ABSTRACT
Background: To evaluate biological activity of synthesised new chromane and its analogues. Materials and Methods: New chromane {3,5,7-trihydroxy 2-(4-hydroxy benzyl) chroman-4-one} isolated from dried leaves of Dillenia indica Linn., family Dilleniaceae is structurally relating with various reported chroman-4-one derivatives displaying remarkable in vivo antidiabetic activity. But the literature reveals that 0.8 – 1.0% yield of pure new chromane was obtained in isolation. Following reported literature data of synthesis and in silico study; Synthesized new chromane and its derivatives (S23-S32) were investigated for in vitro (α-amylase and α-glucosidase) as well as in vivo antidiabetic evaluation respectively. Result and Conclusion: In vitro hypoglycaemic study also displayed the significant antidiabetic potential of new chromane and its O-alkyl substituents (especially S23) while other synthesized compounds (S27-S32) reported for moderate to mild effects w.r.t. reference drug (acarbose). Moreover, synthetic new chromane and O-alkyl substituent (S23) exhibited maximum antidiabetic activity also in terms of lowering glucose concentration while others (S27-S32) showed mild anti-diabetic effect in comparison to reference drug (metformin).
INTRODUCTION
Diabetes Mellitus (DM), a chronic metabolic illness, defined by hyperglycaemia due to inadequacies of insulin biosynthesis with release, action or both is rising globally immensely and become a complex serious metabolic damage with the increased prevalence diabetic complications like diabetic neuropathy and nephropathy.1–2 To overcome diabetes and -associated diabetic complications, several phytocompounds like isolated flavonoids; hesperitin, naringenin, sakuranetin, dihydroquercetin, quercetin, kaempferol, aromadendrin, eriodictyol, butin, pinocembrin, sophorafavanone G, sterubin and nymphaeol A are reported to possess good antidiabetic activity3 through inhibition of dipeptidyl peptidase-IV (DPP4), glucagon-like peptide 1 (GLP-1), Alpha glucosidase (α-glucosidase), peroxisome proliferator receptor gamma (PPAR-γ), phosphatidylinositol 3-kinase (PI3K) respectively.3 Moreover, various isolated and synthetic chromanone analogues like (E)-8-(3,7-dimethylocta- 2,6-dienyl)-5,7-dihydroxy-2-(4-hydroxyphenyl) chroman-4-one, (2S)-7-methoxy-6-(2-hydroxy-3-methylbut-3-en-1-yl)-2- (4-hydroxyphenyl) chroman-4- one, Bavachinone B, 7-hydroxy-2-(4-hydroxy-3-methoxyphenyl)-chroman-4- one, 3-Benzylidene-4-chromanones, 5, 7-Dimethoxy-3-(2′- hydroxybenzyl)-4- chromanone, 6-methyl-4-chromanone, 2H- Chromenylphenylox- azolones derivatives have already been reported for antidiabetic potential.4–12 Therefore, present study focused on novel new chromane i.e., 3,5,7-trihydroxy-2- (4-hydroxybenzyl)-chroman-4-one which was firstly isolated from dried leaves of Dillenia indica (D. indica) Linn., Family Dilleniaceae.13 New chromane is an example of chromanones series and structurally related to kaempferol, quercetin, isomyrecetin, and myricetin which are already reported for antidiabetic activity14. But, the % yield of isolated new chromane from D. indica (0.8-1.0%) is very less for further experimental study.13 To explore the diversity of chromanone nuclei, new chromane and its O-substituted analogues were synthesised at laboratory scale with good yield and purity.15 Furthermore, using reported literature data; synthesized new chromane and its analogues (S23-S32) were investigated for in vitro and in vivo antidiabetic effect in terms of measuring hypoglycaemic level respectively.15
MATERIALS AND METHODS
In vitro screening of hypoglycaemic effect
α-amylase enzyme reaction
The test / standard (10-100 μg/ml) was placed in a test tube and 0.02M sodium phosphate buffer (pH 6.9) containing α-amylase solution was added. The solution was pre-incubated at 25°C for 10 min.; later 1% starch solution in 0.02M sodium phosphate buffer (pH 6.9) was added and then further incubated at 25°C for 10 min. The reaction was terminated by adding 2 ml, dinitro salicylic acid reagent (40 mM, sodium phosphate buffer 1 M, NaOH 0.4 M). The test tubes were then incubated in boiling water for 5 min and cooled to room temperature. The reaction mixture was diluted with distilled water and the absorbance was measured at 25°C at 540 nm using a spectrophotometer. All tests were performed in triplicate. Acarbose was used as standard drug. A control was prepared using the same procedure replacing the test sample with distilled water and percent inhibition was calculated as follows.16–17
Percent inhibition = [(As – Ac) / As] x 100 Where Ac is the absorbance of the control and As is the absorbance of the sample or standard.
α-glucosidase enzyme reaction
Reaction mixture containing 320 μl of 100 mM phosphate buffer (pH=6.8), 50 μl of 10mM PNPG in the buffer and 10 μl of test / standard drug (10-100 μg/ml) in dimethyl sulfoxide was incubated at 30°C for 5 min. Then 20 μl of buffer containing 0.01 mg/ml of α-glucosidase enzyme was added to mixture. After incubation at 30°C for 5 min, 50 mM sodium hydroxide (3 ml) was added in the mixture. Absorbance at 410 nm of the liberated p-nitrophenol was measured. Acarbose was used as standard drug and percent inhibition was calculated as follows.17,18
Percent inhibition = [(A – B)/A] x 100 Where A was the absorbance of the control (blank, without sample/standard), B was the absorbance in the presence of the sample /standard
Experimental animals
Experimental protocol (MMCP-IAEC-87) was permitted by IAEC Institutional Animals Ethics Committee (1355/PO/Re/S/10/ CPCSEA). Male Wistar adult rats having 180-190 g were used and kept in standard environmental conditions (temperature and standard day cycle of 23 ± 2°C and 12 h respectively) as per prescribed CPCSEA guidelines as stated by Ministry of Environment and Forest, Government of India. A standard rodent feed and water were given to animals. Experimental animals were arranged into various groups (Group 1-13) including six rats each (Table 2).
In vivo antidiabetic activity
A freshly prepared solution of streptozotocin (50 mg/kg i.p.) in 0.1 M citrate buffer, pH 4.5 was administered intraperitoneally. The nicotinamide (100 mg/kg i.p.) was given intraperitoneally 15 min before streptozotocin (STZ) administration. Diabetic rats having glycosuria and hyperglycaemia (>140 mg/dl) were taken for experimental studies. Fasting glucose level was determined in triplicate in each rat by using glucose oxidase peroxidase reactive strips and a glucometer. The day of administration of test and standard drugs is considered as 0 day in experimental protocols. The standard drug and test compounds (100 mg/kg) were administered orally to rats once daily at 9:00 AM for 15 days to diabetic rats. The blood glucose concentration was determined in groups of rats treated with test drugs on 0-day, 5th day, 10th day and 15th day.19–20 A diagnostic kit for Glucose estimation was purchased from Reckon Diagnostics Pvt. Ltd., India.
Statistical analysis
Observations formulated as mean SEM and results of every test sample in respective activity were corelated with respective standard drug and control by the statistic software named Sigma Stat following one-way analysis of variance (ANOVA).21
RESULTS
In vitro hypoglycaemic effect
α-amylase inhibitory activity
The test compounds (new chromane and S23 – S32) were investigated for in vitro antidiabetic profile with the help of α-amylase inhibitory assay.22 The results were equated with std. antidiabetic named acarbose. Table 3 and Figure 1 suggesting the action of various synthetic compounds on absorbance and percentage inhibition of enzyme at numerous concentrations (10-100 μg/ml or μM). The observations of test drugs were equated with std. drug acarbose by applying standard statistical analysis. The synthetic compound S23 exhibited maximum antidiabetic activity in term of percentage inhibition of α-amylase enzyme (IC50 = 72.74μg/ml) followed by S25 (IC50 = 78.04 μg/ml), new chromane (IC50 = 78.59μg/ml), S26 (IC50 = 77.59 μg/ml), S24 (IC50 = 83.02 μg/ml), S28 (IC50 = 90.12 μg/ml), S27 (IC50 = 92.98 μg/ml), S30 (IC50 = 99.73 μg/ml), S29 (IC50 = 102.77 μg/ml), S32 (IC50 = 103.95 μg/ml) and S31 (IC50 = 119.46 μg/ml). These results are statistically compared with acarbose; standard antidiabetic drug (IC50 = 74.75 μg/ml).
α-glucosidase activity
The test compounds (new chromane and S23 – S32) were investigated for in vitro antidiabetic profile23 with the help of glucosidase inhibitory assay. Protocol observations were equated with std. antidiabetic named acarbose. Table 3 and Figure 1 suggesting the action of various synthetic compounds on absorbance and percentage inhibition of enzyme at various concentrations (12.5-400 μg/ml). The results of test drugs were equated with std antidiabetic drug acarbose and parent new chromane by applying standard statistical analysis method such as one way ANOVA. The synthetic compound new chromane, S23, S24 exhibited maximum antidiabetic activity in term of percentage inhibition of glucosidase enzyme These results are statistically compared with acarbose standard antidiabetic drug (IC50 = 94.94 μg/ml).
Compounds | R1(C3) | R2(C5) | R3(C7) | R4(C4,) | Structure |
---|---|---|---|---|---|
New Chromane | H | H | H | H | |
S23 | CH3 | H | H | H | |
S24 | C2H5 | H | H | H | |
S25 | C3H7 | H | H | H | |
S26 | C4H9 | H | H | H | |
S27 | H | H | H | ||
S28 | H | H | H | ||
S29 | H | H | H | ||
S30 | H | H | H | ||
S31 | H | H | H | ||
S32 | H | H | H |
Antidiabetic effect
Test compounds were investigated for antidiabetic profile in experimental rats with the help of streptozotocin induced diabetic test.24 The observations compared against std. antidiabetic named metformin. Table 4 suggesting the effect of various synthetic compounds on concentration of glucose in blood of diabetic animals at 100 mg/kg, p.o., dose at different time intervals such as 0th, 5th, 10th and 15th day.
It is evident from Table 4 and Figures 2 and 3 new chromane synthetic parent compound and its various derivatives viz., S23 – S32 were exhibit significant antidiabetic action in comparison to control and standard drug but any of the synthetic compound exhibit activity equivalent to the standard drug. Amongst various synthetic compounds, only new chromane, S23 compound lowers blood sugar level significantly w.r.t. reference.
DISCUSSION
A novel compound of chromanone/chroman-4-one series, named new chromane (2-(4-hydroxybenzyl) 3,5,7-trihydroxy chroman-4-one) isolated from dried leaves of Dillenia indica Linn., family Dilleniaceae is reported for its antidiabetic activity.4 But the literature reveals that 0.8 – 1.0% yield of pure new chromane was obtained when isolated naturally and as the literature indicates chroman-4-one nucleus has excellent potency as anti-oxidant and anti-diabetic.13 Therefore, this novel compound was synthesized at laboratory in order to improve its % yield and further explore its biological activity.15
This study was performed for in vitro and in vivo antidiabetic investigation of synthesized new chromane and its analogues (S23-S32) by following α-amylase, α-glucosidase and streptozotocin induced method respectively. Streptozotocin induces hyperglycaemia by impairing the glucose oxidation via inhibiting synthesis of insulin as of the pancreatic β-cells damage through alkylation of DNA chain and unnecessary generation of free radicals. Nicotinamide (NAD) exerts protective shield against the cytotoxic result of STZ and diminish damage to β-cell causing type 2 DM. in vitro hypoglycaemic study revealed that new chromane and S23 showed significant hypoglycemic effect whereas S27-S32 showed mild effect in comparison to reference drug (acarbose).26
Amongst various synthetic compounds, new chromane and S23 exhibited maximum anti-diabetic activity in comparison to control as well as standard drug (metformin). Therefore, in vivo evaluation revealed, new chromane and O-alkyl substituents (specifically S23) were found more potent than others because O-CH3 substitution help in stabilisation of the radical cation intermediate results good radical scavenging activity as well as hypoglycaemic effect by the electron-donating effect of the methyl group as well as stabilise the planar skeleton of the molecule (chromane) moreover, improve physicochemical properties of chromane.27,28
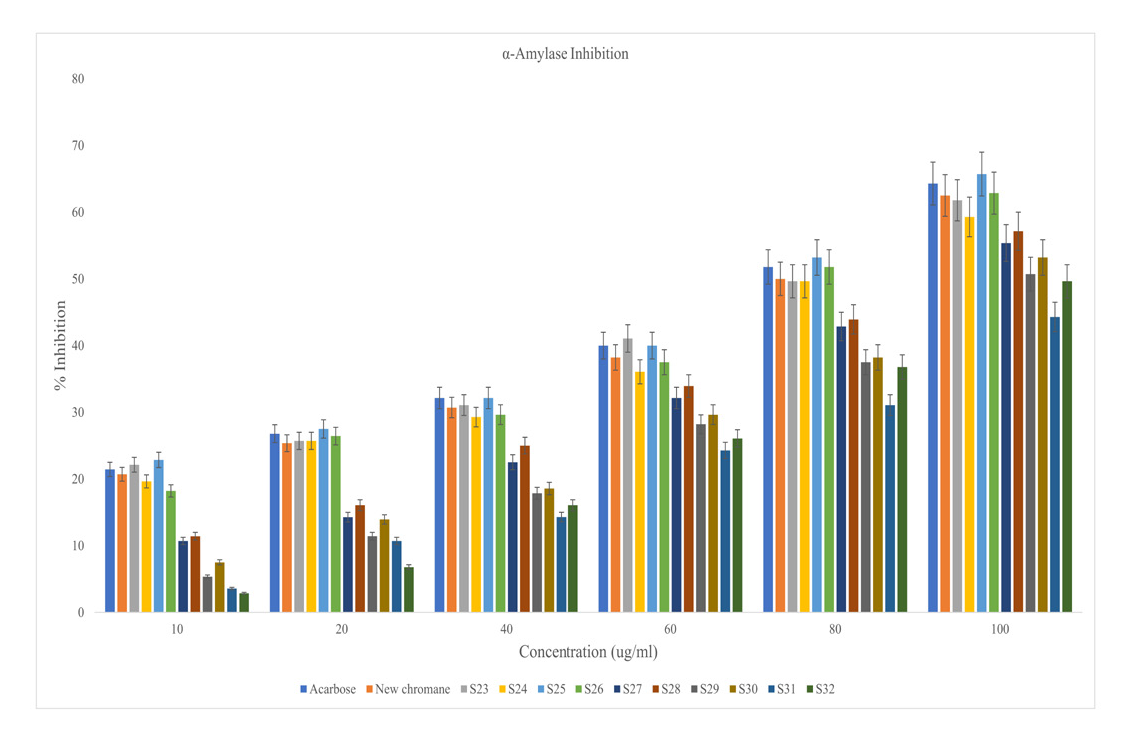
Figure 1.
α-amylase inhibition of new chromane, S23-S32 (test compounds) and acarbose (standard).
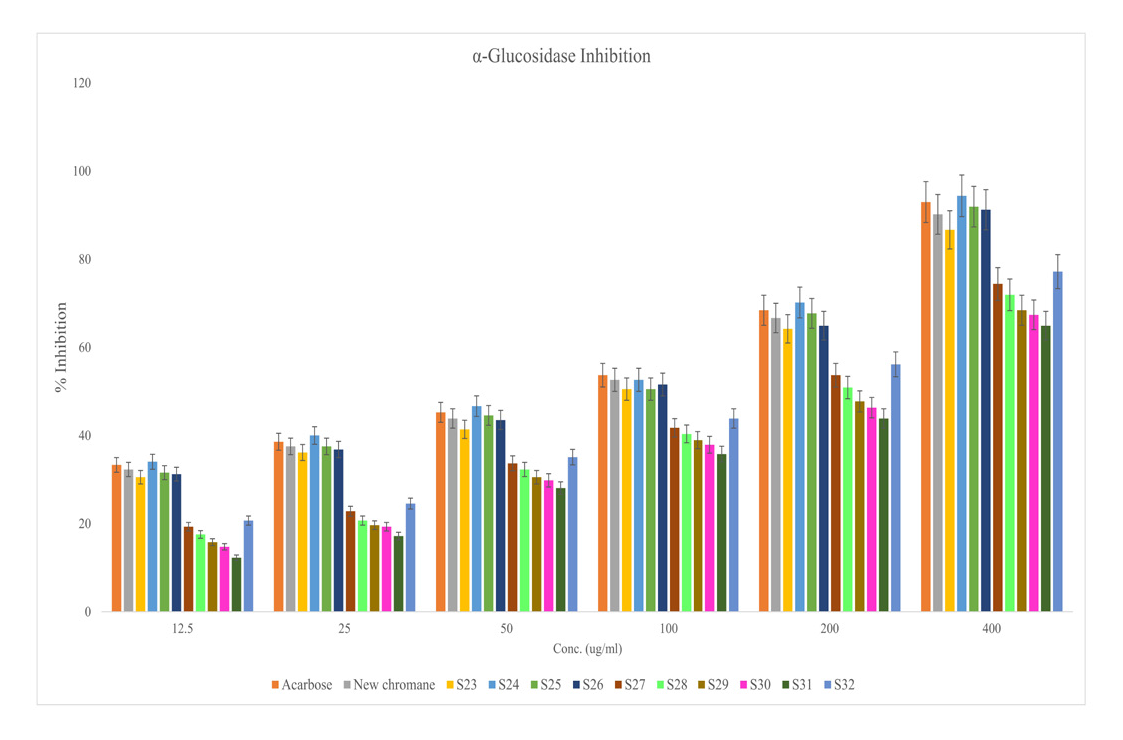
Figure 2.
Glucosidase inhibition of new chromane, S23-S32 (test compounds) and acarbose (standard).
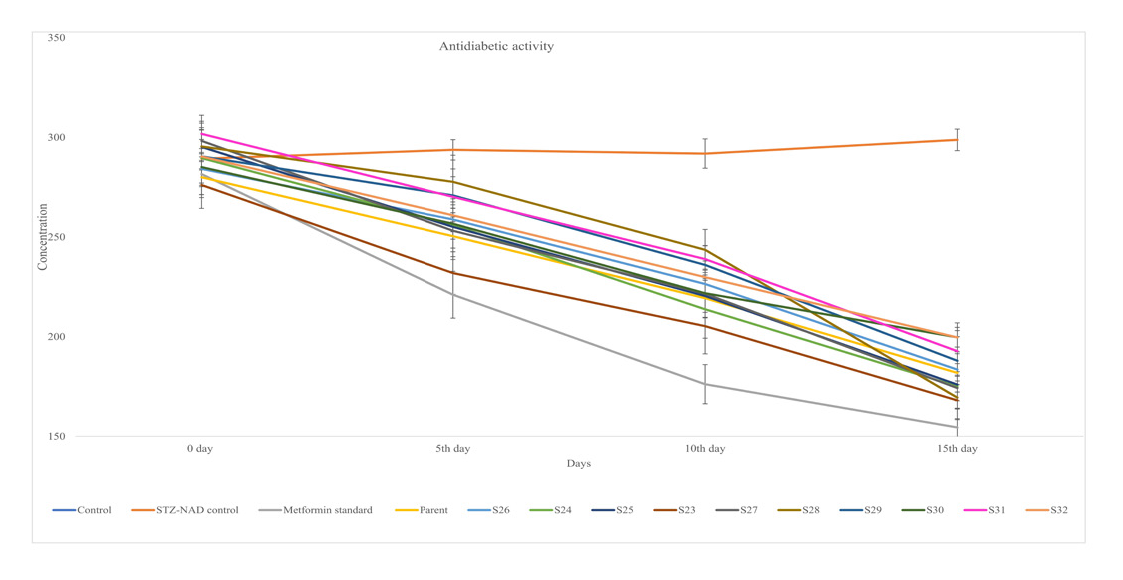
Figure 3.
Anti diabetic activity of test compounds (new chromane and S23-S32) vs metformin (standard) using STZ induced model.
Group 1: | Diabetes + Control |
---|---|
Group 2: | Diabetes + Standard (Metformin,150 mg/kg) |
Group 3: | Diabetes + New chromane (100 mg/kg) |
Group 4-13: | S23-S32 (100 mg/kg) analogues respectively |
Sl. No. | Compounds | α-amylase | α-glucosidase | ||
---|---|---|---|---|---|
% Inhibition | IC50(μg/ml) | % Inhibition | IC50(μg/ml) | ||
1 | Acarbose | 64.29±0.435 | 74.75 | 92.98±0.216 | 94.94 |
2 | New chromane | 62.50±1.149 | 78.59 | 90.18±0.351 | 104.42 |
3 | S23 | 65.71±0.546 | 72.74 | 86.67±0.385 | 101.88 |
4 | S24 | 59.29±0.413 | 83.02 | 94.39±0.359 | 99.05 |
5 | S25 | 61.78±1.444 | 78.04 | 91.93±0.536 | 104.65 |
6 | S26 | 62.86±0.899 | 77.59 | 91.23±0.352 | 109.44 |
7 | S27 | 55.36±0.358 | 92.98 | 74.39±1.233 | 197.99 |
8 | S28 | 57.14±0.891 | 90.12 | 71.93±0.357 | 214.11 |
9 | S29 | 50.71±0.619 | 102.77 | 68.42±1.128 | 234.56 |
10 | S30 | 53.21±0.351 | 99.73 | 67.37±0.054 | 242.83 |
11 | S31 | 44.29±0.413 | 119.46 | 64.91±0.234 | 261.52 |
12 | S32 | 49.64±0.715 | 103.95 | 77.19±0.206 | 182.09 |
Treatment | Dose (mg/kg) | Blood glucose concentration (mg/dl) | |||
---|---|---|---|---|---|
0 day | 5th day | 10th day | 15th day | ||
Control | Vehicle (1ml) | 89.47 ± 5.04*a | 86.98 ± 5.11*a | 86.54 ± 7.347*a | 86.57 ± 5.41* |
STZ-NA control | 50/100 | 289.34 ± 14.87 | 293.75 ± 11.98a | 291.88 ± 13.29a | 298.80 ± 9.44a |
Metformin standard | 150 | 281.58 ± 7.284 | 221.08 ± 8.39* | 176.19 ± 13.53* | 154.48 ± 9.27* |
New chromane | 100 | 279.02 ± 10.21 | 250.48 ± 11.72* | 219.32 ± 9.84* | 181.86 ± 9.61* |
S23 | 100 | 284.21 ± 15.67 | 258.85 ± 7.85* | 226.46 ± 12.59* | 183.54 ± 8.15* |
S24 | 100 | 289.61 ± 14.33 | 256.05 ± 11.58* | 213.79 ± 14.53* | 175.13 ± 11.40* |
S25 | 100 | 295.20 ± 12.43 | 255.16 ± 10.91* | 220.44 ± 9.89* | 176.00 ± 10.08* |
S26 | 100 | 298.28 ± 11.74 | 253.19 ± 10.68* | 221.59 ± 13.92* | 174.28 ± 9.71* |
S27 | 100 | 276.11 ± 9.86 | 231.94 ± 12.99* | 205.32 ± 9.40*a | 168.10 ± 6.45*a |
S28 | 100 | 295.43 ± 11.76 | 277.72 ± 13.32*ab | 243.62 ± 10.22*ab | 169.49 ± 10.62*ab |
S29 | 100 | 290.46 ± 14.46 | 270.93 ± 13.25*ab | 236.03 ± 9.73*ab | 187.99 ± 12.06*ab |
S30 | 100 | 285.11 ± 13.87 | 256.73 ± 7.77*ab | 221.82 ± 12.12*a | 199.73 ± 4.93*a |
S31 | 100 | 301.80 ± 9.37 | 270.16 ± 10.11*ab | 238.93 ± 6.70*ab | 192.80 ± 10.28*ab |
S32 | 100 | 290.42 ± 13.33 | 260.93 ± 8.24*ab | 229.90 ± 8.10*ab | 199.68 ± 7.26*ab |
CONCLUSION
From in vitro hypoglycemic evaluation, new chromane and O-alkyl substituents showed more reducing potential in relation to other compounds. The present report of in vivo antidiabetic activity, revealed that new chromane, S23 lowers blood sugar level significantly w.r.t reference.
In conclusion, new chromane scaffold is an interesting antidiabetic pharmacophore and considered as novel lead of chromane family for more imminent optimizations. Moreover, additional analogues may be proposed, synthesised and furthermore explore so as to establish more points in SAR based on rational.
Cite this article
Kamboj S, Chaudhary J, Jain A, Singh R. In vitro and in vivo Antidiabetic Evaluation of Synthesised Novel New Chromane and its Derivatives. J Young Pharm. 2023;15(2):292-7.
CONFLICT OF INTEREST
The authors declare no conflict of interest.
References
- Singh N, Kesherwani R, Tiwari AK, Patel DK. A review on diabetes mellitus. J Pharm Innov. 2016;5(7):36-40. [Google Scholar]
- Edwards JL, Vincent AM, Cheng HT, Feldman EL. Diabetic neuropathy: Mechanisms to management. Pharmacol Therap. 2008;120(1):1-34. [Google Scholar]
- Gupta R. Active phytoconstituents for diabetes management: A review. J Complement Integr Med. 2018;15(3):20170123 [Google Scholar]
- Talukdar A, Talukdar N, Deka S, Sahariah BJ. Dillenia indica (OUTENGA) as anti-diabetic herb found in Assam: a review. Int J Pharm Sci Res. 2012;3(8):2482 [Google Scholar]
- Srivastava BK, Pande CS. Chemical examination of bark of Dillenia indica. Acta cienc. Indica. 1981;7(1-4):170-4. [Google Scholar]
- Shome U, Khanna RK, Sharma HP. Pharmacognostic studies on Dillenia indica Linn. I. Leaf. Proc Indian Acad Sci. 1979;88:35-48. [Google Scholar]
- Yeshwante SB, Juvekar AR, Nagmoti DM, Wankhede SS, Shah AS, Pimprikar RB, et al. Anti-inflammatory activity of methanolic extracts of Dillenia indica L. leaves. J Young Pharm. 2009;1(1):63-66. [Google Scholar]
- Hasan MS, Ahmed MI, Mondal S, Masud MM, Sadhu SK, Ishibashi M, et al. Antioxidant, antinociceptive activity and general toxicity study of Dendrophthoe falcata and isolation of quercitrin as the major component. Adv trad med. 2006;6(4):355-60. [Google Scholar]
- Kumar S, Kumar V, Prakash O. Antidiabetic and antihyperlipidemic effects of Dillenia indica (L.) leaves extract. Braz J Pharm Sci. 2011;47(2):373-378. [Google Scholar]
- Kumar S, Kumar V, Prakash O. Antidiabetic and hypolipidemic activities of Dillenia indica extract in diabetic rats. Zhong xi yi jie he xue bao. Chin J Integr Med. 2011;9(5):570-4. [Google Scholar]
- Pavanasasivam G, Sultanbawa MUS. Chemical investigation of Ceylonese plants. Part 12. (+)-3,4’,5,7-Tetrahydroxy-3’-methoxyflavone [(+)-dihydroisorhamnetin] and 3,5,7-trihydroxy-3’,4’-dimethoxyflavone (dillenetin): Two new natural products from Dillenia indica L. J Chem Soc Perkin Trans.1. 1975;6:612-13. [Google Scholar]
- Kaur N, Kishore L, Singh R. Antidiabetic effect of new chromane isolated from Dillenia indica L. leaves in streptozotocin induced diabetic rats. J Funct Foods. 2016;22:547-55. [Google Scholar]
- Kamboj S, Singh R. Chromanone-A Prerogative Therapeutic Scaffold: An Overview. Arab J Sci Eng. 2022;47:75-111. [Google Scholar]
- Kamboj S, Sharma M, Singh R. Synthesis and molecular docking studies of new chromane (2-(4-hydroxybenzyl) 3,5,7-trihydroxychroma-4-one) and its O-substituted analogues. Int J Health Sci. 2022;6(S4):11899-11916. [Google Scholar]
- Minami Y, Kenjiro T, Takamatsu K, Matsouka T. Inhibition of α-amylase and α-glucosidase by flavonoids. J Nutr Sci Vitaminol. 2005;52:149-53. [Google Scholar]
- Chen W, Zhou C, Chen J, Wang M, Zhou F, Wang C, et al. Design and synthesis of epigallocatechin (EGC) analogs selective to inhibit α-amylase over α-glucosidases via the incorporation of caffeine acid and its derivatives. Bioorg Chem. 2022;119:105515 [Google Scholar]
- Zhen J, Dai Y, Villani T, Giurleo D, Simon JE, Wu Q, et al. Synthesis of novel flavonoid alkaloids as α-glucosidase inhibitors. Bioorg Medi Chem. 2017;25(20):5355-64. [Google Scholar]
- Dewanjee S, Das AK, Sahu R, Gangopadhyay M. Antidiabetic activity of Diospyros peregrina fruit: Effect on hyperglycemia, hyperlipidemia and augmented oxidative stress in experimental type 2 diabetes. Food Chem Toxicol. 2009;47(10):2679-2685. [Google Scholar]
- Deeds MC, Anderson JM, Armstrong AS. Single dose streptozotocin induced diabetes: Considerations for study design in islet transplantation models. Lab Anim. 2011;45(3):131-140. [Google Scholar]
- Boulfia M, Lamchouri F, Senhaji S, Lachkar N, Bouabid K, Toufik H, et al. Mineral content, chemical analysis, in vitro antidiabetic and antioxidant activities, and antibacterial power of aqueous and organic extracts of Moroccan Leopoldia comosa (L.) parl. Bulbs. Evidence-Based Complem Alt Medi. 2021;23:1-17. [Google Scholar]
- Prasad AR, Joseph A. Synthesis, characterization, in silico, in vitro biological screening of coordination compounds with 1, 2, 4-triazine based biocompatible ligands and selected 3d-metal ions. Heliyon. 2020;6(10):e05144 [Google Scholar]
- Rauter AP, Martins A, Borges C, Mota-Filipe H, Pinto R, Sepodes B, et al. Antihyperglycaemic and protective effects of flavonoids on streptozotocin-induced diabetic rats. Phyto. Res. 2010;24(S2):S133-8. [Google Scholar]
- Bohara M, Ghaju S, Sharma K, Kalauni SK, Khadayat K. In vitro, in silico analysis of Bergenia ciliataMimosa pudica for inhibition of α-amylase. J Chem. 2022;12:1-10. [Google Scholar]
- Kiruthiga N, Selvinthanuja C, Lalitha V, Sivakumar T, Abinaya N. Molecular modelling and evaluation on synthesised 2-phenyl-2, 3-dihydro-4 h-chromaffin-4-one core against oxidative stress. J med pharm allied sci. 2021;10(6):4060-4065. [Google Scholar]
- Imai K, Nakanishi I, Ohkubo K, Ohba Y, Arai T, Mizuno M, et al. Synthesis of methylated quercetin analogues for enhancement of radical-scavenging activity. RSC advances. 2017;7(29):17968-79. [Google Scholar]
- Murti Y, Semwal BC, Goyal A, Mishra P. Naringenin scaffold as a template for drug designing. Curr Tradit Med. 2021;7(1):28-44. [Google Scholar]