ABSTRACT
Psychological or behavioural tendencies that significantly impair one or more key areas of functioning or cause significant distress are considered psychological disorders. Psychological disorders exist in a wide variety, with their own set of signs and causes. Transport of drugs to brain takes place by one of the four methods namely, transport across blood brain barrier, nanoparticles, intracerebral implants and intranasal route. Using nanoparticles through intranasal route has shown great potential for targeted delivery to the brain. It is considered advantageous because of its as low dosing, biocompatibility and its versatility to encapsulate both hydrophobic and lipophilic drugs. Lipid based nanoparticles are prepared using two major components lipids and surfactants which aid in transport of drug to the brain. These particles can be categorised into liposomes and its modifications, solid-lipid nanoparticles and nanostructured lipid carriers. Each category has its own advantages which can be used for advancements in the drug delivery to the brain. This review focuses on the types of lipid-based nanoparticles and the recent research done on the same. More research and clinical trials are required to bring this novel method from laboratory to the market.
INTRODUCTION
Psychiatric disorders comprise a group of mental health conditions characterised by psychosis, in which reality is distorted. These disorders affect an individual’s thoughts, emotions and behaviour, leading to significant impairment in daily functioning. Genetic predisposition, abnormalities in brain structure or function, neurotransmitter imbalances (particularly dopamine), prenatal exposures and psychosocial stressors may contribute to the development of psychotic disorders. The symptoms of psychotic disorder such as schizophrenia, bipolar disorder etc., are managed using antipsychotic drugs. They work by modulating neurotransmitter activity in the brain, particularly dopamine and serotonin.1
Antipsychotics are categorized into typical (first-generation) and atypical (second-generation) classes. Selection of the specific medication depends on factors such as symptom severity, tolerability, side effect profile and patient preference. It’s important to monitor for efficacy, tolerability and side effects regularly and adjust medication as needed. The blood-brain barrier, however, presents a significant obstacle to the management of psychotic disorders.2
The blood-brain barrier controls the safe and targeted uptake, excretion, as well as metabolism of endogenous and exogenous substances inside and outside of the brain, protecting the central nervous system.3 Sadly, this means that many possible medications for the majority of brain disorders are likewise unable to pass through the blood-brain barrier. Different approaches to drug delivery and targeting are therefore being developed at this time. For drugs to achieve their intended sites in the Central Nervous System (CNS), the majority of implemented treatments seek to travel across the Blood-Brain Barrier (BBB).4
The strategies may include invasive techniques like direct injection or temporary disruption of the BBB, although the advantages are not always greater than the risks.5 Click or tap here to enter text. These days, nanotechnology-based methods are becoming more popular as a way to administer medications without being concerned about the molecules’ makeup because they often encapsulate any molecule, hiding any restricting Physical and chemical inadequacy.6
Anatomy of the Blood-Brain Barrier
The brain’s intracerebral milieu is preserved by the Blood-Brain Barrier (BBB), a highly selective anatomical barrier that protects the brain from harmful substances and environmental cues.7
The development of the BBB is essential to the successful evolution of the complex brain. It is mostly composed of cells that support immune function, such as microglia, neurons and basement membrane, in addition to astrocytes, pericytes and capillary endothelial cells. These parts, often called a Neurovascular Unit (NVU), maintain a healthy blood-brain barrier to ensure proper activation of the central nervous system.8
Tight junctions
TJs create a judicious diffusion blockade that blocks the majority of blood-borne materials from entering the brain by being enclosed between cerebral endothelial cells. The paracellular aqueous diffusional pathways connecting adjacent endothelial cells are blocked by TJs. Because of their adhesive properties, they block the passive diffusion of polar solutes and proteins within and outside of the Central Nervous System (CNS) and closed microvessels.9
Astrocyte end feet
Astrocytes end-feet encase blood vessels in the brain. It is believed that they preserve the fundamental framework of the cerebral vasculature. They are essential for the formation and upkeep of the TJ barrier. It is not thought that astrocyte end-feet serve as a barrier in the mammalian brain.10
Pericytes
Pericytes are multifunctional cells found in the lining of capillaries throughout the body and the brain. The Neurovascular Unit (NVU), a collection of cells that controls the interaction between brain cells and the cerebral vasculature to supply energy to the brain, includes pericytes.11 Pericytes are involved in the control of angiogenesis and vascular development, the restoration of the Blood-Brain Barrier (BBB) and the regulation of blood flow to the cerebrum. Pericytes can stimulate neuroinflammatory processes and exhibit properties similar to those of stem cells.12
Functions of Blood-Brain Barrier
The BBB is a part of the Neurovascular Unit (NVU), serving as the interface that mediates interactions between the central and peripheral nervous systems. Via a range of ion channels and transporters, the BBB provides a stable environment for brain activity while also preserving the optimal ionic makeup for synaptic signalling function.13,14
The BBB blocks the brain from receiving a lot of macromolecules. The BBB has low passive permeation concerning many of the essential water-soluble nutrients and metabolites which nervous tissue requires. As a result, the BBB specifies particular transportation networks to ensure an adequate supply of these drugs.15,16
It guards the CNS from chemicals that can cause neurotoxicity. These neurotoxins could be endogenous proteins or metabolites, or they could be xenobiotics that were obtained from the environment or consumed through food.17
TRANSPORT OF DRUGS TO BRAIN
Transport of drugs to the brain takes place by one of the four methods as mentioned in Figure 1.
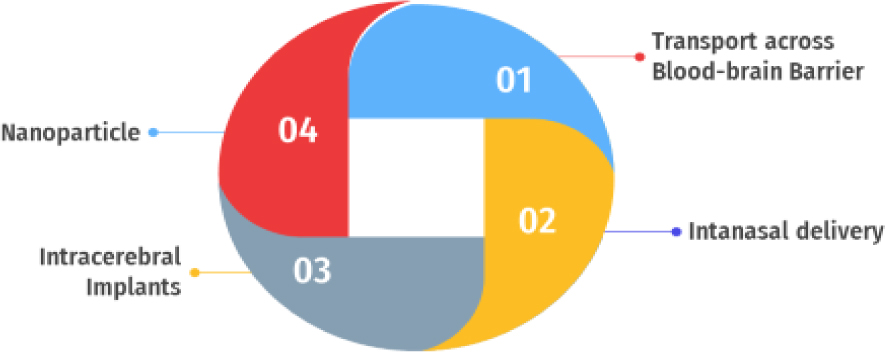
Figure 1.
Transport of drugs to brain.
Transport beyond the BBB
Transport across the Blood Brain Barrier is carried out by various pathways such as paracellular pathway, transport proteins, lipophilic diffusion, receptor mediated transcytosis and adsorptive transcytosis. The mechanism of transport is depicted in Figure 2.
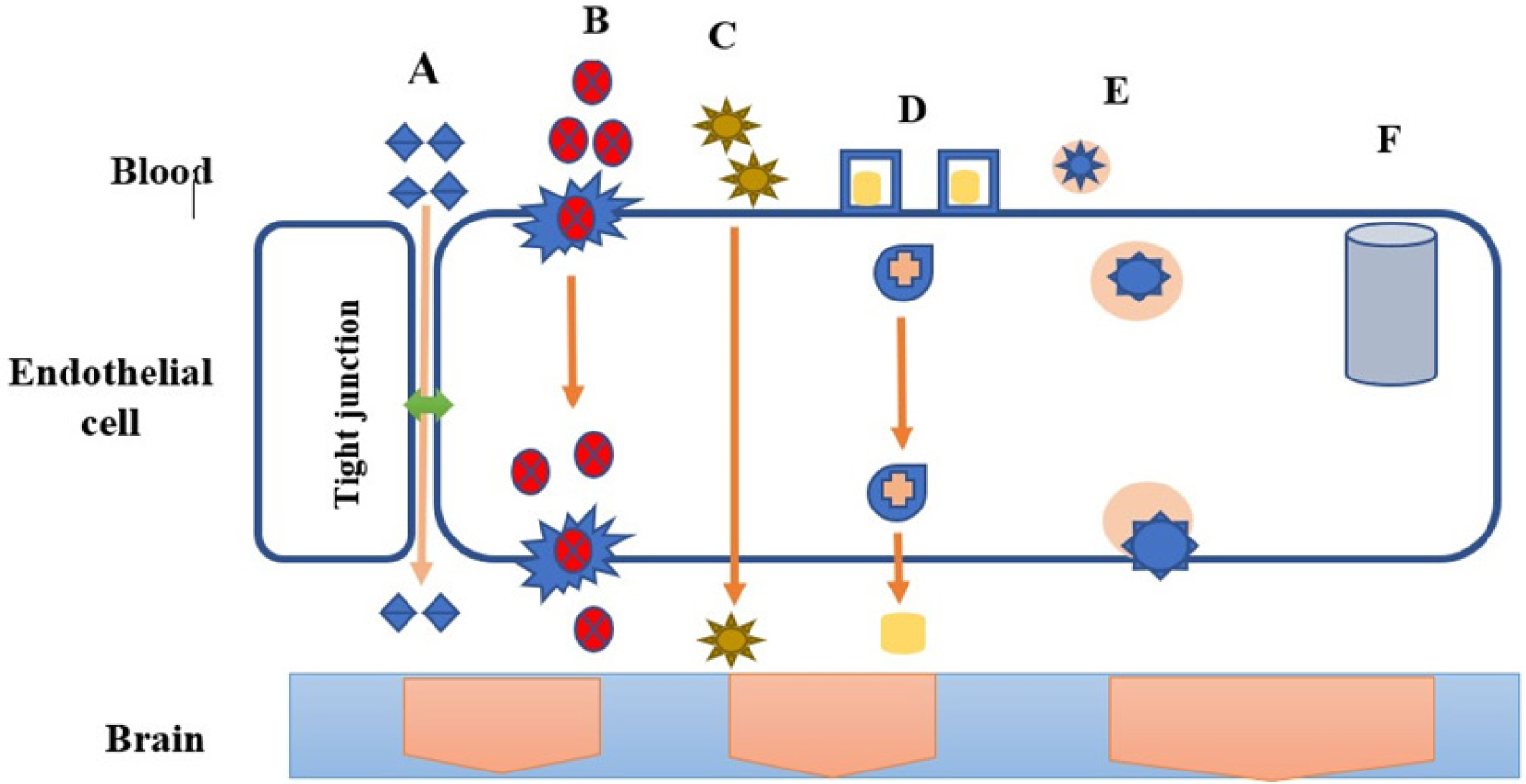
Figure 2.
Transport across blood brain barrier; (A) Paracellular Pathway (B) Protein mediated transport (C) Lipophilic diffusion (D) Receptor mediated Transcytosis (E) Adsorptive Transcytosis (F) Efflux pumps.
Paracellular pathway
In contrast to the peripheral microvessel wall, the Blood Brain Barrier’s tight junctions formed by endothelial structure limit the passage of hydrophilic molecules through the intercellular gaps.18
Transport proteins
Transport proteins play an evident contribution in mediating the route of specific molecules across the Blood-Brain Barrier (BBB). Proteins such as GLUT, amino acid, nucleosides and choline transporters aid in selective permeability of the BBB, ensuring that essential nutrients and molecules required for proper brain function are efficiently transported while limiting the passage of potentially harmful substances.19 To develop methods for delivering medications for the treatment of neurological diseases, it is imperative to comprehend the mechanisms underlying protein-mediated transport through the blood-brain barrier.20
Lipophilic diffusion pathway
The process of passive diffusion can occur spontaneously, based on the nature of the concentration gradient. Several molecules soluble in lipids have the ability to pass through cell membranes and passively diffuse into the brain through the endothelium.21
Receptor-mediated transcytosis
Depending on the composition of the concentration gradient, passive diffusion may happen on its own. Many molecules that are soluble in lipids can cross cell membranes and diffuse into the brain via the endothelium.22 Certain molecules that the receptors recognise can be transported via the controlled and selective process of receptor-mediated transcytosis. The BBB is crossed by drug delivery systems that take advantage of this mechanism, especially for substances that might not pass through the barrier through passive diffusion or different transport mechanisms.23
Adsorptive transcytosis
The BBB is well-suited to the AMT process because it offers the ability for cationic molecules to attach to the endothelial cells and then undergo exocytosis at the abluminal surface; additionally, the BBB’s transcytosis pathways, together with its morphological and enzymatic characteristics and the high mitochondrial content of cerebral Endothelial Cells, provide the means for the molecules to be transported through the cytoplasm of the endothelial cells.24,25
Intranasal drug delivery
A helpful method for treating neurologic disorders is the Intranasal (IN) administration of medications to the CNS. Subject to drug size or charge, the Blood-Brain Barrier (BBB) prevents many drugs from penetrating the Central Nervous System (CNS), which limits their ability to assist with memory loss and neurodegeneration.26
Transport of API from nasal cavity to brain
When API is introduced in the nasal cavity, it undergoes 3 pathways as mentioned in Figure 3. Since many potential medications cannot cross the blood-brain barrier, such as oxytocin, IGF-1, insulin, glutathione and many others, nose-to-brain transport is necessary.27
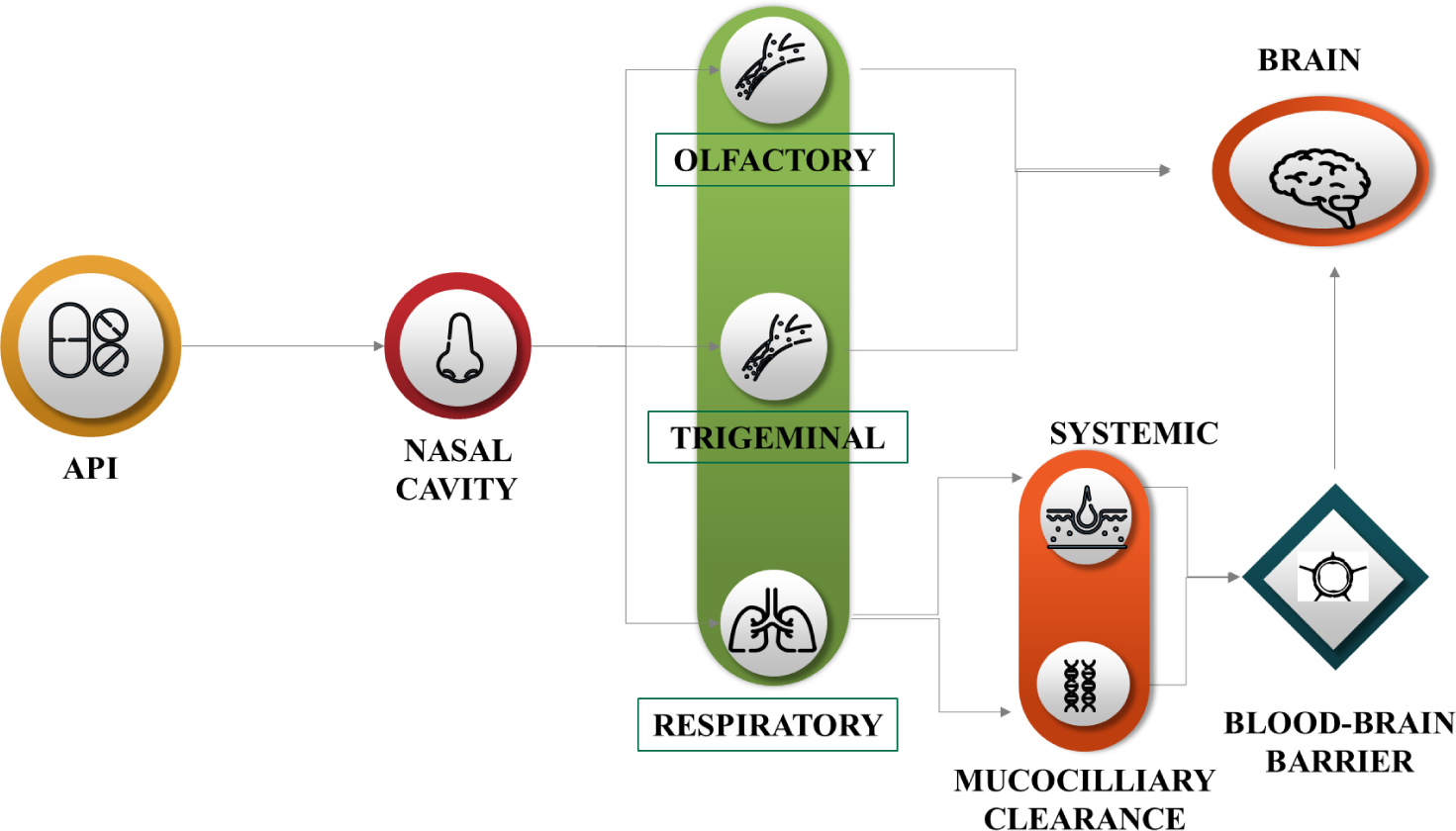
Figure 3.
Transport of API from nasal cavity to brain.
Compared to traditional delivery methods, intranasal administration will be advantageous for all of these compounds to reach the central nervous system. There will be an increasing need for novel therapeutic targets in the brain, necessitating the development of more specialised delivery systems, as our knowledge of the brain, its internal circuits and the pathophysiology of central nervous system disorders grows.28 Certain brain regions that would not normally be accessible may be directly accessed through the olfactory, trigeminal and vomeronasal routes. Optimising this route or routes, as well as fully comprehending dosing and safety after nasal drug administration are still necessary to target neurons for a direct CNS therapy.29
Intracerebral implants
Research on brain implantable technologies, like neurostimulators, is advancing quickly. They have been employed to manage and treat various illnesses, including epilepsy, Parkinson’s disease and depression that is resistant to treatment.30
Johannes Gurke et al. presented a simple method for the electrode array and microfluidic channel prototyping of intracranial implants. They demonstrated how the implant can concurrently record brain activity using its electrodes and modify hippocampal neuronal activity through localised drug delivery. Additionally, after a week of implantation, the implants showed little tissue reaction and good implant stability. This work opens the door for a new strategy in the creation of multifaceted implants and demonstrates the possibilities of hybrid fabrication, which combines multiple methods of production in neurotechnology.31
BRAIN-TARGETED DRUG DELIVERY USING NANOPARTICLES THROUGH INTRANASAL ROUTE
Drugs and biological products may cause issues because of their physicochemical characteristics after being administered intravenously. On the other hand, the nasal cavity’s typical anatomical features might cause the formulation to clear quickly.32 Combining excipients that prolong nasal residence time with micro- and nanotechnologies can be used in creation strategies.33 Nasal delivery necessitates a device that can sufficiently disperse the dosage form and guarantee its deposition in the necessary region of the nasal cavity, in addition to formulation characteristics. A detailed description of the formulation, its excipients and the dosing device is necessary to explain the selection of both the excipient and the device.34
Nanoparticles have shown promising potential in delivering drugs and therapeutic agents beyond BBB for various disorders.35 Various types of nanoparticles are shown in the Figure 4 This review focuses on brain-targeted drug delivery using nanoparticles through the intranasal route.
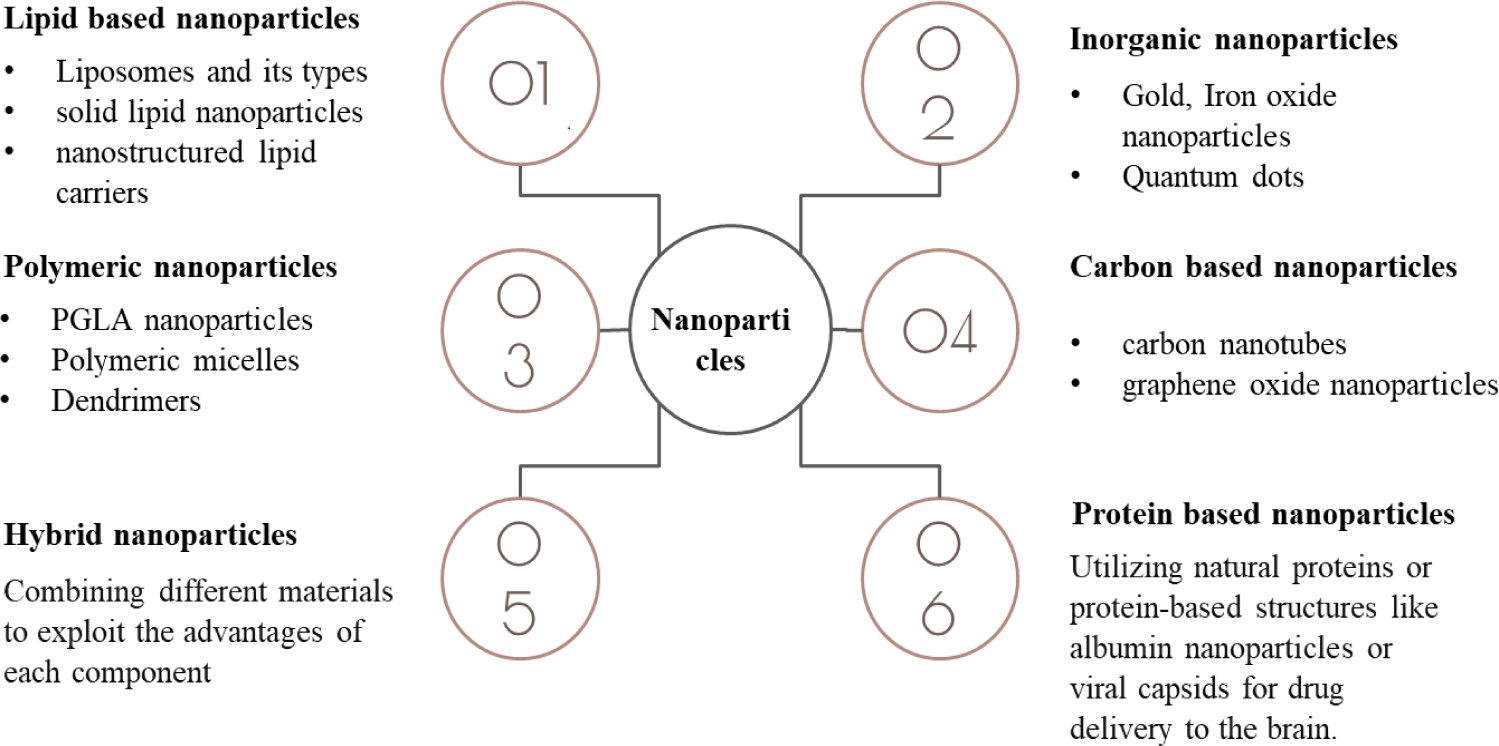
Figure 4.
Types of nanoparticles.
Lipid-based nanoparticles
A class of nanocarriers known as lipid-based nanoparticles uses lipids as their main structural element. Because of these nanoparticles’ biocompatibility, adaptability and capacity to incorporate both lipophilic and hydrophilic drugs, drug delivery with them has been the subject of extensive research36 These lipids-based vesicular structures can be classified into the following:
Liposomes
The most studied nanocarriers utilised in targeted drug delivery systems are liposomes. Liposomal vesicles, known as liposomes, are produced through the emulsification of either synthetic or natural lipids in an aqueous medium.37 They are composed of one or more lipid bilayers and generally exhibit a diameter of 50-500 nm.38
To cross the blood-brain barrier through the intranasal route, Tatiana N. Pashirova et al. created liposomes exhibiting cationic charge using L-α-phosphatidylcholine and Dihexadecylmethyl Hydroxyethyl Ammonium Bromide (DHDHAB). Low haemolytic action against human red blood cells, good solubility properties towards the hydrophobic dye Orange OT, low critical association concentration (0.01 mM) and microbial inhibition against gram-positive bacteria, such as Staphylococcus aureus and Bacillus cereus, were all attained. When cationic liposomes with rhodamine B are administered by intravenous route as opposed to intravenously, it has been demonstrated that the latter method increases the bioavailability to the brain. This positive finding suggests that commercially available positively charged oximes could be used as non-invasive medical countermeasures against organophosphorus poisoning to reactivate central acetylcholinesterase through the “nose-brain” pathway.39
Niosomes
Niosomes are a new class of Nanoparticles (NPs) that mainly consist of a self-assembled bilayer of non-ionic surfactant. They have shown great promise as a vehicle for efficient transport of drug molecules to a variety of locations, including the brain.40
To transfer the loaded materials to the brain, niosomes, which are nanoscale vesicular carriers, can ensnare, encapsulate, or dissolve the active molecules.41 Niosomal vesicles improve chemical stability, boost cellular uptake and lessen systemic adverse effects. They can also increase bioavailability, enhance drug passage via the olfactory region and enhance patient compliance.42
Rasha A. Khallaf et al. formulated niosomes through surface modification using olanzapine as the drug for brain targeting via nasal route. Different ratios of surfactants were mixed with cholesterol to prepare the niosomes which were further coated with chitosan. The developed vesicles were examined using Confocal Laser Scanning Microscopy (CLSM) to determine their physicochemical and stability parameters. Additionally, rats were used to measure the optimised formula’s brain-targeting properties. When compared to the drug’s intranasal solution, the brain’s Olanzapine concentration increased threefold in the optimised nasal Chitosan-coated niosomes. To sum up, the vesicles that were created were effective in delivering Olanzapine to the brain through the nose.43
Transferosomes
Transferosomes are vesicular carrier systems that are specifically engineered to include an edge activator and an inner aqueous compartment encompassed by a bilayer of lipids.44 This lipid bilayer encircling the aqueous core creates ultra-deformable vesicles with the ability to self-optimize and self-regulate.45
An ionic-responsive in situ gel comprising nanotransfersomes has been developed by Somayeh Taymouri et al. for the nasal delivery of aripiprazole. The film hydration method was used to prepare the Aripiprazole-loaded TFS and an irregular factorial design was used for optimisation. Particle size, zeta potential, Polydispersity Index (PdI), Entrapment Efficiency (EE) and Release Efficiency (RE) were among the variables that were taken into account when optimising the prepared formulations. The optimised Aripiprazole transferosomes were dispersed in an ion-activated deacetylated Gellan gum solution and then examined for pH, gelling time, viscosity features and in vitro release research. When transferosomes and gel were compared, the drug release from the former was lower. The capability of gel to alleviate psychotic symptoms in mice and enhance drug delivery to the brain was demonstrated by the results of pharmacodynamic studies.46
Ethosomes
Ethosomes are microscopic spheres used to deliver medication through the skin.
They are a type of phospholipid vesicle, similar to liposomes, but with a key difference: ethosomes contain a high concentration of ethanol. This ethanol helps the ethosomes to permeate the deep layers of the skin, delivering the medication more effectively.47,48
Mohammed Layth Hamzah et al. developed Frovatriptan-loaded binary ethosomes nasal in situ gel for treatment of migraines. Formulation of binary ethosomes helped in overcoming several problems associated with oral routes such as low permeability. Ethosomes were formed using the film hydration technique and characterised by various parameters including vesicle size, zeta potential, polydispersity index and encapsulation efficiency. It was concluded that binary ethosomes gel poses an excellent non-invasive drug delivery enhancing the bioavailability and sustained drug release.49
Comparison between Liposomes and their types
Syeda Shabana Sultana et al. carried out comparison studies between liposomes, ethosomes and transferosomes using naproxen sodium as the active material. Naproxen sodium is an anti-inflammatory drug widely used in the treatment of rheumatoid arthritis. However, it has systemic side effects such as arrhythmia, wheezing and coughing caused by contraction of the bronchi. Development of lipid-based vesicular systems may help in overcoming these side effects. In contrast, liposomes maintained the drug release for an extended amount of time. Transferosomes were discovered to be a superior vesicular system when the entrapment efficiencies of all of the vesicular systems were compared. Ethosomes are found to be superior for topical drug delivery due to their small mean particle diameter. When it comes to topical gel formulation, ethosomes and transferosomes are far more desirable than liposomes. While ethosomes and transferosomes had first-order kinetics with a non-Fickian diffusion mechanism, liposomes had zero-order kinetics. Because liposomes follow zero order kinetics, they are therefore the most suitable drug delivery system for oral use.50,51
Solid Lipid Nanoparticles (SLNs)
They are a category of nanoparticle systems used in drug delivery and cosmetic formulations. They are composed of lipids that are solid at room temperature and stabilized with surfactants.52 They are commonly spherical in morphology with a vesicle size of 50-1000 nm. Solid lipid nanoparticles, which combine the best features of polymeric nanoparticles, liposomes and microemulsion, have completely transformed the landscape of drug delivery.53,54
Hezhong Ouyang et al. formulated solid lipid nanoparticles to improve the biopharmaceutical performance of Olanzapine via the intraperitoneal route. Olanzapine has a low Blood-Brain Barrier (BBB) penetration rate and is poorly soluble in water. Here, Olanzapine-SLNs were created by the High-Pressure Homogenization (HPH) technique and their particle properties were then examined. Furthermore, Olanzapine-SLNs’in vivo pharmacokinetic profiles and in vitro release profiles were contrasted with those of pure drugs. The bioavailability of Olanzapine-SLNs was found to be significantly enhanced (ninefold) in comparison to Olanzapine suspension. Ultimately, it can be said that, as demonstrated for Olanzapine in this study, SLNs possess the ability to transport active pharmaceutical ingredients to the brain, primarily to increase their bioavailability and antipsychotic effect.55
Nanostructured Lipid Carriers (NLCs)
A Nanostructured lipid carrier is an innovative pharmaceutical formulation comprising surfactants and co-surfactants, as well as physiological and biocompatible lipids.56 A novel advancement of lipid nanoparticles, known as nanostructured lipid carriers, arose in reaction to the shortcomings of the first generation, or SLNs. Emulsifiers and compatible, biodegradable liquid and solid lipids are used in the creation of NLCs.57,58
Nano-lipid carriers have shown a promising future as a means of medicine delivery by various routes of administration such as oral, parenteral. Ocular, pulmonary, topical and transdermal route. Recent applications of NLCs include brain targeting, chemotherapy, biotechnology, the food sector and the delivery of cosmeceuticals and nutraceuticals.58,59
Sanjay Kumar Singh formulated Asenapine nanostructured lipid carriers for improved drug delivery to the brain via the intranasal route. Asenapine maleate is an antipsychotic drug used in the treatment of schizophrenia. The oral bioavailability of this drug is less than 2% since it undergoes extensive hepatic metabolism. This limitation was overcome using the intranasal route of administration. They used a quality by design approach in the development and optimization of the lipid carriers. The Korsmeyer-Peppas model best fits the anomalous release observed in the in vitro drug release study, which lasted up to 24 hr. The characterization of the Asenapine nanostructured lipid carriers’s surface and solid state revealed spherical-shaped particles with a smooth surface, where Asenapine was present in a molecularly dispersed state within a lipid matrix. Furthermore, Asenapine nanostructured lipid carriers have a higher brain bioavailability, a better therapeutic and safety profile and an improved intranasal route to administration of pure drug as demonstrated by studies on the brain and plasma pharmacokinetics and long-term animal behavioural assessment. Nonetheless, pre-clinical biochemical and toxicological investigations are required for additional assessment. These results show that a novel and promising drug delivery method for the intranasal administration of Asenapine in the management of schizophrenia may be nanostructured lipid carriers.60
CONCLUSION
Treatment of the psychotic disorder is hindered by various factors such as low therapeutic efficacy, enzymatic degradation, tolerance, blood-brain barrier etc. The BBB regulates the distribution of substances to the brain. As a result, most of the medications in the management of brain disorders are not able to cross the BBB. The intranasal route of drug delivery is progressively used to treat brain-related disorders. This route helps in circumventing the barrier. The limitations of intranasal delivery such as low nasal residence time can be overcome by using appropriate excipients. Nanoparticles are also a potential method for transport of therapeutic agents across the blood-brain barrier. Lipid-based nanoparticles comprising liposomes and their modifications, solid lipid nanoparticles and nanostructured lipid carriers consist of lipids and surfactants. These have various advantages such as biocompatibility and the ability to incorporate both hydrophilic and lipophilic drugs. Overall lipid-based nanoparticles pose a promising approach for drug delivery to the brain. This will take more investigation in this field to bring these intriguing nanocarriers from the lab to the market. It is necessary to assess the toxicity profile of these nanocarriers and find solutions to issues related to nanomedicines, such as formulation and characterization complexity. These nanocarriers are developing slowly because there aren’t enough clinical studies and data.
Cite this article:
Sidhaye I, Karadagi AV, Harish KH, Dasankoppa FS, Swamy AHMV. Recent Advances in Intranasal Delivery with Lipid Nanoparticles for Brain Targeting: A Review. J Young Pharm. 2025;17(1):54-61.
ACKNOWLEDGEMENT
I would like to thank our Professors at Department of Pharmaceutics, KLE College of Pharmacy. Hubballi -580031, a constituent unit of KAHER, Belagavi for providing us with valuable guidance at insights throughout the process of writing this review. I would also like to extend warm regards to the Principal, KLE College of Pharmacy, Hubballi-580031 for providing this opportunity and platform for doing more literature review.
ABBREVIATIONS
BBB | Blood brain barrier |
---|---|
CNS | Central Nervous System |
NVU | Neurovascular Unit |
TJ | Tight junctions |
IN | Intranasal |
SLN | Solid Lipid Nanoparticles |
NLC | Nanostructured Lipid Carriers |
References
- McCutcheon RA, Reis Marques T, Howes OD. Schizophrenia-an overview. JAMA Psychiatry. 2020;77(2):201-10. [PubMed] | [CrossRef] | [Google Scholar]
- Remington G, Hahn MK, Agarwal SM, Chintoh A, Agid O. Schizophrenia: antipsychotics and drug development. Behav Brain Res [Internet]. 2021;414:113507 [PubMed] | [CrossRef] | [Google Scholar]
- Achar A, Myers R, Ghosh C. Drug delivery challenges in brain disorders across the blood-brain barrier: novel methods and future considerations for improved therapy. Biomedicines. 2021;9(12):1834 [PubMed] | [CrossRef] | [Google Scholar]
- Galea I. The blood-brain barrier in systemic infection and inflammation. Cell Mol Immunol. 2021;18(11):2489-501. [PubMed] | [CrossRef] | [Google Scholar]
- Ronaldson PT, Davis TP. Blood-brain barrier transporters: a translational consideration for CNS delivery of neurotherapeutics. Expert Opin Drug Deliv. 2024;21(1):71-89. [PubMed] | [CrossRef] | [Google Scholar]
- Helms HC, Kristensen M, Saaby L, Fricker G, Brodin B. Drug delivery strategies to overcome the blood-brain barrier (BBB). 2020:151-83. [PubMed] | [CrossRef] | [Google Scholar]
- Alahmari A. Blood-brain barrier overview: structural and functional correlation. Neural Plast. 2021;2021:6564585 [PubMed] | [CrossRef] | [Google Scholar]
- Fernandez M, Nigro M, Travagli A, Pasquini S, Vincenzi F, Varani K, et al. Strategies for drug delivery into the brain: a review on adenosine receptors modulation for central nervous system diseases therapy. Pharmaceutics. 2023;15(10):2441 [PubMed] | [CrossRef] | [Google Scholar]
- Mondal S, Ghosh S. Liposome-mediated anti-viral drug delivery across blood-brain barrier: can lipid droplet target be game changers?. Cell Mol Neurobiol. 2023;44(1):9 [PubMed] | [CrossRef] | [Google Scholar]
- Wevers NR, Vries HE. Microfluidic models of the neurovascular unit: a translational view. Fluids Barriers CNS. 2023;20(1):86 [PubMed] | [CrossRef] | [Google Scholar]
- Brown LS, Foster CG, Courtney JM, King NE, Howells DW, Sutherland BA, et al. Pericytes and neurovascular function in the healthy and diseased brain. Front Cell Neurosci. 2019;13:282 [PubMed] | [CrossRef] | [Google Scholar]
- Brown LS, Foster CG, Courtney JM, King NE, Howells DW, Sutherland BA, et al. Pericytes and neurovascular function in the healthy and diseased brain. Front Cell Neurosci. 2019;13:282 [PubMed] | [CrossRef] | [Google Scholar]
- Kadry H, Noorani B, Cucullo L. A blood-brain barrier overview on structure, function, impairment and biomarkers of integrity. Fluids Barriers CNS. 2020;17(1):69 [PubMed] | [CrossRef] | [Google Scholar]
- Alahmari A. Blood-brain barrier overview: structural and functional correlation. Neural Plast. 2021;2021:6564585 [PubMed] | [CrossRef] | [Google Scholar]
- Wu D, Chen Q, Chen X, Han F, Chen Z, Wang Y, et al. The blood-brain barrier: structure, regulation and drug delivery. Signal Transduct Target Ther. 2023;8(1):217 [PubMed] | [CrossRef] | [Google Scholar]
- Kaya M, Ahishali B. Basic physiology of the blood-brain barrier in health and disease: a brief overview. Tissue Barriers. 2021;9(1):1840913 [PubMed] | [CrossRef] | [Google Scholar]
- Umoh IO, dos Reis HJ, de Oliveira AC. Molecular mechanisms linking osteoarthritis and Alzheimer’s disease: shared pathways, mechanisms and breakthrough prospects. Int J Mol Sci. 2024;25(5):3044 [PubMed] | [CrossRef] | [Google Scholar]
- Fu BM. Transport across the blood-brain barrier. Adv Exp Med Biol. 2018;1097:235-59. [PubMed] | [CrossRef] | [Google Scholar]
- Nilles KL, Williams EI, Betterton RD, Davis TP, Ronaldson PT. Blood-brain barrier transporters: opportunities for therapeutic development in ischemic stroke. Int J Mol Sci. 2022;23(3):1898 [PubMed] | [CrossRef] | [Google Scholar]
- Mehta P, Soliman A, Rodriguez-Vera L, Schmidt S, Muniz P, Rodriguez M, et al. Interspecies brain PBPK modeling platform to predict passive transport through the blood-brain barrier and assess target site disposition. Pharmaceutics. 2024;16(2):226 [PubMed] | [CrossRef] | [Google Scholar]
- Bellettato CM, Scarpa M. Possible strategies to cross the blood-brain barrier. Ital J Pediatr. 2018;44(Suppl 2):131 [PubMed] | [CrossRef] | [Google Scholar]
- Barar J, Rafi MA, Pourseif MM, Omidi Y. Blood-brain barrier transport machineries and targeted therapy of brain diseases. BioImpacts. 2016;6(4):225-48. [PubMed] | [CrossRef] | [Google Scholar]
- Pulgar VM. Transcytosis to cross the blood brain barrier, new advancements and challenges. Front Neurosci. 2019:13 [PubMed] | [CrossRef] | [Google Scholar]
- Mohapatra P, Gopikrishnan M, Doss CGP, Chandrasekaran NN. How Precise are nanomedicines in overcoming the blood-brain barrier? A comprehensive review of the literature. Int J Nanomedicine. 2024;19:2441-67. [PubMed] | [CrossRef] | [Google Scholar]
- Mohapatra P, Gopikrishnan M, Doss CGP, Chandrasekaran NN. How Precise are nanomedicines in overcoming the blood-brain barrier? A comprehensive review of the literature. Int J Nanomedicine. 2024;19:2441-67. [PubMed] | [CrossRef] | [Google Scholar]
- Fonseca LC, Lopes JA, Vieira J, Viegas C, Oliveira CS, Hartmann RP, et al. Intranasal drug delivery for treatment of Alzheimer’s disease. Drug Deliv Transl Res. 2021;11(2):411-25. [PubMed] | [CrossRef] | [Google Scholar]
- Veronesi MC, Alhamami M, Miedema SB, Yun Y, Ruiz-Cardozo M, Vannier MW, et al. Imaging of intranasal drug delivery to the brain. Am J Nucl Med Mol Imaging. 2020;10(1):1-31. [PubMed] | [Google Scholar]
- Lombardo R, Musumeci T, Carbone C, Pignatello R. Nanotechnologies for intranasal drug delivery: an update of literature. Pharm Dev Technol. 2021;26(8):824-45. [PubMed] | [CrossRef] | [Google Scholar]
- Erdő F, Bors LA, Farkas D, Bajza Á, Gizurarson S. Evaluation of intranasal delivery route of drug administration for brain targeting. Brain Res Bull. 2018;143:155-70. [PubMed] | [CrossRef] | [Google Scholar]
- Dabbour AH, Tan S, Kim SH, Guild SJ, Heppner P, McCormick D, et al. The safety of micro-implants for the brain. Front Neurosci. 2021;15:796203 [PubMed] | [CrossRef] | [Google Scholar]
- Gurke J, Naegele TE, Hilton S, Pezone R, Curto VF, Barone DG, et al. Hybrid fabrication of multimodal intracranial implants for electrophysiology and local drug delivery. Mater Horiz. 2022;9(6):1727-34. [PubMed] | [CrossRef] | [Google Scholar]
- Formica ML, Real DA, Picchio ML, Catlin E, Donnelly RF, Paredes AJ, et al. On a highway to the brain: a review on nose-to-brain drug delivery using nanoparticles. Appl Mater Today. 2022;29:101631 [CrossRef] | [Google Scholar]
- Moreira R, Nóbrega C, de Almeida LP, Mendonça L. Brain-targeted drug delivery – nanovesicles directed to specific brain cells by brain-targeting ligands. J Nanobiotechnology. 2024;22(1):260 [PubMed] | [CrossRef] | [Google Scholar]
- Boyuklieva R, Zagorchev P, Pilicheva B. Computational, and . Biomedicines. 2023;11(8):2198 [PubMed] | [CrossRef] | [Google Scholar]
- Huang Q, Chen X, Yu S, Gong G, Shu H. Research progress in brain-targeted nasal drug delivery. Front Aging Neurosci. 2023;15:1341295 [PubMed] | [CrossRef] | [Google Scholar]
- Yonezawa S, Koide H, Asai T. Recent advances in siRNA delivery mediated by lipid-based nanoparticles. Adv Drug Deliv Rev. 2020;154-155:64-78. [PubMed] | [CrossRef] | [Google Scholar]
- Shah S, Dhawan V, Holm R, Nagarsenker MS, Perrie Y. Liposomes: advancements and innovation in the manufacturing process. Adv Drug Deliv Rev. 2020;154-155:102-22. [PubMed] | [CrossRef] | [Google Scholar]
- Nsairat H, Khater D, Sayed U, Odeh F, Al Bawab A, Alshaer W, et al. Liposomes: structure, composition, types and clinical applications. Heliyon. 2022;8(5):e09394 [PubMed] | [CrossRef] | [Google Scholar]
- Pashirova TN, Zueva IV, Petrov KA, Lukashenko SS, Nizameev IR, Kulik NV, et al. Mixed cationic liposomes for brain delivery of drugs by the intranasal route: the acetylcholinesterase reactivator 2-PAM as encapsulated drug model. Colloids Surf B Biointerfaces. 2018;171:358-67. [PubMed] | [CrossRef] | [Google Scholar]
- Al Jayoush AR, Hassan HA, Asiri H, Jafar M, Saeed R, Harati R, et al. Niosomes for nose-to-brain delivery: A non-invasive versatile carrier system for drug delivery in neurodegenerative diseases. J Drug Deliv Sci Technol. 2023;89:105007 [CrossRef] | [Google Scholar]
- Kauslya A, Borawake PD, Shinde JV, Chavan RS. Niosomes: A novel carrier drug delivery system. J Drug Deliv Ther. 2021;11(1):162-70. [CrossRef] | [Google Scholar]
- Sas AZ, El-Sawy sH, El-Nabarawi ma, Teaima Mh. Focus on niosomal-based drug delivery systems for nasal route: applications and challenges. Int J Appl Pharm. 2023:36-43. [CrossRef] | [Google Scholar]
- Khallaf RA, Aboud HM, Sayed OM. Surface modified niosomes of olanzapine for brain targeting via nasal route; preparation, optimization and evaluation. J Liposome Res. 2020;30(2):163-73. [PubMed] | [CrossRef] | [Google Scholar]
- Gupta R, Kumar A. Transfersomes: the ultra-deformable carrier system for non-invasive delivery of drug. Curr Drug Deliv. 2021;18(4):408-20. [PubMed] | [CrossRef] | [Google Scholar]
- Opatha SA, Titapiwatanakun V, Chutoprapat R. Transfersomes: A promising nanoencapsulation technique for transdermal drug delivery. Pharmaceutics. 2020;12(9):855 [PubMed] | [CrossRef] | [Google Scholar]
- Taymouri S, Shahnamnia S, Mesripour A, Varshosaz J. Array. Pharm Dev Technol. 2021;26(8):867-79. [PubMed] | [CrossRef] | [Google Scholar]
- Shabreen R, Sangeetha S. Ethosomes: A novel drug delivery system and their therapeutic applications -A review. Res J Pharm Technol. 2020;13(4):1972 [CrossRef] | [Google Scholar]
- Lin HM, Lin LF, Sun MY, Liu J, Wu Q. Topical delivery of four neuroprotective ingredients by ethosome-gel: synergistic combination for treatment of oxaliplatin-induced peripheral neuropathy. Int J Nanomedicine. 2020;15:3251-66. [PubMed] | [CrossRef] | [Google Scholar]
- Hamzah ML, Kassab HJ. Formulation and characterization of intranasal drug delivery of frovatriptan-loaded binary ethosomes gel for brain targeting. Nanotechnol Sci Appl. 2024;17:1-19. [PubMed] | [CrossRef] | [Google Scholar]
- Sultana SS, Sailaja AK. Preparation and evaluation of naproxen sodium-loaded liposomes, ethosomes and transferosomes. J Bionanosci. 2017;11(4):284-91. [CrossRef] | [Google Scholar]
- Nayak D, Tippavajhala VK. A comprehensive review on preparation, evaluation and applications of deformable liposomes. Iran J Pharm Res. 2021;20(1):186-205. [PubMed] | [CrossRef] | [Google Scholar]
- Paliwal R, Paliwal SR, Kenwat R, Das Kurmi BD, Sahu MK. Solid lipid nanoparticles: a review on recent perspectives and patents. Expert Opin Ther Pat. 2020;30(3):179-94. [PubMed] | [CrossRef] | [Google Scholar]
- Duan Y, Dhar A, Patel C, Khimani M, Neogi S, Sharma P, et al. A brief review on solid lipid nanoparticles: part and parcel of contemporary drug delivery systems. RSC Adv. 2020;10(45):26777-91. [PubMed] | [CrossRef] | [Google Scholar]
- Agrawal M, Saraf S, Saraf S, Dubey SK, Puri A, Patel RJ, et al. Recent strategies and advances in the fabrication of nano lipid carriers and their application towards brain targeting. J Control Release. 2020;321:372-415. [PubMed] | [CrossRef] | [Google Scholar]
- Ouyang H, Hu J, Qiu X, Wu S, Guo F, Tan Y, et al. Improved biopharmaceutical performance of antipsychotic drug using lipid nanoparticles via intraperitoneal route. Pharm Dev Technol. 2022;27(7):853-63. [PubMed] | [CrossRef] | [Google Scholar]
- Amiri M, Jafari S, Kurd M, Mohamadpour H, Khayati M, Ghobadinezhad F, et al. Engineered solid lipid nanoparticles and nanostructured lipid carriers as new generations of blood-brain barrier transmitters. ACS Chem Neurosci. 2021;12(24):4475-90. [PubMed] | [CrossRef] | [Google Scholar]
- Elmowafy M, Al-Sanea MM. Nanostructured lipid carriers (NLCs) as drug delivery platform: advances in formulation and delivery strategies. Saudi Pharm J. 2021;29(9):999-1012. [PubMed] | [CrossRef] | [Google Scholar]
- Alam T, Pandit J, Vohora D, Aqil M, Ali A, Sultana Y, et al. Optimization of nanostructured lipid carriers of lamotrigine for brain delivery: characterization and efficacy in epilepsy. Expert Opin Drug Deliv. 2015;12(2):181-94. [PubMed] | [CrossRef] | [Google Scholar]
- Chauhan I, Yasir M, Verma M, Singh AP. Nanostructured lipid carriers: A groundbreaking approach for transdermal drug delivery. Adv Pharm Bull. 2020;10(2):150-65. [PubMed] | [CrossRef] | [Google Scholar]
- Singh SK, Dadhania P, Vuddanda PR, Jain A, Velaga S, Singh S, et al. Intranasal delivery of asenapine loaded nanostructured lipid carriers: formulation, characterization, pharmacokinetic and behavioural assessment. RSC Adv [Internet]. 2016;6(3):2032-45. [CrossRef] | [Google Scholar]