ABSTRACT
Background
A proficient hydroponic system for the cultivation of Rhinacanthus nasutus has been implemented with the aim of augmenting rhinacanthin production. Elicitation stands out as a viable technique for bolstering the yield of rhinacanthin.
Materials and Methods
A hydroponics system of R. nasutus was treated with six elicitors, namely chitosan, Trichoderma harzianum extract, sodium alginate, lawsone, methyl jasmonate, and salicylic acid at three concentrations. A suitable elicitation period for the selected elicitor was also determined. The contents of rhinacanthin-C, -D, and -N accumulated were determined using an HPLC method.
Results
Amongst these, chitosan (0.15 mg/mL) and T harzianum extract (1.0 mg/mL) significantly enhanced rhinacanthin production and gave the highest total rhinacanthin content, up to 6.0-6.1% w/w, which was 2.2-fold higher than the untreated roots (2.7% w/w). In addition, lawsone (3.0 μM) and salicylic acid (100 μM) significantly increased rhinacanthin production up to 4.6% and 3.4% w/w, respectively, while neither methyl jasmonate nor sodium alginate increased rhinacanthin production. The suitable elicitor-contact periods for chitosan, T harzianum extract, and lawsone were 48, 24, and 72 h, respectively. Moreover, the leaves harvested from these optimized conditions also contained markedly high contents of rhinacanthins, up to 5.4%, 6.7%, and 5.1% w/w, respectively.
Conclusion
The present study established the optimized elicitation methods for the R. nasutus hydroponics system using chitosan, T harzianum extract, and lawsone as elicitors to obtain the high-rhinacanthin-producing roots and leaves. These hydroponics and elicitation systems might be alternative sources of the high-quality R. nasutus roots and leaves.
INTRODUCTION
Plants contain a wide range of secondary metabolites, such as alkaloids, terpenoids, sterols, flavonoids, coumarin, and quinones, which usually possess therapeutic properties against the causes of fatal ailments such as diabetes, cancer, infectious, musculoskeletal, and cardiovascular diseases. However, the production of secondary metabolites in plants is definitely influenced by environmental variations, which markedly affect the consistency of therapeutic efficacy of herbal medicines. Rhinacanthins (Figure 1), namely rhinacanthin-C (RC), -D (RD), and -N (RN) are the major bioactive naphthoquinone esters mainly accumulated in the roots and leaves of Rhinacanthus nasutus (L.) Kurz.1 These naphthoquinones have been reported as the active constituents of R. nasutus for the treatments of various diseases associated with their antifungal,2 antibacterial,3 anti-inflammatory,4 anti-allergic,5 anti-tumor,6 anti-Alzheimer,7 anti-Parkinson,8 hypoglycemic and hypolipidemic9–12 activities. Although R. nasutus roots and leaves are the major sources of rhinacanthins, most of the raw materials commercially supplied for R. nasutus-based herbal products are the whole aerial parts, which contain a low content of rhinacanthins, thus leading to low-quality products.
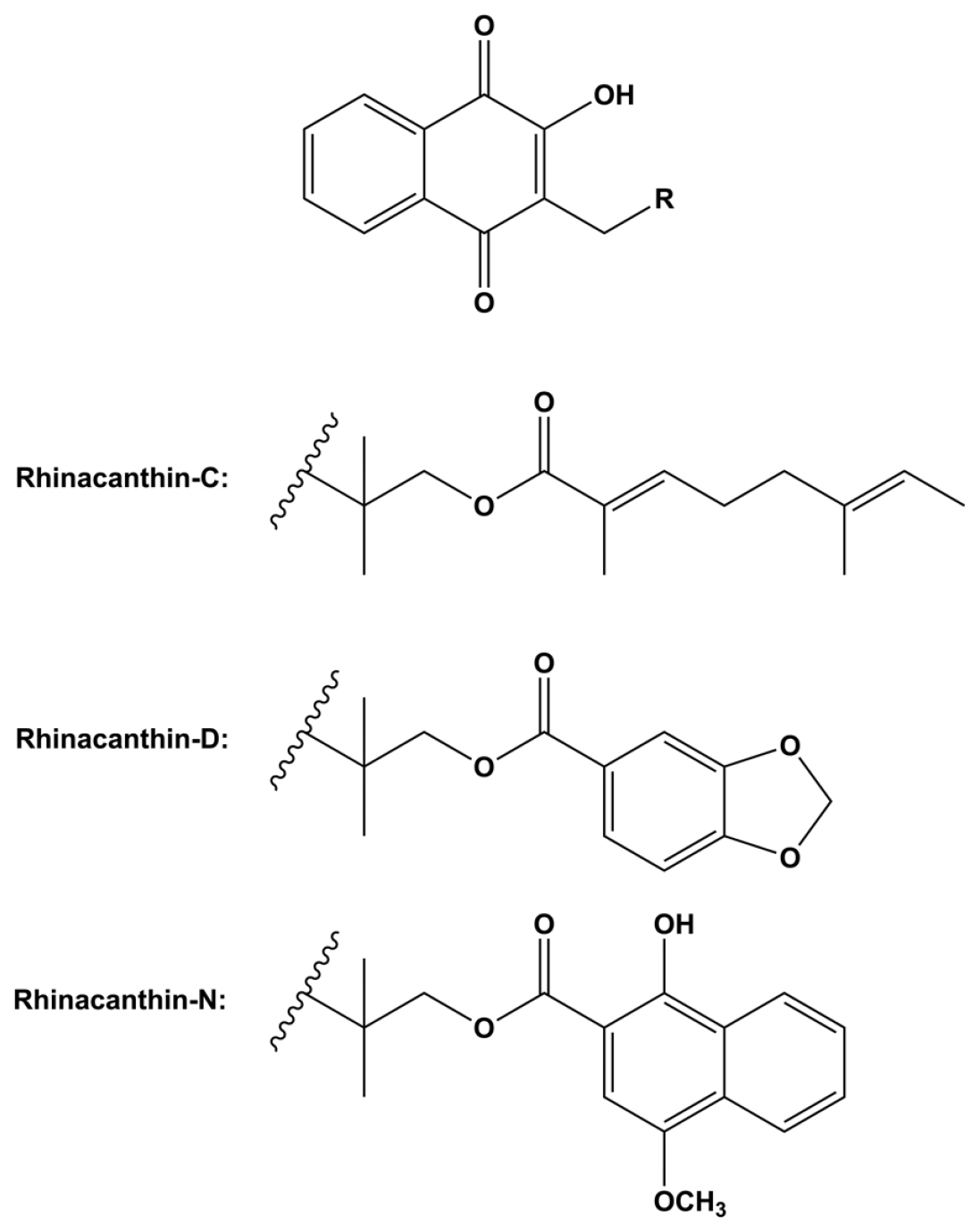
Figure 1:
Chemical structures of rhinacanthin-C, rhinacanthin-D, and rhinacanthin-N.
Several efforts are being made toward the establishment of an in-house cultivation system, i.e., a hydroponics system, in which the growth conditions can be easily controlled, in order to enhance secondary metabolite production in plants. Recently, a hydroponics system for R. nasutus has been established to overcome the limitations of commercially available R. nasutus roots. This hydroponics system produced high dried root biomass (0.25 g/plant) with a high rhinacanthin content (3.3% w/w) in a short period of cultivation. However, the content of rhinacanthin was rather lower than that of the natural roots (3.4-4.1% w/w). The quality of R. nasutus roots certainly depends on the content of rhinacanthins. Fortunately, an elicitation technique could be easily applied with a hydroponics system to enhance phytoalexin production. Therefore, the present study puts an emphasis on increasing rhinacanthin production in the hydroponics system of R. nasutus using an elicitation technique. Both biotic and biotic elicitors, including chitosan, T. harzianum extract, sodium alginate, lawsone, methyl jasmonate, and salicylic acid, were determined for their biosynthetic-stimulating properties at different concentrations. The selected elicitors were subjected to further optimization for the periods of the elicitor contract to enhance rhinacanthin production in the hydroponics system of R. nasutus.
MATERIALS AND METHODS
Chemicals
Murashige and Skoog (MS) Basal Salt Mixture M524 was obtained from Phytotechlab (KS, USA). The authentic rhinacanthins (RC, RD, and RN) were previously purified.12 Methanol (HPLC grade), ethyl acetate (analytical grade), and ethanol (analytical grade) were purchased from Labscan Asia (Bangkok, Thailand). Water was purified by a Milli-Q system (Millipore, Bedford, MA, USA). Chitosan (Toronto Research Chemicals, Canada), methyl jasmonate, sodium alginate, salicylic acid, and lawsone (Sigma-Aldrich, USA) were analytical grade. Trichoderma harzianum extract was obtained from iLab SV Biotech (Bangkok, Thailand). Other chemicals used were analytical grade and purchased from Sigma (St. Louis, MO) or MERCK (Darmstadt, Germany).
Plant materials
R. nasutus shoots were collected from two-year-old plants grown in the Botanical Garden of the Faculty of Pharmaceutical Sciences, Prince of Songkla University, in 2021. The plant was identified by Pharkphoom Panichayupakaranant, and the voucher specimen (no. 001 18 14) was deposited at the Faculty of Pharmaceutical Sciences, Prince of Songkla University, Thailand.
Establishment and maintenance of the hydroponics system
The shoots of R. nasutus were washed with tap water and subsequently soaked in a 2% sodium hypochlorite solution (15 min), and then washed with deionized water. The shoots were subsequently transferred to a growing container for root initiation (20 plants/container). The roots were initiated in deionized water (8 L) to produce the plantlets. The hydroponics conditions of R. nasutus were as follows: the plantlets were grown in 20% MS liquid medium (8 L) supplied with aeration (4.5 L/ min) and was cultivated at 25±2°C under LED white light (230 μmol/s/m2) for 16 h/day. The solution of 20% MS medium was periodically added every 4 weeks. Root and leaf harvesting could be performed every 12 weeks.
Harvesting and extraction
After the treatments, the roots or leaves (in some cases) were cut and washed with tap water and deionized water, respectively. The plant samples were dried in a hot air oven at 60°C for 24 h, then ground and passed through a no. 20 sieve. The sample powders (100 mg) were extracted with 50 mL of ethyl acetate using a microwave-assisted extraction. The extraction conditions were as follows: microwave frequency and power of 2,450 MHz and 450 watts, respectively; 3 irradiation cycles (1 cycle=45 sec power-on and 30 sec power-off); a final extraction temperature of 72±2°C. The extracts were filtered and evaporated to dryness using a rotary evaporator at 40°C.
Quantitative HPLC method
HPLC conditions
Quantitative HPLC analysis was carried out using the method previously described2 with minor modifications. Briefly, the method was performed using a Shimadzu HPLC system (Model LC-20, Shimadzu, Tokyo, Japan) equipped with a LC-20AD pump, an SPD-M20A photodiode-array detector, and an SIL-20A autosampler. A 150 mm x 4.6 mm Phenomenex ODS column was eluted with a mobile phase of methanol and 5% aqueous acetic acid (80:20, v/v) at a flow rate of 1 mL/min. The quantitative UV detection was set at 254 nm.
Calibration curve
The calibration curves were established from the authentic RC (12.5-200 μg/mL), RD (3.12-50 μg/mL), and RN (3.12-50 μg/mL), respectively. The linear equations of y=37966x + 21418 (r2=0.9999), and y=95934x-33213 (r2=0.9999), and y=150297x-86082 (r2=0.9997) were established as the calibration curves of RC, RD, and RN, respectively.
Sample preparation
The dried extracts were reconstituted with methanol (10 mL) in a volumetric flask and then filtered through a 0.45 μm membrane filter. All experiments were carried out in triplicate.
Preparation of elicitors
The stock solutions of elicitors, including chitosan, T. harzianum extract, sodium alginate, and lawsone, were prepared in deionized water, while those of methyl jasmonate and salicylic acid were prepared in 50% v/v ethanol.
Effect of elicitor type and concentration
R. nasutus hydroponics plants (12-week-old) were treated with each elicitor at three different concentrations (Table 1) for 48 h. The control groups were treated with an equal amount of the solvent used for each elicitor (water or ethanol). After the treatments, the roots were harvested and subjected to the drying process. The dry weights of roots were determined using an analytical balance, and their HPLC determinations of RC, RD, and RN contents were carried out.
Elicitors and Concentrations | Dried root biomass (mg/ plant) | Rhinacanthin content* (% w/w) | |||
---|---|---|---|---|---|
RC | RD | RN | Total | ||
Control | 125.26±4.04a | 2.18±0.16a | 0.24±0.02a | 0.30±0.01a | 2.72±0.20a |
Chitosan | |||||
0.05 mg/mL | 126.34±6.10a | 3.96±0.18b | 0.39±0.01b | 0.53±0.02b | 4.89±0.20b |
0.15 mg/mL | 123.31±8.26a | 4.96±0.27c | 0.48±0.01c | 0.67±0.02c | 6.10±0.09c |
0.25 mg/mL | 116.64±3.47a | 2.78±0.03d | 0.28±0.01ad | 0.38±0.01a | 3.44±0.03d |
T. harzianum extract | |||||
1.0 mg/mL | 134.45±6.78a | 5.53±0.10e | 0.29±0.03d | 0.22±0.01d | 6.04±0.12c |
2.0 mg/mL | 123.39±3.28a | 4.10±0.11b | 0.20±0.01e | 0.18±0.01d | 4.48±0.12b |
3.0 mg/mL | 124.32±4.65a | 4.16±0.63b | 0.18±0.03ef | 0.17±0.03d | 4.50±0.69b |
Lawsone | |||||
1.5 μM | 137.43±4.57a | 3.10±0.02g | 0.19±0.01e | 0.40±0.01bc | 3.69±0.02d |
3.0 μM | 133.79±13.51a | 3.96±0.18b | 0.21±0.00ae | 0.46±0.02bc | 4.63±0.24b |
6.0 μM | 126.14±7.32a | 3.82±0.25b | 0.22±0.03ae | 0.41±0.03c | 4.45±0.32b |
Salicylic acid | |||||
50 μM | 133.01±8.91a | 2.82±0.03d | 0.11±0.01f | 0.30±0.01a | 3.24±0.04d |
100 μM | 132.70±9.39a | 3.31±0.02g | 0.06±0.01g | 0.08±0.01f | 3.45±0.02d |
150 μM | 121.12±1.54a | 3.19±0.04g | 0.05±0.01g | 0.06±0.01f | 3.31±0.04d |
Sodium alginate | |||||
0.8 mg/mL | 132.04±7.41a | 2.33±0.16a | 0.15±0.02f | 0.12±0.01f | 2.61±0.01a |
1.5 mg/mL | 136.58±8.11a | 2.34±0.27a | 0.09±0.02fg | 0.12±0.03f | 2.54±0.01a |
3.0 mg/mL | 136.11±10.34a | 2.49±0.06a | 0.13±0.01f | 0.05±0.00f | 2.67±0.01a |
Methyl jasmonate | |||||
100 μM | 124.55±2.32a | 2.14±0.06a | 0.25±0.02ad | 0.21±0.01d | 2.60±0.08a |
200 μM | 135.63±3.05a | 2.13±0.15a | 0.26±0.02ad | 0.21±0.01d | 2.60±0.16a |
400 μM | 136.44±7.26a | 1.58±0.06f | 0.21±0.01ae | 0.17±0.01e | 1.96±0.06e |
Effect of elicitor contact time
The hydroponics plants (12 weeks old) were treated with chitosan (0.15 mg/mL), T. harzianum extract (1 mg/mL), and lawsone (6 μM) for 24, 48, and 72 h. Subsequently, the roots were harvested and subjected to the drying process to determine the dry biomass weights. The contents of RC, RD, and RN were determined using the HPLC method.
Statistical analysis
The data was presented as the Mean±Standard Error (SE). Statistical significance was analyzed using a one-way ANOVA, followed by Tukey’s test. By convention, results are considered statistically significant when p<0.05.
RESULTS
Types and concentrations of elicitors, as well as the elicitor contact periods, usually affect the growth and production of phytoalexins in plant tissues.13 Therefore, the present study determined the effects of various elicitors, including chitosan, T. harzianum extract, sodium alginate, lawsone, methyl jasmonate, and salicylic acid at three concentrations, on root biomass and rhinacanthin (RC, RD, and RN) production in R. nasutus hydroponics. The 12 weeks old hydroponics plants were used to treat with the elicitors because they were in the linear growth phase, which actively responded to the elicitor stimulation (result not shown). None of elicitor at all concentrations used in this study exhibited detrimental effects on plant growth and root biomass production compared to the control groups (Table 1). Regarding rhinacanthin production, four elicitors, including chitosan, T. harzianum, lawsone, and salicylic acid, but not methyl jasmonate and sodium alginate, significantly increased rhinacanthin production in the hydroponics plants. In addition, chitosan and T. harzianum extract gave the highest rhinacanthin content up to 6.0-6.1% w/w, followed by lawsone (up to 4.6% w/w) and salicylic acid (up to 3.4% w/w), respectively (Table 1).
Rhinacanthin production was changed by the amounts of chitosan, T. harzianum extract, and lawsone, but not by salicylic acid. The highest amounts of total rhinacanthins were found in chitosan (0.15 mg/mL) and T. harzianum extract (1.0 mg/mL) (Table 1). The results show that chitosan and T. harzianum extract are the best elicitors for increasing rhinacanthin production in R. nasutus hydroponics roots. This is because both elicitors led to 2.2 times more rhinacanthin production than the roots that were not treated (2.7% w/w). Notably, T. harzianum extract exhibited more specifically increased rhinacanthin-C production (5.5% w/w) than the others. This finding is the first report of T. harzianum extract eliciting naphthoquinone production in plants.
The elicitor contact period plays a significant role in phytoalexin biosynthesis. Extending the elicitor contact period might result in either increased metabolic production or physiological damage. As a result, determining an optimal contact period for any elicitor was needed to maximize rhinacanthin production. The top three elicitors that gave the highest boosting power of rhinacanthin production, including chitosan, T. harzianum extract, and lawsone were selected to determine the effect of elicitor contact periods (24, 48, and 72 h) on rhinacanthin production in the roots. The results revealed that an increase in elicitor contact periods up to 72 h did not significantly affect the growth and root biomass production of R. nasutus hydroponics when compared to the control (Table 2). In contrast, the elicitor-contact periods exhibited different eliciting powers on the rhinacanthin production of the hydroponics plant. An increase in the contact period for chitosan and T. harzianum extract elicitations to 72 h resulted in a decrease in rhinacanthin production (Table 2). However, the optimal contact periods that gave the highest content of rhinacanthins for chitosan and T. harzianum extract elicitations were 48 and 24 h, respectively. In contrast, a significant increase in rhinacanthin production was observed when the contact period of lawsone was increased to 72 h. The results indicated that each elicitor needed their own optimal elicitor contact periods to stimulate secondary metabolite production. Therefore, the suitable elicitation periods for chitosan, T. harzianum extract, and lawsone to increase rhinacanthin production in the roots were 48, 24, and 72 h, respectively, which maximized rhinacanthin production up to 6.1%, 6.1%, and 4.6% w/w, respectively.
Elicitors and Contact periods | Dried root biomass (mg/ plant) | Rhinacanthin content* (% w/w) | |||
---|---|---|---|---|---|
RC | RD | RN | Total | ||
Chitosan (0.15 mg/mL) | |||||
24 h | 125.88±3.01a | 3.95±0.03a | 0.41±0.01a | 0.52±0.01a | 4.89±0.03a |
48 h | 123.31±8.26a | 4.96±0.07b | 0.48±0.01b | 0.67±0.02b | 6.12±0.09b |
72 h | 116.64±7.43a | 4.58±0.29b | 0.43±0.02a | 0.59±0.04a | 5.61±0.35b |
T harzianum extract (1.0 mg/mL) | |||||
24 h | 121.70±4.99a | 5.63±0.08c | 0.22±0.02c | 0.22±0.01c | 6.07±0.08b |
48 h | 119.28±3.14a | 5.53±0.10c | 0.29±0.03c | 0.22±0.01c | 6.04±0.12b |
72 h | 117.03±6.46a | 3.92±0.08a | 0.17±0.01d | 0.16±0.01d | 4.25±0.08c |
Lawsone (3.0 μM) | |||||
24 h | 131.60±3.35a | 3.56±0.03d | 0.13±0.01d | 0.36±0.01e | 4.15±0.03c |
48 h | 121.01±14.35a | 3.59±0.05d | 0.22±0.01c | 0.41±0.01f | 4.22±0.05c |
72 h | 128.82±12.92a | 3.86±0.02a | 0.25±0.01c | 0.46±0.01f | 4.57±0.02d |
During the determination of elicitor contact periods, the leaves of R. nasutus hydroponics plants were also harvested and subjected to the determination of the dried leaf biomass and rhinacanthin content. The results are shown in Table 3. Treatment with chitosan for 24 h gave the highest content of rhinacanthin in the leaves, up to 5.4% w/w, which was significantly higher than the control leaves (4.6% w/w). Therefore, the leaves should be harvested after 24 h of chitosan elicitation, but the roots should be harvested after 48 h of treatment. In contrast, variation of the contact periods between 24 and 72 h did not significantly affect rhinacanthin production by T. harzianum extract elicitation. The highest content of rhinacanthins (6.7% w/w) was observed after 24 h of treatment. While a significant increase in rhinacanthin production (5.1% w/w) was also observed when the contact period of lawsone was increased to 72 h. Therefore, roots and leaves of the hydroponics plants could be harvested at the same time after the suitable contact periods of T. harzianum extract and lawsone.
Elicitors and Contact periods | Dried leaf biomass (mg/plant) | Rhinacanthin content* (% w/w) | |||
---|---|---|---|---|---|
RC | RD | RN | Total | ||
Control | 158.83±8.76a | 3.94±0.03a | 0.22±0.01a | 0.42±0.01a | 4.58±0.03a |
Chitosan (0.15 mg/mL) | |||||
24 h | 168.90±11.41a | 4.77±0.11b | 0.24±0.01a | 0.42±0.01a | 5.42±0.11b |
48 h | 165.00±13.04a | 4.15±0.03a | 0.22±0.03a | 0.47±0.01b | 4.84±0.03c |
72 h | 173.86±15.62a | 4.11±0.39a | 0.20±0.02a | 0.52±0.03c | 4.82±0.42ab |
T harzianum extract (1.0 mg/mL) | |||||
24 h | 168.90±2.47a | 5.72±0.19c | 0.30±0.02b | 0.64±0.01d | 6.66±0.22d |
48 h | 165.00±16.27a | 5.84±0.22c | 0.23±0.01a | 0.51±0.02c | 6.58±0.26d |
72 h | 173.86±13.88a | 4.96±0.02a | 0.48±0.01c | 0.62±0.03d | 6.10±0.04d |
Lawsone (3.0 μM) | |||||
24 h | 162.56±14.25a | 3.88±0.01a | 0.23±0.01a | 0.50±0.01c | 4.60±0.02a |
48 h | 168.99±13.47a | 3.96±0.11a | 0.25±0.01a | 0.45±0.01b | 4.66±0.11a |
72 h | 172.85±11.89a | 4.38±0.07d | 0.29±0.01b | 0.46±0.01b | 5.14±0.07bc |
DISCUSSION
The elicitation technique serves as a fundamental approach employed to augment or induce the production of secondary metabolites in plants. Distinct plant species, including R. nasutus, necessitate varying elicitation techniques tailored to elevate their secondary metabolite output.14 Agents or stimuli utilized in elicitation are termed elicitors, and they can be categorized based on their origins into abiotic and biotic elicitors.15 The underlying mechanisms of elicitation across diverse elicitors predominantly involve the elicitors, substances, or factors acting as stressors that instigate defensive mechanisms, often resulting in the synthesis of secondary metabolites through intracellular signaling pathways.16 The objective of employing elicitors in the hydroponic cultivation of R. nasutus is to facilitate their absorption through the roots, thereby instigating the synthesis of rhinacanthin via intracellular signaling pathways. The application of elicitation techniques to different plant species necessitates consideration of several key factors, including plant age, elicitor concentration, elicitation duration, and harvesting timing. It is discerned that both biotic and abiotic elicitors exhibit the potential to enhance secondary metabolite production in specific plants. Consequently, the implementation of elicitors, encompassing both biotic and abiotic types, in the root system of R. nasutus for the purpose of augmenting rhinacanthin production remains a formidable challenge. Notably, owing to their relatively low toxicity, elicitors such as chitosan, T. harzianum extract, lawsone, salicylic acid, sodium alginate, and methyl jasmonate emerge as promising candidates for effective employment in enhancing rhinacanthin production. Furthermore, the strategic manipulation of specific contact times may potentially contribute to further amplifying rhinacanthin production within the hydroponic cultivation of R. nasutus.
Chitosan is a nontoxic, natural, and biodegradable polymer that is well-known to stimulate plant growth as well as induce defensive mechanisms in plants, both of which necessitate changes in plant metabolite profiles.17 Chitosan has been reported to activate jasmonic acid, a signal molecule involved in defensive gene regulation and Phospholipase C/protein Kinase C (PKC) cascades.18 Furthermore, it has been reported to stimulate naphthoquinone productions in some plant tissue cultures, such as an increased plumbagin production in Plumbago indica root cultures19 and an enhanced methylene-3,3-bilawsone biosynthesis in Impatiens balsamina root cultures.20 The ability of chitosan to specifically increase naphthoquinone production might be due to mimicking a natural defense response or stimulating the enzymes involved in the biosynthesis of naphthoquinones. Although the mechanisms of chitosan for eliciting naphthoquinone biosynthesis have not yet been clarified, various mechanisms have been proposed, including stimulation of the antioxidant defense machinery, stimulation of nitrogen metabolism, increased uptake of water, and reduction of transpiration and essential nutrients through adjusting cell osmotic pressure.17
Trichoderma spp. are natural soil microorganisms that have been reported to boost the biosynthesis of various bioactive phytochemicals. Trichoderma spp. frequently leads to higher nutrient absorption, greater root growth and development, increased production, and improved tolerance to different pressures, including disease. The delivery of some effector chemicals from Trichoderma spp. during the interaction with plants may be involved in these effects. In addition, the biostimulant mechanisms of Trichoderma extracts may include the production of mitogen-activated proteins, auxin, phenylpropanoids, and phytoalexins, as well as the release of volatile and non-volatile substances that increase plant survival and nutrient uptake.21,22
Lawsone is a naturally occurring naphthoquinone, uniquely found in the leaves of Lawsonia inermis and Impatiens balsamina. The present study is the first to demonstrate that lawsone is capable of increasing secondary metabolite, especially naphthoquinone production in plants. Up to date, the precise elicitation mechanism of lawsone has not been identified yet. However, the eliciting property of lawsone in rhinacanthin production might be related to its oxidative stress induction by increasing both H2O2 production and antioxidative enzyme activities in plant cells.23 The redox homeostasis in plants is generally known as a key factor involved in auxin-mediated growth regulation. On the other hand, according to its 1,4-naphthoquinone core structure with an ortho-hydroxyl group substitution, which is a subunit of the rhinacanthin core structure, therefore, it might be an intermediate in the biosynthetic pathways of rhinacanthins. In addition, lawsone and rhinacanthins have been proposed to be biosynthesized via the shikimate pathways.24 In this regard, the mechanism by which lawsone increases rhinacanthin production might be through the precursor feeding.
CONCLUSION
The present study established the practical elicitation methods to increase the production of rhinacanthins in the hydroponics system of R. nasutus using chitosan, T. harzianum extract, and lawsone as the elicitors. The optimized elicitation conditions made the hydroponics plant an alternative source of the high-rhinacanthin-producing roots and leaves of R. nasutus. The roots and leaves obtained from these cultivation systems contained a higher content of rhinacanthins than those obtained from natural sources and could be more easily harvested. Moreover, the established hydroponics systems might be a valuable model for exploring and elucidating the genes involved in the biosynthesis of rhinacanthins, as well as underlying the elicitation mechanism for increasing rhinacanthin production.
Cite this article:
Suksawat T, Panichayupakaranant P. Enhanced Rhinacanthin Production in Rhinacanthus nasutus Roots Using a Hydroponics and Elicitation System. J Young Pharm. 2024;16(2):216-22.
ACKNOWLEDGEMENT
This work was supported by the PSU-PhD Scholarship (grant no. PSU_PHD2562-005) and an overseas thesis research study (grant no. OTR2566-001) funded by the Graduate School of Prince of Songkla University, Songkhla, Thailand. The authors wish to thank Miss Saffanah Mohd Ab Azid for her assistance with English editing.
ABBREVIATIONS
ANOVA | Analysis of variance |
---|---|
HPLC | High performance liquid chromatography |
LED | Light-emitting diode |
MS | Murashige and Skoog |
ODS | Octadecyl-silica |
PKC | Phospholipase C/ protein Kinase C |
RC | Rhinacanthin-C |
RD | Rhinacanthin-D |
RN | Rhinacanthin-N |
References
- Suksawat T, Panichayupakaranant P. Variation of rhinacanthin content in and its health products. J Pharm Biomed Anal. 2023;224:115177 [PubMed] | [CrossRef] | [Google Scholar]
- Panichayupakaranant P, Charoonratana T, Sirikatitham A. RP-HPLC analysis of rhinacanthins in : validation and application for the preparation of rhinacanthin high-yielding extract. J Chromatogr Sci. 2009;47(8):705-8. [PubMed] | [CrossRef] | [Google Scholar]
- Puttarak P, Charoonratana T, Panichayupakaranant P. Antimicrobial activity and stability of rhinacanthins-rich extract. Phytomedicine. 2010;17(5):323-7. [PubMed] | [CrossRef] | [Google Scholar]
- Tewtrakul S, Tansakul P, Panichayupakaranant P. Effects of rhinacanthins from on nitric oxide, prostaglandin E2 and tumor necrosis factor-alpha releases using RAW264.7 macrophage cells. Phytomedicine. 2009;16((6-7)):581-5. [PubMed] | [CrossRef] | [Google Scholar]
- Tewtrakul S, Tansakul P, Panichayupakaranant P. Anti-allergic principles of leaves. Phytomedicine. 2009;16(10):929-34. [PubMed] | [CrossRef] | [Google Scholar]
- Boueroy P, Saensa Ard S, Siripong P, Kanthawong S, Hahnvajanawong C. Rhinacanthin-C extracted from (L.) inhibits cholangiocarcinoma cell migration and invasion by decreasing MMP-2, uPA, FAK and MAPK pathways. Asian Pac J Cancer Prev. 2018;19(12):3605-13. [PubMed] | [CrossRef] | [Google Scholar]
- Chuang KA, Li MH, Lin NH, Chang CH, Lu IH, Pan IH, et al. Rhinacanthin C alleviates amyloid-β fibrils’ toxicity on neurons and attenuates neuroinflammation triggered by LPS, amyloid-β, and interferon-γ in glial cells. Oxid Med Cell Longev. 2017;2017 [PubMed] | [CrossRef] | [Google Scholar]
- Saleem U, Gull Z, Saleem A, Shah MA, Akhtar MF, Anwar F, et al. Appraisal of anti- Parkinson activity of rhinacanthin-C in haloperidol-induced parkinsonism in mice: A mechanistic approach. J Food Biochem. 2021;45(4):e13677 [PubMed] | [CrossRef] | [Google Scholar]
- Shah MA, Khalil R, Ul-Haq Z, Panichayupakaranant P. α-glucosidase inhibitory effect of rhinacanthins-rich extract from leaf and synergistic effect in combination with acarbose. J Funct Foods. 2017;36:325-31. [CrossRef] | [Google Scholar]
- Shah MA, Muhammad H, Mehmood Y, Khalil R, Ul-Haq Z, Panichayupakaranant P, et al. Superoxide scavenging and antiglycation activity of rhinacanthins-rich extract obtained from the leaves of
. Pharmacogn Mag. 2017;13(52):652-8. [PubMed] | [CrossRef] | [Google Scholar] - Rosdah AA, Lusiana E, Reagan M, Akib A, Khairunnisa F, Husna A, et al. A preliminary study: extract modulates acetylcholine in the heart. J Phys Conf Ser. 2019;1246:e012048 [PubMed] | [CrossRef] | [Google Scholar]
- Shah MA, Reanmongkol W, Radenahmad N, Khalil R, Ul-Haq Z, Panichayupakaranant P, et al. Anti-hyperglycemic and anti-hyperlipidemic effects of rhinacanthins-rich extract from leaves in nicotinamide-streptozotocin induced diabetic rats. Biomed Pharmacother. 2019;113:108702 [PubMed] | [CrossRef] | [Google Scholar]
- Naik PM, Al-Khayri JM. Abiotic and biotic elicitors-role in secondary metabolites production through culture of medicinal plants. abiotic and biotic Stress in plants—recent advances and future perspectives. 2016:247-77. [PubMed] | [CrossRef] | [Google Scholar]
- Narayani M, Srivastava S. Elicitation: a stimulation of stress in plant cell/tissue cultures for enhancement of secondary metabolite production. Phytochem Rev. 2017;16(6):1227-52. [CrossRef] | [Google Scholar]
- Eilert U. Elicitation: methodology and aspects of application. Array. 1987;4:153-96. [CrossRef] | [Google Scholar]
- Hussain MS, Fareed S, Ansari S, Rahman MA, Ahmad IZ, Saeed M, et al. Current approaches toward production of secondary plant metabolites. J Pharm Bioallied Sci. 2012;4(1):10-20. [PubMed] | [CrossRef] | [Google Scholar]
- Sathiyabama M, Bernstein N, Anusuya S. Chitosan elicitation for increased curcumin production and stimulation of defence response in turmeric ( L.). Ind Crops Prod. 2016;89:87-94. [CrossRef] | [Google Scholar]
- Rajestary R, Landi L, Romanazzi G. Chitosan and postharvest decay of fresh fruit: meta-analysis of disease control and antimicrobial and eliciting activities. Compr Rev Food Sci Food Saf. 2021;20(1):563-82. [PubMed] | [CrossRef] | [Google Scholar]
- Jaisi A, Panichayupakaranant P. Chitosan elicitation and sequential Diaion® HP-20 addition a powerful approach for enhanced plumbagin production in root cultures. Process Biochem. 2017;53:210-5. [CrossRef] | [Google Scholar]
- Sakunphueak A, Panichayupakaranant P. Increased production of naphthoquinones in root cultures by elicitation with methyl jasmonate. Bioresour Technol. 2010;101(22):8777-83. [PubMed] | [CrossRef] | [Google Scholar]
- Siddaiah CN, Satyanarayana NR, Mudili V, Kumar Gupta V, Gurunathan S, Rangappa S, et al. Elicitation of resistance and associated defense responses in
. [PubMed] | [CrossRef] | [Google Scholar]