per million, 72 hr). | Akbaba and Turkez et al. ,34 |
3 | Eosinophils of human (HEPES-P/S) | 30 min, 3 hr, 24 hr and 48 hr. | 100 lg/mL | Inflammation of Eosinophilic found in (100 lg/mL, 48 hr). | Silva and girard et al.,33 |
4 | Cell line of Mouse microglial, | 24 hr | 6.6 lg/mL | Due to Activate microglia were induction of neuroinflammation found in (6.6 lg/mL, 24 hr). | Sharma et al. ,35 |
5 | Cell line of Mice macrophage (RAW 264.7) | 24 hr | 15, 50, 150 as well as 300 lg/mL. | Inflammation found in (300 lg/mL, 24 hr). | Uski et al. ,36 |
CONCLUSION
ZnO NPs exhibit significant anti-convulsant properties, making them a promising option for epilepsy treatment. Their unique physicochemical traits, including a large surface area and
intrinsic antioxidant capabilities, allow ZnO NPs to inhibit oxidative stress, a key factor in epileptogenesis. Experimental studies show that ZnO NPs can decrease Reactive Oxygen Species (ROS) levels and protect against neuronal damage. By modulating neurotransmitter release, including glutamate and GABA, ZnO NPs help maintain the brain’s excitatory-inhibitory balance, potentially reducing seizures. The nanoscale size of ZnO NPs enables them to cross the blood-brain barrier effectively, enhancing the bioavailability and efficacy of traditional anti-convulsant drugs. Additionally, ZnO NPs regulate calcium influx and preserve neuronal membrane integrity, providing neuroprotection against excitotoxicity in seizure conditions. They also serve as drug delivery carriers, increasing the bioavailability of standard anti-epileptic medications and potentially improving treatment outcomes while minimizing side effects.
In conclusion, ZnO NPs hold significant promise as an adjunct therapy for epilepsy, offering neuroprotection, enhanced drug delivery, and neurotransmission modulation, making them a valuable addition to epilepsy treatment options.
Cite this article:
Aishwarya V, Bharathi DR, Chandan K, Jayanth BM, Ahmed SS. Zinc Oxide Nanoparticles for their Anti-Convulsant Property: A Comprehensive Review. J Young Pharm. 2025;17(1):46-53.
ACKNOWLEDGEMENT
The authors would like to express their gratitude to the Department of Pharmacology, Sri Adichunchanagiri College of Pharmacy, Adichunchanagiri University, located in B.G. Nagar, Karnataka, India, for their support and encouragement during the preparation and submission of this article. The scholarly environment provided by the institution has greatly contributed to the development of this research.
ABBREVIATIONS
ZnO NPs | Zinc Oxide Nanoparticles |
---|---|
NPs | Nanoparticles |
GABA | Gamma-aminobutyric acid |
ROS | Reactive Oxygen Species |
PHT | Phenytoin |
PB | Phenobarbital |
PRD | Primidone |
VBG | Vigabatrin |
CuO NPs | Copper Oxide Nanoparticles |
Au NPs | Gold Nanoparticles |
MPI | Magnetic Particle Imaging |
References
- Beghi E. The epidemiology of epilepsy. Neuroepidemiology. 2020;54(2):185-91. [PubMed] | [CrossRef] | [Google Scholar]
- Jehi L. The epileptogenic zone: concept and definition. Epilepsy Curr. 2018;18(1):12-6. [PubMed] | [CrossRef] | [Google Scholar]
- Zhu L, Chen L, Xu P, Lu D, Dai S, Zhong L, et al. Genetic and molecular basis of epilepsy-related cognitive dysfunction. Epilepsy Behav. 2020;104(A):106848 [PubMed] | [CrossRef] | [Google Scholar]
- Landmark CJ, Johannessen SI. Modifications of antiepileptic drugs for improved tolerability and efficacy. Perspect Med Chem. 2008;2:21-39. 1177391X0800200 [PubMed] | [CrossRef] | [Google Scholar]
- Silva GA. Introduction to nanotechnology and its applications to medicine. Surg Neurol. 2004;61(3):216-20. [PubMed] | [CrossRef] | [Google Scholar]
- Fariq A, Khan T, Yasmin A. Microbial synthesis of nanoparticles and their potential applications in biomedicine. J Appl Biomed. 2017;15(4):241-8. [CrossRef] | [Google Scholar]
- Farahani HN, Ashthiani AR, Masihi MS. Study on serum zinc and selenium levels in epileptic patients. Neurosciences (Riyadh). 2013;18(2):138-42. [PubMed] | [Google Scholar]
- S S, Ss A, S SB, Hk V, Pv M. Determination of the bioavailability of zinc oxide nanoparticles using ICP-AES and associated toxicity. Colloids Surf B Biointerfaces. 2020;188:110767 [PubMed] | [CrossRef] | [Google Scholar]
- Daraee H, Eatemadi A, Abbasi E, Fekri Aval S, Kouhi M, Akbarzadeh A, et al. Application of gold nanoparticles in biomedical and drug delivery. Artif Cells Nanomed Biotechnol. 2016;44(1):410-22. [PubMed] | [CrossRef] | [Google Scholar]
- Jafernik K, Ładniak A, Blicharska E, Czarnek K, Ekiert H, Wiącek AE, et al. Chitosan-based nanoparticles as effective drug delivery systems—a review. Molecules. 2023;28(4):1963 [PubMed] | [CrossRef] | [Google Scholar]
- Rudramurthy GR, Swamy MK. Potential applications of engineered nanoparticles in medicine and biology: an update. J Biol Inorg Chem. 2018;23(8):1185-204. [PubMed] | [CrossRef] | [Google Scholar]
- Hoshyar N, Gray S, Han H, Bao G. The effect of nanoparticle size on pharmacokinetics and cellular interaction. Nanomedicine (Lond). 2016;11(6):673-92. [PubMed] | [CrossRef] | [Google Scholar]
- Król A, Pomastowski P, Rafińska K, Railean-Plugaru V, Buszewski B. Zinc oxide nanoparticles: synthesis, antiseptic activity and toxicity mechanism. Adv Colloid Interface Sci. 2017;249:37-52. [PubMed] | [CrossRef] | [Google Scholar]
- Keerthana S, Kumar A. Potential risks and benefits of zinc oxide nanoparticles: a systematic review. Crit Rev Toxicol. 2020;50(1):47-71. [PubMed] | [CrossRef] | [Google Scholar]
- Ansari SA, Husain Q, Qayyum S, Azam A. Designing and surface modification of zinc oxide nanoparticles for biomedical applications. Food Chem Toxicol. 2011;49(9):2107-15. [PubMed] | [CrossRef] | [Google Scholar]
- Cloyd T, Haussner D, Winograd SM. Definitions and classifications. 2024 [PubMed] | [CrossRef] | [Google Scholar]
- Stafstrom CE, Carmant L. Seizures and epilepsy: an overview for neuroscientists. Cold Spring Harb Perspect Med. 2015;5(6):a022426 [PubMed] | [CrossRef] | [Google Scholar]
- Akyuz E, Polat AK, Eroglu E, Kullu I, Angelopoulou E, Paudel YN, et al. Revisiting the role of neurotransmitters in epilepsy: an updated review. Life Sci. 2021;265:118826 [PubMed] | [CrossRef] | [Google Scholar]
- Kaplan DI, Isom LL, Petrou S. Role of sodium channels in epilepsy. Cold Spring Harb Perspect Med. 2016;6(6):a022814 [PubMed] | [CrossRef] | [Google Scholar]
- Bonilla L, Esteruelas G, Ettcheto M, Espina M, García ML, Camins A, et al. Biodegradable nanoparticles for the treatment of epilepsy: from current advances to future challenges. Epilepsia Open. 2022(7 Suppl 1):S121-32. [PubMed] | [CrossRef] | [Google Scholar]
- Pottoo FH, Sharma S, Javed MN, Barkat MdA, Harshita , Alam MdS, et al. Lipid-based nanoformulations in the treatment of neurological disorders. Drug Metab Rev. 2020;52(1):185-204. [PubMed] | [CrossRef] | [Google Scholar]
- Sepasi T, Ghadiri T, Bani F, Ebrahimi-Kalan A, Khodakarimi S, Zarebkohan A, et al. Nanotechnology-based approaches in diagnosis and treatment of epilepsy. J Nanopart Res. 2022;24(10):199 [CrossRef] | [Google Scholar]
- Prasad AS. Zinc deficiency. BMJ. 2003;326(7386):409-10. [PubMed] | [CrossRef] | [Google Scholar]
- Sensi SL, Paoletti P, Bush AI, Sekler I. Zinc in the physiology and pathology of the CNS. Nat Rev Neurosci. 2009;10(11):780-91. [PubMed] | [CrossRef] | [Google Scholar]
- Krall RF, Tzounopoulos T, Aizenman E. The function and regulation of zinc in the brain. Neuroscience. 2021;457:235-58. [PubMed] | [CrossRef] | [Google Scholar]
- Fatima K, Asif M, Farooq U, Gilani SJ, Bin Jumah MN, Ahmed MM, et al. Antioxidant and anti-inflammatory applications of Aerva persica aqueous-root extract-mediated synthesis of ZnO nanoparticles. ACS Omega. 2024;9(14):15882-92. [PubMed] | [CrossRef] | [Google Scholar]
- Rasmussen JW, Martinez E, Louka P, Wingett DG. Zinc oxide nanoparticles for selective destruction of tumor cells and potential for drug delivery applications. Expert Opin Drug Deliv. 2010;7(9):1063-77. [PubMed] | [CrossRef] | [Google Scholar]
- Wu YL, Fu S, Tok AI, Zeng XT, Lim CS, Kwek LC, et al. A dual-colored bio-marker made of doped ZnO nanocrystals. Nanotechnology. 2008;19(34):345605 [PubMed] | [CrossRef] | [Google Scholar]
- Sudhagar S, Sathya S, Pandian K, Lakshmi BS. Targeting and sensing cancer cells with ZnO Nanoprobes . Biotechnol Lett. 2011;33(9):1891-6. [PubMed] | [CrossRef] | [Google Scholar]
- Zhang P, Liu W. ZnO QD@ PMAA-co-PDMAEMA nonviral vector for plasmid DNA delivery and bioimaging. Biomaterials. 2010;31(11):3087-94. [PubMed] | [CrossRef] | [Google Scholar]
- Nie L, Gao L, Feng P, Zhang J, Fu X, Liu Y, et al. Three‐dimensional functionalized tetrapod‐like ZnO nanostructures for plasmid DNA delivery. Small. 2006;2(5):621-5. [PubMed] | [CrossRef] | [Google Scholar]
- Sruthi S, Ashtami J, Mohanan PV. Biomedical application and hidden toxicity of zinc oxide nanoparticles. Mater Today Chem. 2018;10:175-86. [CrossRef] | [Google Scholar]
- Silva LR, Girard D. Human eosinophils are direct targets to nanoparticles: zinc oxide nanoparticles (ZnO) delay apoptosis and increase the production of the pro-inflammatory cytokines IL-1β and IL-8. Toxicol Lett. 2016;259:11-20. [PubMed] | [CrossRef] | [Google Scholar]
- Akbaba GB, Türkez H. Investigation of the genotoxicity of aluminum oxide, β-Tricalcium Phosphate, and Zinc Oxide Nanoparticles . Int J Toxicol. 2018;37(3):216-22. [PubMed] | [CrossRef] | [Google Scholar]
- Sharma AK, Singh V, Gera R, Purohit MP, Ghosh D. Zinc oxide nanoparticle induces microglial death by NADPH-oxidase-independent reactive oxygen species as well as energy depletion. Mol Neurobiol. 2017;54(8):6273-86. [PubMed] | [CrossRef] | [Google Scholar]
- Uski O, Torvela T, Sippula O, Karhunen T, Koponen H, Peräniemi S, et al. Array. Toxicol . 2017;42:105-13. [PubMed] | [CrossRef] | [Google Scholar]