ABSTRACT
Background
Remdesivir is a antiviral drug, which has approved in COVID-19 pandemic as emergency use. In order to solubilize the remdesivir in injection form, Sulfobutylether-Beta-Cyclodextrin (SBECD) is used as solubility enhancer. Since SBECB is excreted by the kidney and it is contradicted for patients with severe renal impairment.
Objectives
This research aim to formulate and evaluate the dry powder inhaler formulation of remdesivir mucoadhesive nanoparticle for respiratory diseases.
Materials and Methods
The nanoparticle was prepared by nanoprecipitation method using PLGA and Chitosan, the prepared nanoparticle was subjeted to various studies include physiochemical characterization, mucoadhesive properties. The formulation has loaded in Dry Powder Inhaler (DPI) and evaluated its dispensing properties.
Results
The prepared nanoparticle has spherical shape with 104.6 nm in size and zeta potential of -12.7 mV. The nanoparticle has 72.06% of entrapment efficiency with significant in sustained drug release of 85.1% at 48 hr. The mucoadhesive nanoparticle showed negligible damage in epithelial integrity with DPI emitted dose of 87.02% and MMAD of 2.90 μm. The prepared nanoparticle showed significant mucoadhesive strength of 77.1% with lungs mucosa.
Conclusion
Based on the above studies, remdesivir mucoadhesive nanoparticle deliver through DPI, it could be the best alternative route of administration during emergency situation.
INTRODUCTION
Drug has delivered to systemic circulation via inhalation route is an best alternative for parenteral or oral drug delivery.1 Drug reaches to lungs through inhalation technique has amble of advantages such as rapid onset of action,2 high bioavailability,3 improved patient compliance,4 self-administration, non-invasive nature5 and less drug degradation due to bypassing the first pass metabolism.6 Pulmonary route has significantly used for local delivery of drugs like antibiotic, protein, peptide, chemotherapeutics, enzymes, deoxyribonucleases, vaccines and much more.7 This route has many challenges that poor deposition in respiratory airway, fast clearance, incompetent penetration via epithelial and mucosal barriers, this could be the reason for frequent adminstration of drug. These limitations has been overcome by inhalation technique using nanocarriers, which has greater empowerment to encapsulate the molecules.8 It helps in penetration into the epithelial and mucosal barriers and it enhance the residence of drug within the pulmonary tract.
There are many nanocarriers for delivery of drugs to lung, among that the nanocarriers prepared by using chitosan, PLGA, poly caprolactone and polylactic acid are most preferable polymers used for encapsulation and enhance the sustained release of drugs.9
Remdesivir, is a broad-spectrum antiviral prodrug, which is actively metabolized as nucleoside triphosphate by the host. Its a Adenosine Nucleosides Analog (ANA) and it could cease the viral RNA polymerase, avoid proof reading by viral exonucleases and subsequently inhibit the viral RNA production.10 Numerous route to deliver remdesivir into human body such as Intravenous (IV), Intramuscular (IM) and oral administration has been well studied.11 It was found that do not suitable for oral delivery. Besides that, it has poor hepatic stability and bioavailability due to first-pass effect. IM route also facing many challenges including variable release from muscle and slow acting in peripheral blood mononuclear cells.12 Moreover, it is insoluble in water, so SBECD is used as solubility enhancer in IV injection. The complexed molecule is excreted by the kidneys and it is contradicted patients with severe renal impairment.13
In COVID-19 pandemic suitation, the covid virus has predomentaly affected the respiratory tract, especially in the deep lungs.14 Thus, the inhalation technique might be the promising route to maximize the direct delivery of drug into deep lungs by-passing the first-pass metabolism and it could boost the local antiviral activity. Moreover, many patients could be treat by low dose with less cost as compared with IV injection. Mucoadhesive nanoparticles are administered through nasal mucosa and it could protect the drug from enzymatic degradation, increases the uptake by the epithelium, enhance the drug dissolution rate, intensify the contact of the formulation within the mucosa and act as a controlled release system.15 Therefore, this work aimed to develop remdesivir mucoadhesive nanoparticles and to deliver through DPI and it could be the alternative route of administration of remdesivir.
MATERIALS AND METHODS
Materials
Remdesivir (Pure substance) was obtained as gift sample from Hetero Healthcare Ltd., India. PLGA (lactide:glycolide (50:50), mol wt 30,000-60,000) and Chitosan (Mol wt:160 kDa) was purchased from Labkart Scientific Solutions, India. Dimethyl Sulfoxide (DMSO), Dichloro Methane (DCM), Tween 60 were purchased from Finar chemicals, India. All the reagents and solvent were used in this research was analaytical grade.
Methods
Formulation of Remdesivir mucoadhesive nanoparticle
Remdesivir loaded mucoadhesive nanoparticle was formulated by the nanoprecipitation method.16 Chitosan was dissolved (1:1 ratio) separately with 0.25% v/v of acetic acid in bath sonicator for 5 min. About 100 mg of PLGA and 10 mg of remdesivir was dissolved separately in DMSO and the homogeneous mixture were added drop-wise into double the volume of aqueous solution containing 1% v/v of Tween 80. The mixture was subjected under Ultra-probe Q700 sonicator (Qsonica, Cole-Parmer India Pvt. Ltd., India) at 60 kHz frequency for 2 min. The nanoparticle was precipitated in the non-solvent system (water) and the resulted precipitated suspension was stirred in uncovered condition for 8 hr at room temperature. The formed nanoparticle was washed by centrifuge (Remi, India) at 1000 rpm for 30 min to detach the un-entrapped drugs. Finally, the nanoparticle was freeze-dried at -20°C using laboratory Lyophilizer (Borg Scientific, India) to obtain dry powder form.
X-ray Diffraction (XRD), and Differential Scanning Calorimetry (DSC) studies
X-ray diffractometer have been utilized to identify the crystalline changes of remdesivir in the nanoparticle and the physical status was tested by DSC analysis (Innovatech Labs, USA).
Estimation of encapsulation efficiency
The percentage of remdesivir entrapped within the nanoparticles was indirectly determined by centrifugation technique, the supernatant solution containig free drug has been estimated by RP-HPLC (PerkinElmer, USA) method.17
Determination of size, polydispersity index, zeta potential and surface morphology of nanoparticle
Particle size and Polydispersity Index (PDI) of the nanoparticle was evaluated by dynamic light scattering technique.18 The zeta potential was examined on the basis of electrophoretic mobility under a electric field by using Zetasizer (Malvern Panalytical Ltd., UK).
Scanning Electron Microscope (SEM) was used to study the morphology of nanoparticles. A little quantity of nanoparticles was spread on a metal stub and it was coated with conductive gold by Hitachi 1010 ion sputter. The prepared specimen could be observed under Hitachi 3000 N SEM (JSM 5610 LV SEM, JEOL, Japan) chamber. The image was clicked at an acceleration voltage of 20 kV with a chamber pressure of 0.6 mmHg.
In vitro drug release studies
In vitro drug release of nanoparticle was determined by using cellulose Dialysis tube19 (Himedia laboratory, India). Nanoparticle (drug equivalent of 2 mg) was dispersed in 5 mL of water and it loaded into Dialysis tube composed of cellulose (Molecular weight cut-off 35 kDa). Then the Dialysis tube was immersed in 300 mL dissolution medium at 37°C under a magnetic stirrering of 75 rpm. An adequate volume of the samples was eluted at different time interval and replaced with equal volume of the fresh receptor fluid. The eluted sample was centrifuged and the drug content of supernatant was estimated by using HPLC technique.
Lungs epithelial integrity test
Pulmonary epithelial cells integrity was calculated in Calu-3 type cell line.20 In description, 1×105 Calu-3 cells were placed in polycarbonate made insert of 24 transwell plates. The nutrients were supplied to cells with composition of Eagle’s essential medium with 10% Fetal bovine serum. Transition of epithelial electrical resistance was monitored every day with changing medium using an EVOM2 Epithelial Voltohmmeter (Labindia instruments, India) and the device value was maintained 900-1000 ohm-cm2, which indicate the monolayer formation. About 1 mg/mL of remdesivir loaded mucoadhesive nanoparticle were dispersed in growth medium and the electrical resistance was monitored at different time interval (0, 0.5, 1, 2, 4, 6,12 and 24 hr) and the plain medium as negative control.
Dispersion performance test
The US Pharmacopeia provides aerosol performance criteria as previously reported, the aerosol dispersion performance was assessed using Next Generation Impactor (TSI, USA).21 The NGI with a stainless steel induction port and the gravimetric insert cups were used to determine the aerosol dispersion parameters of the DPI. Prior to each experiment, the airflow rate (Q) was measured and adjusted in Flow-meter. The NGI was linked to a critical flow controller through an vacuum pump and set to a constant flow rate of Q=60 L/min. The effective cutoff diameter for each impactor stage for the NGI Q=60 L/min were calibrated by the manufacturer and are as follows: stage one (8.06 m); stage two (4.46 m); stage three (2.82 m); stage four (1.66 m); stage five (0.94 m); stage six (0.55 m); and stage seven (0.34 m). To reduce re-entrapment of particle, the glass fibre filter was kept in the stainless steel and the gravimetric insert cups for stages one through seven. Hard gelatin was loaded with 10 mg of nanoparticle and it is firmly placed into the induction port of high resistance DPI device. The mass of particles deposited into each stage was calculated gravimetrically for each run measuring the difference in mass of the glass filter after particle deposition. A mathematical programme was used to calculate the Mass Mean Aerodynamic Diameter (MMAD) and Geometric Standard Deviation (GSD), Fine Particle Fraction (FPF), Respirable Fraction (RF) and Emission Dose (ED). All experiments were performed in triplicate manner (n=3).
Determination of mucoadhesive binding efficiency of nanoparticle
The nanoparticle-mucin binding efficiency was calculated by using UV Spectrophotometic method.22 Briefly 0.5% v/v mucin was prepared by dissolving mucin in phosphate buffer (pH 6.5). From this, 1 mL of mucin solution was mixed with 2 mL of nanoparticle suspension (10 mg nanoparticles were suspended in 2 mL of distilled water). The mixture was incubated in an incubator at 37°C for 2 hr and then centrifuged for 2 hr at 35,000 rpm. The supernatant was extracted and the free mucin was calculated and the spectra were recorded at 255 nm using a UV-visible spectrophotometer (Jasco Spectrophotometer,Japan). The remdesivir nanoparticle was compared with blanks (Chtiosan nanoparticle and PLGA nanoparticle respectively). The mucin binding efficiency was determined using following formula.

RESULTS
The X-ray diffraction patterns of remdesivir nanoparticle has shown in Figure 1. The XRD spectra of remdesivir showed 6 sharp peaks at (2 theta) 5.37°, 8.45°, 12.61°, 14.45°, 16.05° and 22.20° and these sharp peaks were confirming that remdesivir is absolutly crystalline. Similarly, chitosan showed two distinguished peaks at 9.63 and 20.53 (2 theta), that would be the proof of semi-crystalline nature.
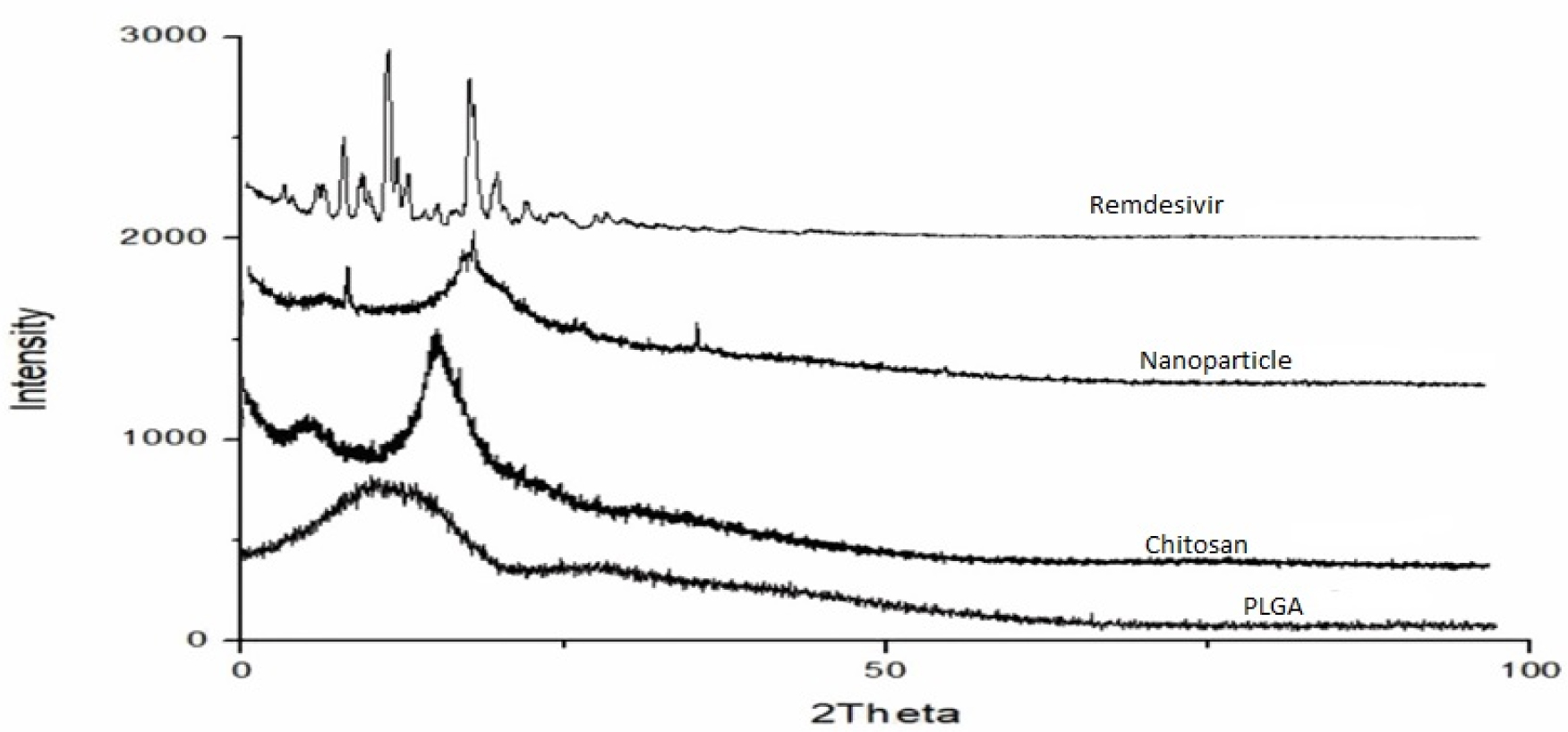
Figure 1:
XRD spectra of Remdesivir mucoadhesive nanoparticle.
The DSC spectra was used to estimate the glass transition Temperature (Tg) of remdesivir in the formulation. The thermograms of remdesivir and nanoparticle were shown in Figure 2. Remdesivir showed two exothermic peaks at 135.7 and 173.5°C and three endothermic peaks at 31.3, 121.7 and 132.6°C. These sharp peak confirmed that the remdesivir is crystalline nature. In other hand nanoparticle showed a broaden exothermic peak at 138.1°C and no other sharp peaks were observed, which concludes that remdesivir was matrix in polymeric network structure as amorphous form. Remdesivir nanoparticle provide better dissolution property in lung fluid that provide enhancement in the drug bioavailability and efficacy, when its administered through pulmonary route.23
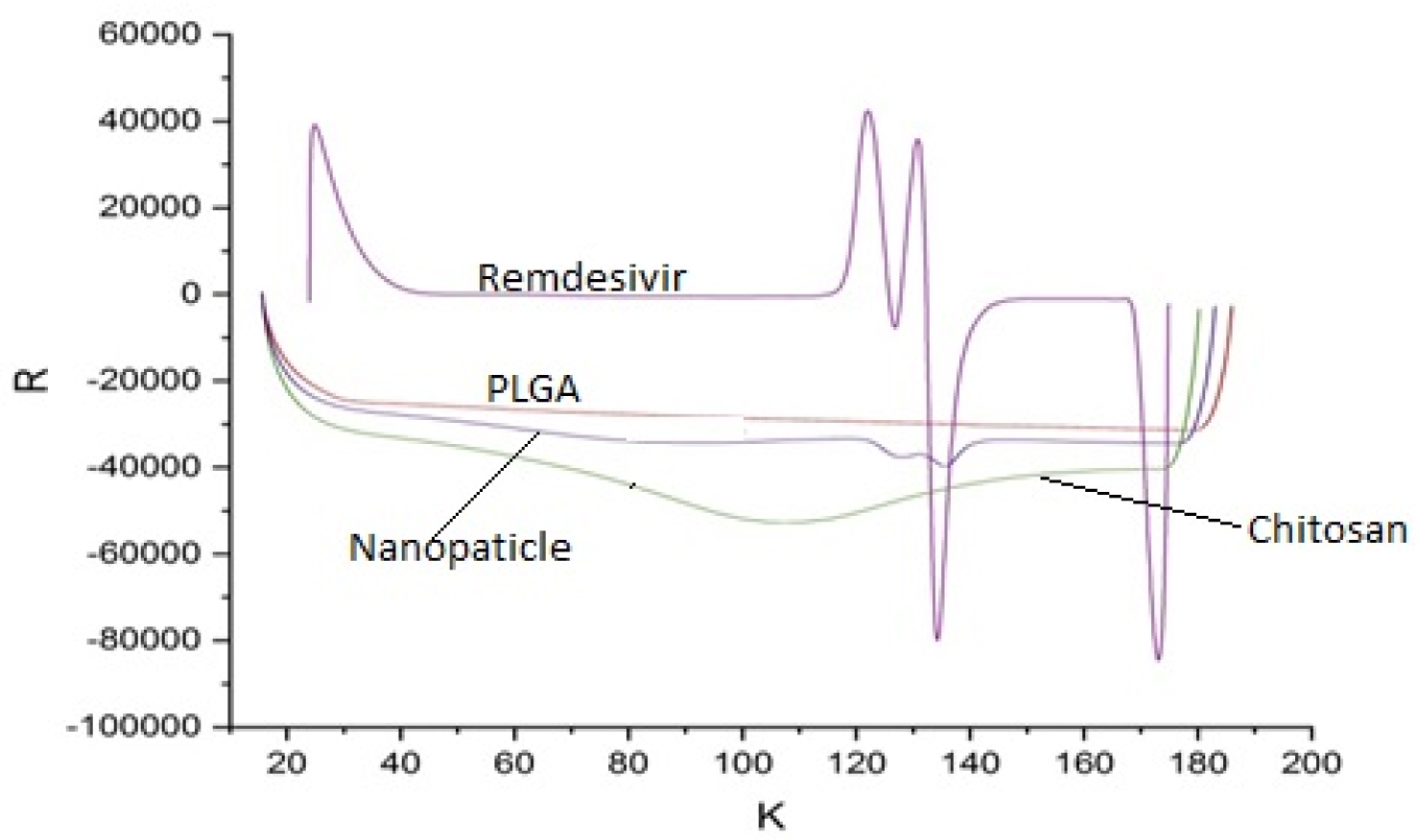
Figure 2:
DSC spectra of Remdesivir mucoadhesive nanoparticle.
The surface morphology of nanoparticle was characterized by SEM imaging and it showed that the formulated nanoparticle have spherical shape with smooth surface (Figure 3).
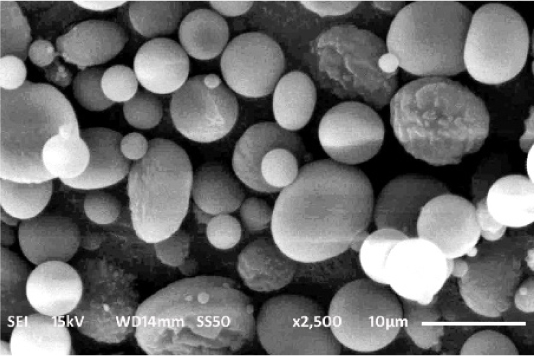
Figure 3:
SEM image of Remdesivir mucoadhesive nanoparticle.
The prepared nanoparticle having the size of 104.6 nm with PDI of 0.02 (Figure 4). Jacob D. Ramsey et al. developed remdesivir PLGA nanoparticle with the size range of 400-450 nm and PDI of about 0.20, which are greater than our formulation. The Zeta potential of the nanoparticle is a important factor for particle-particle aggregation24 and the prepared remdesivir nanoparticles showed -12.7 mV (Figure 5), which has more stable without any aggregation. Ketan Patel et al., prepared remdesivir liposome has zeta potential of -32±2 mV, which would higher potential than prepared nanoparticle. The ideal Zeta potential value of most stable nanoparticle would be in the range between ±10 to 30 mV.25 The entrapment of remdesivir in the nanoparticle showed 72.06±0.2% and its confirmed that satisfied quantity of drug would be reaches lung to prevent the viral replication.
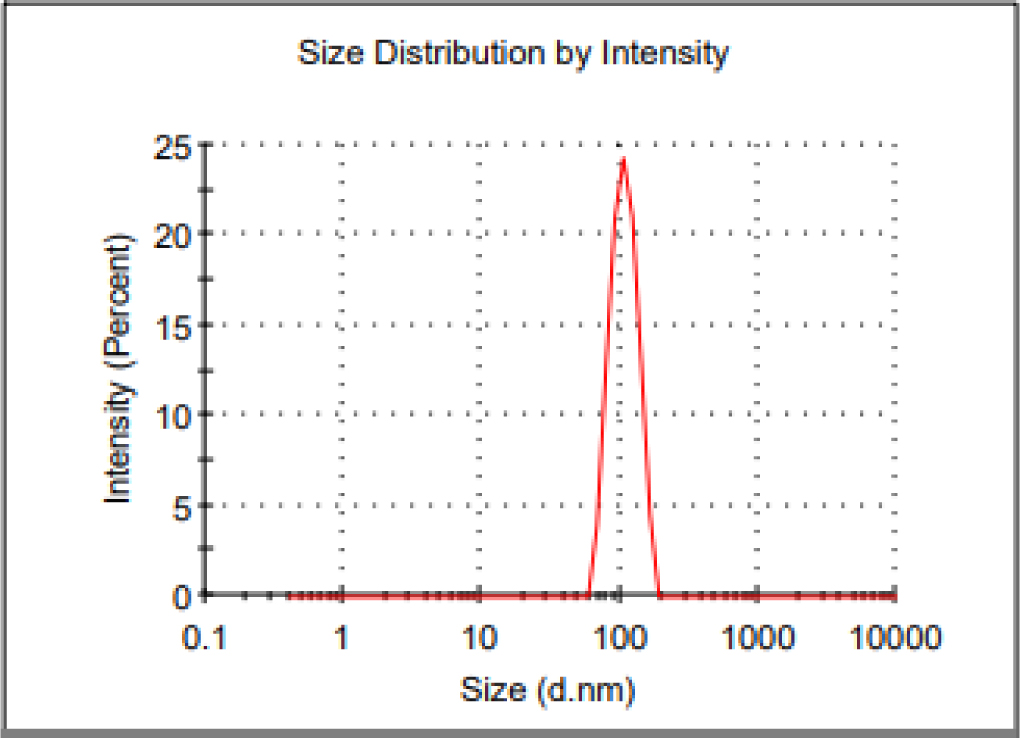
Figure 4:
Particle size of Remdesivir mucoadhesive nanoparticle.
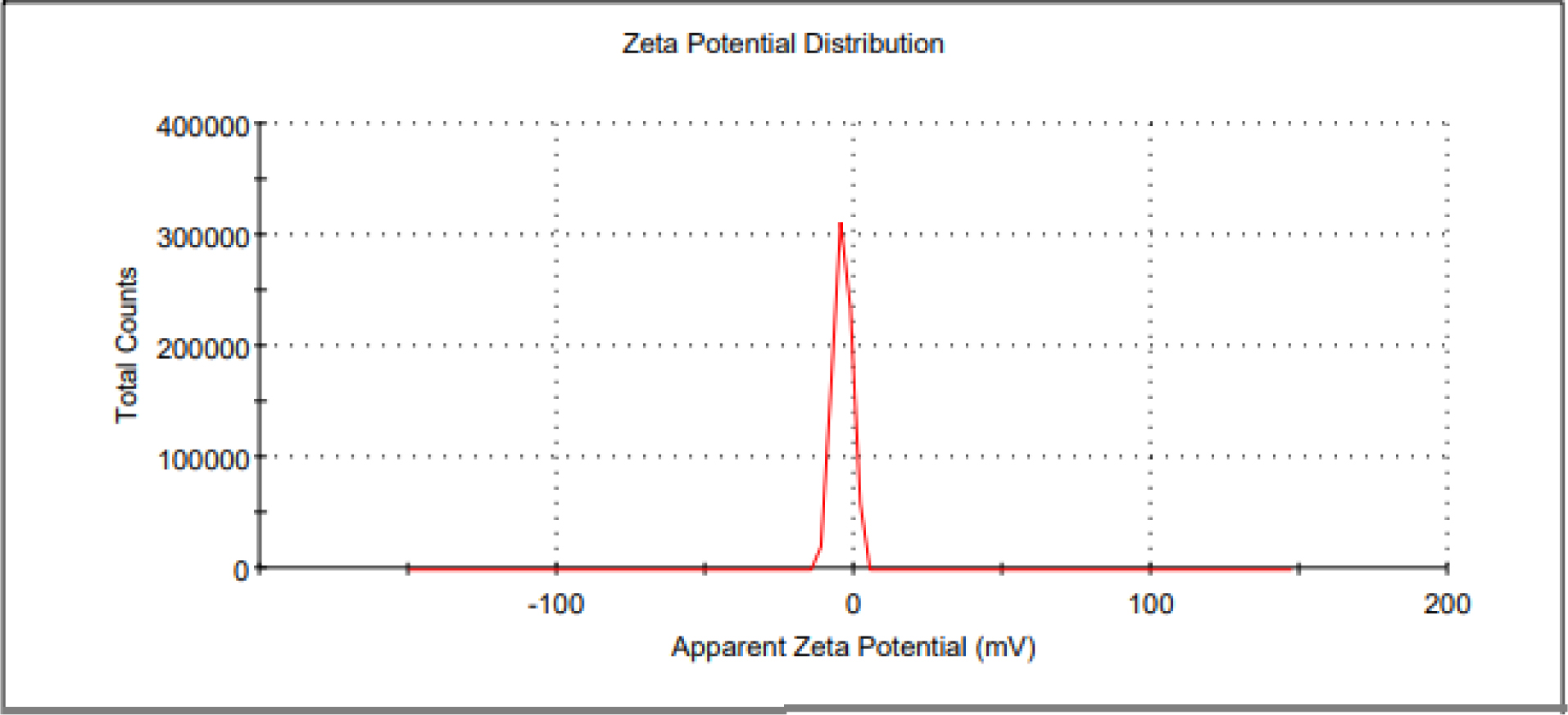
Figure 5:
Zeta potential of Remdesivir mucoadhesive nanoparticle.
The drug release profile of remdesivir mucoadhesive nanoparticle was evaluated by Cellulose dialysis tube and the released remdesivir was analyzed by HPLC technique. The precipitated mucoadhesive nanoparticle has strong matrix network structure of polymer and the remdesivir has been entrapped in the matrix, that showed significant sustained release. About 16.2±1.02% drug has release initially at 2 hr (Figure 6). At the end of 48 hr, significant amount (85.1±4.18%) of drug has release and it confirms that sustained release over the period and it stretches satisfied bioavailability. The release follows zero order kinetic mechanism (R2=0.9095) and the Higuchi’s equation linearity showed drug release would be diffusion mechanism. Peppa’s linearity showed that non-Fickian anomalous transport from polymeric matrix into diffusion medium.
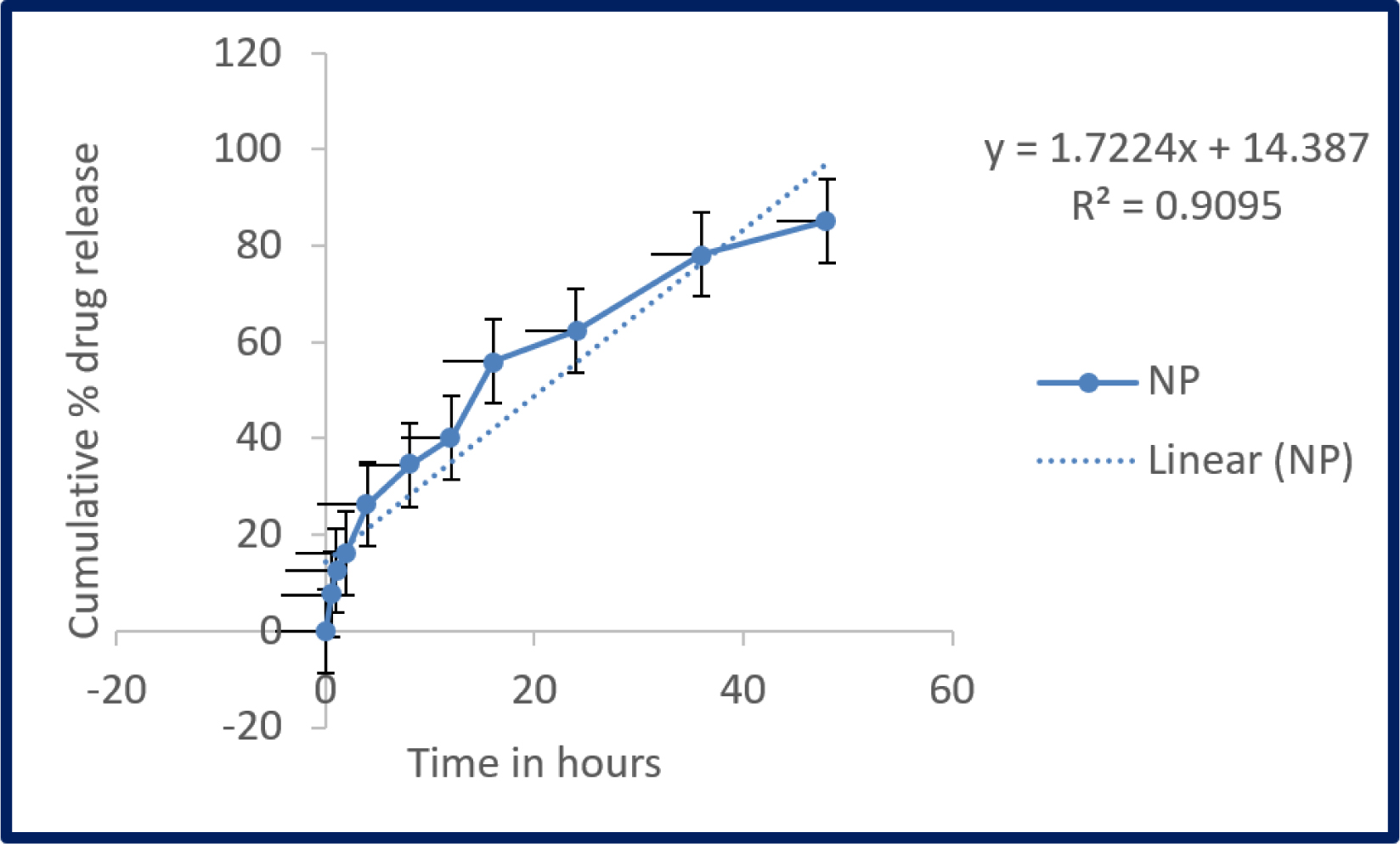
Figure 6:
In vitro release profile of Remdesivir mucoadhesive nanoparticle. Samples were analysed in triplicate (Mean ± SD).
Trans-epithelial electrical resistance of monolayer of cells in medium was calculated as 100% and the resistance was measured after incubating with nanoparticles, Calu-3 cells expressed 3±0.02% decline at 24 hr as compared with negative control (Figure 7).
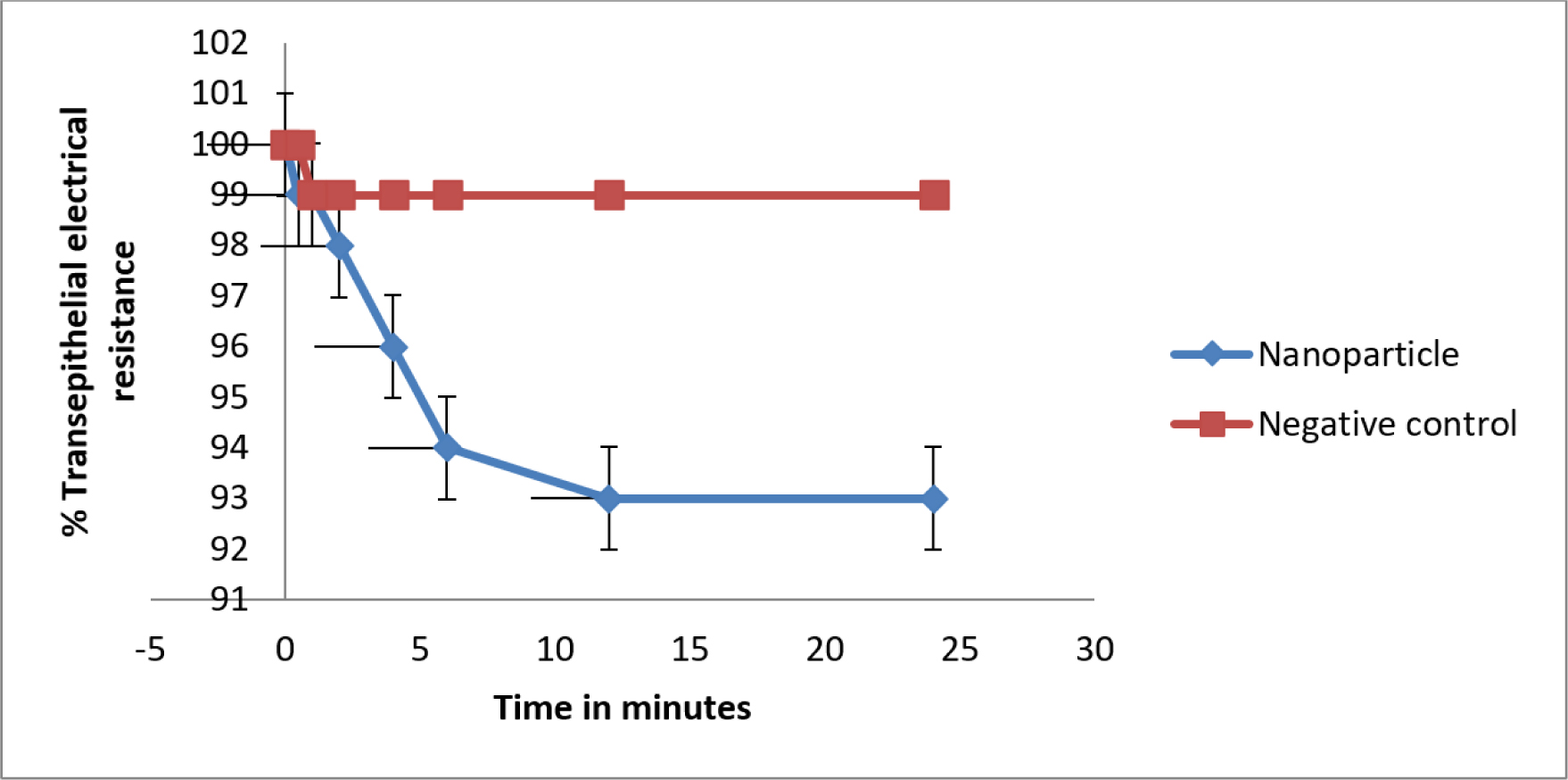
Figure 7:
Trans-epithelial electrical resistance of Remdesivir mucoadhesive nanoparticle compared with plain medium as negative control. Whereas the resistance of samples were measurements at triplicate (Mean ± SD)..
The DPI aerosol dispersion of remdesivir mucoadhesive nanoparticle was measured by using NGI certified by the USP. The Figure 8 shows that detectable deposition occurs on all stages, including the lowest one. The DPI aerosols dispersion of nanoparticle was exhibit significant deposition on stages demonstrated for middle and deep lung delivery. Remdesivir nanoparticle has showed higher deposit in stage 6 (55.14±8.70%) and lesser deposit in stage one (2.18±0.03%). The ED value are very high (87.02±1.38%) and it represents aerosolization efficiency and the Rf values was 86.18±0.14%. The FPF value is less than 50% (36.14±1.02%) and the marketed formulation of DPI in the range of 10 to 20%. The MMAD value of nanoparticle was 2.90±0.03, which is ideal for high lung deposition targeting of smaller airways (Values in the range 2.5 to 3) (Table 1).
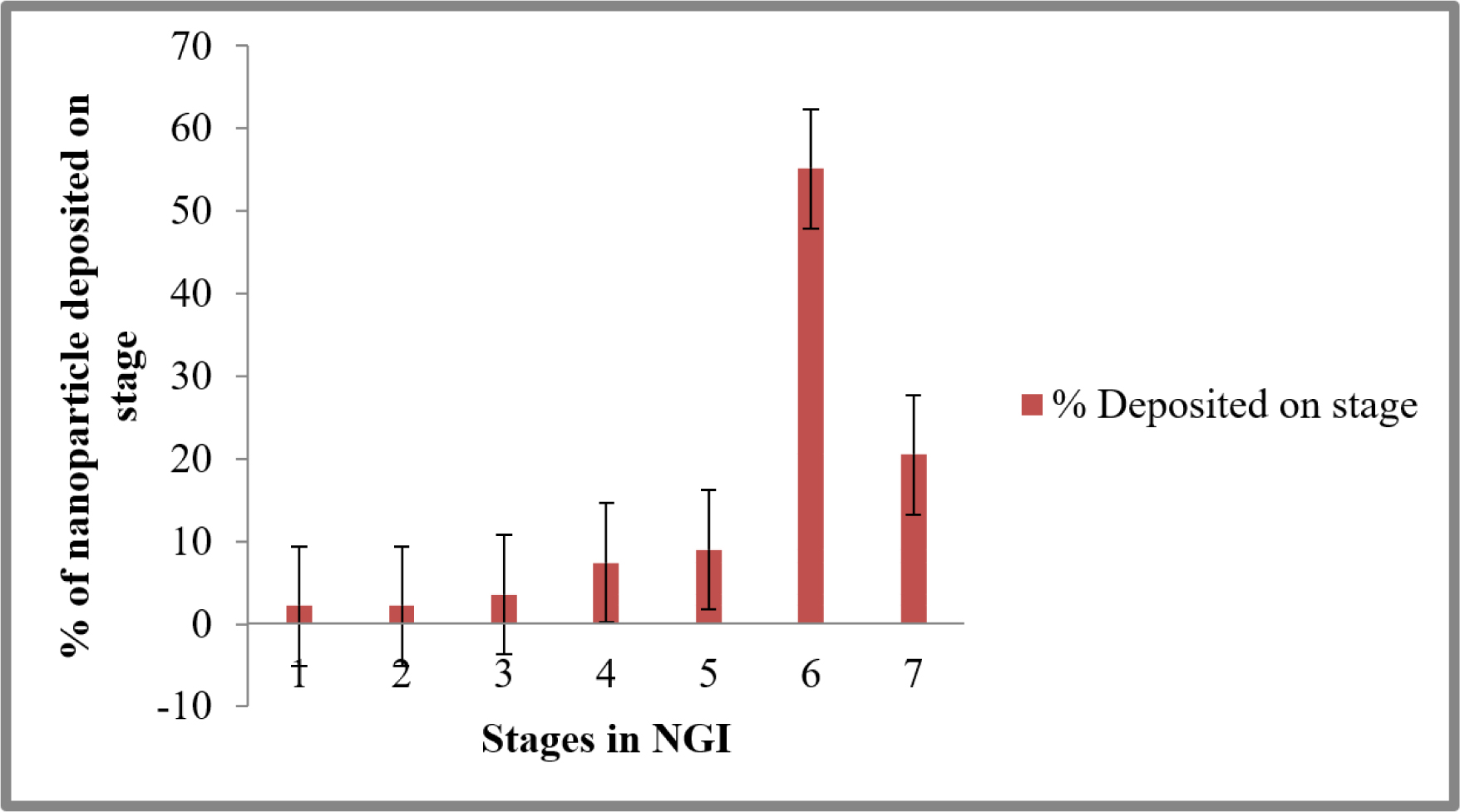
Figure 8:
DPI Aerosol dispersion performance test showed as percentage of Remdesivir deposited in every stage of next-generation impactor (Mean ± SD; n = 3). The cutoff diameter of stages in impactor as stage one (8.06 m); stage two (4.46 m); stage three (2.82 m); stage four (1.66 m); stage five (0.94 m); stage six (0.55 m); and stage seven (0.34 m).
Sl. No. | Aerosol performance parameters | Values (n=3) |
---|---|---|
1 | ED (%) | 87.02±1.38 |
2 | FPF (%) | 36.14±1.02 |
3 | RF (%) | 86.18±0.14 |
4 | MMAD (pm) | 2.90±0.03 |
5 | GSD | 2.08±0.02 |
The mucoadhesive nature of remdesivir nanoparticle would be compared with plain Chitosan and PLGA nanoparticles respectively (Figure 9). PLGA nanoparticle showed lowest binding strengh of 32.2±1.2% and Chitosan nanoparticle showed 54.6±5.08% compared to remdesivir nanoparticle, which had the greatest binding effectiveness of 77.1±6.7%.
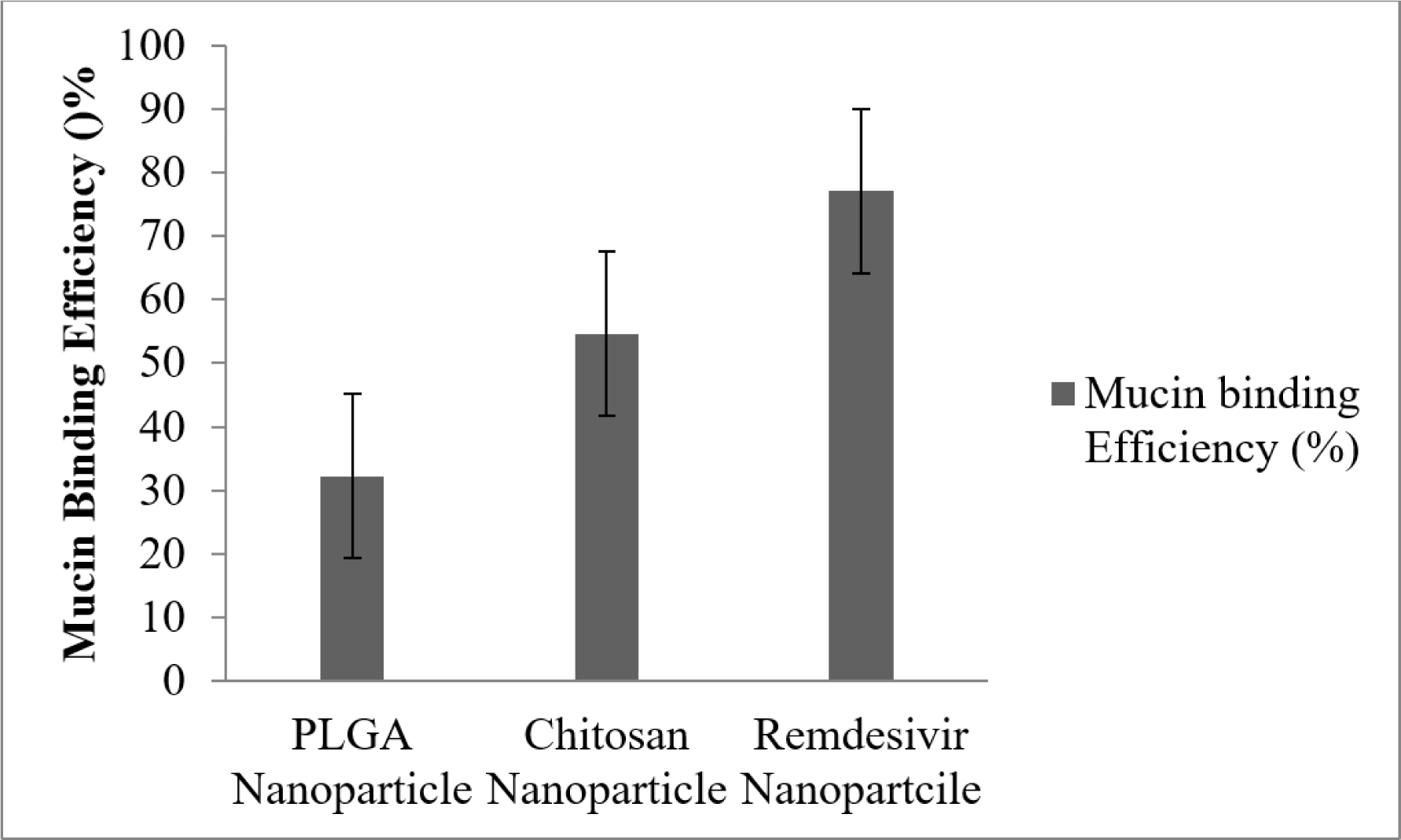
Figure 9:
Mucin binding efficiency of mucoadhesive nanoparticle. Whereas mucin concentrations were analysed in triplicate (Mean ± SD).
DISCUSSION
Pulmonary route of drug delivery is a well-secure technique for the management of pulmonary conditions such as COPD, Asthma, Lung infections and Cystic Fibrosis.26 Difficulties of pulmonic route has successfully managed by different inhalation devices namely, Dry Powder Inhalers (DPIs), Nebulizers, Metered Dose Inhalers (MDIs) and Soft-Mist Inhalers (SMIs).27 Out of which, DPI is a well-recognized device for the pulmonary delivery of pharmaceutical drugs.
Many studies have been tried in different methods for the preparation of mucoadhesive polymeric nanoparticle with their appropriateness for pulmonary delivery.28,29 It has been formulated by nanoprecipitation technique with the help of Ouzo effect.30 The effect has processed by organic solvent containing components were precipitated in aqueous solution to produced nanoparticle and this process would be enhanced by low frequency in the ultra-probe sonicator. when both the phases are mixed properly, the organic phase is back to back dispersed as drops within the non-solvent phase to produced nanoparticle precipitate. It caused by interfacial agitation and thermal variability in the mixture.31
In the remdesivir nanoparticle spectra has less intensed peaks, that referenced remdesivir is encapsulated as amorphous form. The drug loading and co-solvent type had no effect on the morphology of remdesivir in nanoparticle. The nanoparticle has smaller in size and it causes broaden peaks in the diffraction. The peaks have broadened by low number of crystalline planes and its turn to cause a disappearance of intensity in the diffraction patterns.32 During the nanoprecipitation, remdesivir was completely embedded in network structure of PLGA and Chitosan. In remdesivir nanoparticle, three sharp peaks were disappeared as it was with pure remdesivir, due to conversion of crystallinity to amorphous nature. Amorphous nature of remdesivir showed excellent absorption and drug release kinetics. When the nanoparticle administered to the biological fluid, the nano-sized particles were smoothly surrounded by bio-macromolecules, which would be modify all the biological characters and eliminate from biological system by opsonin action.33 The nanoprecipitation technique formed uniform shape and size of nanoparticles by Ouzo effect, which was enhanced by Ultra-sonication technique.
The nanoparticle size, Zeta potential and PDI of remdesivir nanoparticles were analyzed by Zetasizer. These properties could be the main factor in drug diffusion, permeation and stability of the nanoparticle.34 The negative zeta potential was higher in the nanoparticle preparation as a consequences of terminal carboxyl functional groups in the polymers. Lowering the negative zeta potential value directly propositional to the particle’s stability, hence the prepared mucoadhesive remdesivir nanoparticle was found to be stable. The % Entrapment efficiency would be depending of concentration of PLGA and Chitosan. Since, the multi-block copolymer shows more entrapment efficiency than polymeric chain. More hydrocarbon chain might be the reason for high entrapment efficiency.35 In vitro release of drug showed clearly that the low percentage of drug was release due to the formation of more solid wall around the drug by polyeric lacto-glycolic-acid linkage.24 It possesses the sustained release for an extended period of time.
Pulmonary epithelial integrity is significantly associated with permeation of virion to systemic circulation. High value of trans-epithelial electric resistance showed increased destruction of epithelial membrane integrity.36 However, remdesivir loaded mucoadhesive nanoparticles showed negligible difference compared with normal cells. The nanoparticles have prepared with biodegradable and biocompatible polymers such as PLGA and Chitosan and these polymers are not stimulating proinflammatory cytokines.
The NGI in conjunction with the DPI device displayed significant aerosol dispersion performance, indicating that the formed nanoparticles would be ideal for targeted distribution as aerosolized powders by DPI. This confirms the significant potential of DPI aerosols for effective deliver of remdesivir nanoparticle to deep airways in lungs.37
There are several well-established techniques for assessing mucoadhesion strength of dosage forms such as pills, gels and films. Texture analysis is one of the procedures used to measure the mucoadhesive strength of dosage form in mucous membranes. This approach, would be precise and robust, may not be appropriate for analyzing nanoparticles, microparticles, or other nanocarriers in the nano-size range.38 As the results, the emphasis has shifted to other methodologies and able measure the mucoadhesive strength by using spectral properties, viscosity, surface characteristics, diffusion coefficient and so on to evaluate their strength of nanoparticle with mucin. Spectroscopic investigation is the easiest approach for analyzing the interaction of nanoparticles with mucin.
This mucoadhesive nanoparticle has been prepared with combination of PLGA and Chitosan and these polymers has hydrogen bond with hydrophilic functional groups (-OH- and -NH2-) mucin and electrostatic attraction between the positive charge of polymers and the negative charge of sialic acid present in mucin.39 Despite the fact that all the nanoparticles showed mucous binding efficiency, which depends on type of the polymer charge and hydrogen bonding. The ideal characteristics of a mucoadhesive polymers would be rapid adherence from the mucosal layer without any change in their physical nature, minimize the interference of drug release, biodegradable without producing any toxic by-products and enhance the penetration of the active agents.40
CONCLUSION
We approached the delivery of remdesivir mucoadhesive nanoparticle by dry powder inhaler. In this attempt, the prepared nanoparticle have appropriate particle size to reach lungs and significant mucoadhesive strength on pulmonary tract with substaintial DPI dispersion properties. Therefore, remdesivir mucoadhesive nanoparticle loaded dry powder inhaler colud be the best and altenative route of adminstrtion during the pandemic suitation.
Cite this article
Vijayan V, Ravindran S, Sockalingam A. Dry Powder Inhaler Formulation of Remdesivir Mucoadhesive Nanoparticle for Respiratory Diseases. J Young Pharm. 2024;16(4):753-61.
AKNOWLEDGEMENT
This research work was supported by intramural research grant under seed money grant (Ref: Sbv/IRC/Seed Money/78/2021) of Sri Balaji Vidyapeeth Demeed University, Puducherry, India.
ABBREVIATIONS
SBECD: | Sulfobutylether-Beta-Cyclodextrin |
---|---|
DPI: | Dry Powder Inhaler |
PLGA: | Poly-lacto- Glycolic Acid |
UV: | Ultra Violet |
n: | Release Exponent |
R2: | Regression |
PDI: | Polydispersity index |
XRD: | X-ray Diffraction |
DSC: | Differential Scanning Calorimetry |
SEM: | Scanning Electron Microscope |
NGI: | Next Generation Impactor |
MMAD: | Mass Mean Aerodynamic Diameter |
GSD: | Geometric Standard Deviation |
FPF: | Fine Particle Fraction |
RF: | Respirable fraction |
ED: | Emission dose |
References
- Kwatra S, Taneja G, Nasa N. Alternative routes of drug administration- transdermal, Pulmonary and parenteral. Indo Glob J Pharm Sci. 2012;02(4):409-26. [CrossRef] | [Google Scholar]
- Parmar R, Patel H, Yadav N, Patidar M, Tyagi RK, Dalai SK, et al. Route of administration of attenuated sporozoites is instrumental in rendering immunity against Plasmodia infection. Vaccine. 2016;34(28):3229-34. [PubMed] | [CrossRef] | [Google Scholar]
- Ibrahim M, Verma R, Garcia-Contreras L. Inhalation drug delivery devices: technology update. Med Devices (Auckl). 2015;8:131-9. [PubMed] | [CrossRef] | [Google Scholar]
- Ghadiri M, Young PM, Traini D. Strategies to enhance drug absorption via nasal and pulmonary routes. Pharmaceutics. 2019;11(3):113 [PubMed] | [CrossRef] | [Google Scholar]
- Patil JS, Sarasija S. Pulmonary Drug Delivery Strategies: A concise, systematic review. Lung India. 2012;29(1):44-9. [PubMed] | [CrossRef] | [Google Scholar]
- Chandel A, Goyal AK, Ghosh G, Rath G. Recent advances in aerosolised drug delivery. Biomed Pharmacother. 2019;112:108601 [PubMed] | [CrossRef] | [Google Scholar]
- Thakur AK, Chellappan DK, Dua K, Mehta M, Satija S, Singh I, et al. Patented therapeutic drug delivery strategies for targeting pulmonary diseases. Expert Opin Ther Pat. 2020;30(5):375-87. [PubMed] | [CrossRef] | [Google Scholar]
- Smola M, Vandamme T, Sokolowski A. Nanocarriers as Pulmonary Drug Delivery Systems to treat and to diagnose respiratory and non respiratory diseases. Int J Nanomedicine. 2008;3(1):1-19. [PubMed] | [CrossRef] | [Google Scholar]
- Vinjamuri BP, Kotha AK, Kolte A, Haware RV, Chougule MB. Polymer applications in Pulmonary Drug Delivery. Appl Polym Drug Deliv. 2021:333-54. [CrossRef] | [Google Scholar]
- Kwatra S, Taneja G, Nasa N. Alternative routes of drug administration- transdermal, Pulmonary and parenteral. Indo Glob J Pharm Sci. 2012;02(4):409-26. [CrossRef] | [Google Scholar]
- De Wit E, Feldmann F, Cronin J, Jordan R, Okumura A, Thomas T, et al. Prophylactic and therapeutic remdesivir (GS-5734) treatment in the rhesus macaque model of MERS-COV infection. Proc Natl Acad Sci U S A. 2020;117(12):6771-6. [PubMed] | [CrossRef] | [Google Scholar]
- Grein J, Ohmagari N, Shin D, Diaz G, Asperges E, Castagna A, et al. Compassionate Use of Remdesivir for Patients with Severe Covid-19. N Engl J Med. 2020;382(24):2327-36. [PubMed] | [CrossRef] | [Google Scholar]
- Yang Z, Xiao D, Ling KH, Tarnowski T, Humeniuk R, Parmentier B, et al. The determination of sulfobutylether cyclodextrin sodium (SBECD) by LC-MS/MS and its application in REMDESIVIR pharmacokinetics study for pediatric patients. J Pharm Biomed Anal. 2022;212:114646 [PubMed] | [CrossRef] | [Google Scholar]
- Yasmeen R, Mobeen N, Chaudhry S. Covid-19 and cardiovascular diseases. Pak J Zool. 2021;53(5):1-9. [CrossRef] | [Google Scholar]
- Pallavi S, Patil SV, Patil SS, Shinde S. Preparation and evaluation of mucoadhesive nanoparticles of rosuvastatin. Indian J Pharm Sci. 2018;80(3) [CrossRef] | [Google Scholar]
- Tarhini M, Benlyamani I, Hamdani S, Agusti G, Fessi H, Greige-Gerges H, et al. Protein-based nanoparticle preparation via nanoprecipitation Method. Materials (Basel). 2018;11(3):394 [PubMed] | [CrossRef] | [Google Scholar]
- Kishore D, Prasad KR, Darapureddy C, Phani RS. Development and validation of a new HPLC bioanalytical internal standard method for the analysis of Remdesivirin Human plasma. Rasayan J Chem. 2022;14(4):2639-44. [CrossRef] | [Google Scholar]
- Li Y, Li M, Rantanen J, Yang M, Bohr A. Transformation of nanoparticles into compacts: A study on Plga and celecoxib nanoparticles. Int J Pharm. 2022;611:121278 [PubMed] | [CrossRef] | [Google Scholar]
- Braga GK, Oliveira WP. Manufacturing Drug Loaded chitosan microspheres by spray drying: development, characterization and potential use in dentistry. Drying Technol. 2007;25(2):303-10. [CrossRef] | [Google Scholar]
- Perotin JM, Adam D, Vella-Boucaud J, Delepine G, Sandu S, Jonvel AC, et al. Delay of airway epithelial wound repair in COPD is associated with airflow obstruction severity. Respir Res. 2014;15(1):151 [PubMed] | [CrossRef] | [Google Scholar]
- Yoshida H, Kuwana A, Shibata H, Izutsu KI, Goda Y. Comparison of aerodynamic particle size distribution between a next generation impactor and a cascade impactor at a range of flow rates. AAPS PharmSciTech. 2017;18(3):646-53. [PubMed] | [CrossRef] | [Google Scholar]
- Amin MK, Boateng JS. Enhancing stability and mucoadhesive properties of chitosan nanoparticles by surface modification with sodium alginate and polyethylene glycol for potential oral mucosa vaccine delivery. Mar Drugs. 2022;20(3):156 [PubMed] | [CrossRef] | [Google Scholar]
- Sahakijpijarn S, Moon C, Warnken ZN, Maier EY, DeVore JE, Christensen DJ, et al. Array. Int J Pharm X. 2021;3:100073 [PubMed] | [CrossRef] | [Google Scholar]
- Guadarrama-Escobar OR, Sánchez-Vázquez I, Serrano-Castañeda P, Chamorro-Cevallos GA, Rodríguez-Cruz IM, Sánchez-Padrón AY, et al. Development, characterization, optimization and evaluation of methacrylic acid-ethyl acrylate copolymer nanoparticles loaded with glibenclamide in diabetic rats for oral administration. Pharmaceutics. 2021;13(12):2023 [PubMed] | [CrossRef] | [Google Scholar]
- Kotakadi VS, Rao YS, Gaddam SA, Prasad TN, Reddy AV, Gopal DV, et al. Simple and rapid biosynthesis of stable silver nanoparticles using dried leaves of Catharanthus roseus. Linn G Donn and its anti microbial activity. Colloids Surf B Biointerfaces. 2013;105:194-8. [PubMed] | [CrossRef] | [Google Scholar]
- Diaz-Fuentes G, Hashmi HR, Venkatram S. Perioperative evaluation of patients with pulmonary conditions undergoing non-cardiothoracic surgery. Health Serv Insights. 2016(9s1;9):9-23. Suppl 1 [PubMed] | [CrossRef] | [Google Scholar]
- Haidl P, Heindl S, Siemon K, Bernacka M, Cloes RM. Inhalation device requirements for patients’ inhalation maneuvers. Respir Med. 2016;118:65-75. [PubMed] | [CrossRef] | [Google Scholar]
- Takeuchi H, Yamamoto H, Kawashima Y. Mucoadhesive nanoparticulate systems for Peptide Drug Delivery. Adv Drug Deliv Rev. 2001;47(1):39-54. [PubMed] | [CrossRef] | [Google Scholar]
- Mohammed MA, Syeda JT, Wasan KM, Wasan EK. An overview of chitosan nanoparticles and its application in non-parenteral drug delivery. Pharmaceutics. 2017;9(4):53 [PubMed] | [CrossRef] | [Google Scholar]
- Lepeltier E, Bourgaux C, Couvreur P. Nanoprecipitation and the “Ouzo effect”: application to drug delivery devices. Adv Drug Deliv Rev. 2014;71:86-97. [PubMed] | [CrossRef] | [Google Scholar]
- Feng Y, Ji X, Zhang Y, Muhammad MM, Zang D. Dynamics of acoustically levitated drops with ouzo effect. Sci Sin-Phys Mech Astron. 2021;51(5):054701 [CrossRef] | [Google Scholar]
- Ghose D, Patra CN, Ravi Kumar BV, Swain S, Jena BR, Choudhury P, et al. QBD-based formulation optimization and characterization of polymeric nanoparticles of cinacalcet hydrochloride with improved biopharmaceutical attributes. Turk J Pharm Sci. 2021;18(4):452-64. [PubMed] | [CrossRef] | [Google Scholar]
- Whittemore JH, Jones AT, Mendon SK, Rawlins JW. Investigation of ouzo effect colloid formation via organosilica nanoparticles. Colloid Polym Sci. 2015;293(9):2671-80. [CrossRef] | [Google Scholar]
- Acosta MF, Abrahamson MD, Encinas-Basurto D, Fineman JR, Black SM, Mansour HM, et al. Inhalable nanoparticles/microparticles of an AMPK and Nrf2 activator for targeted pulmonary drug delivery as dry powder inhalers. AAPS J. 2020;23(1):2 [PubMed] | [CrossRef] | [Google Scholar]
- Luo L, Liang S, Long M, Xu H. Influence of probe-sonication process on drug entrapment efficiency of liposomes loaded with a hydrophobic drug. Int J Polym Mater Polym Biomater. 2018;68:193-7. [CrossRef] | [Google Scholar]
- Fernández DA, Louzao MC, Vilariño N, Espiña B, Fraga M, Vieytes MR, et al. The kinetic, mechanistic and cytomorphological effects of palytoxin in human intestinal cells (Caco-2) explain its lower-than-parenteral oral toxicity. FEBS Journal. 2013;280(16):3906-19. [PubMed] | [CrossRef] | [Google Scholar]
- Hoe S, Traini D, Chan HK, Young PM. Measuring charge and mass distributions in dry powder inhalers using the Electrical Next Generation Impactor (ENGI). Eur J Pharm Sci. 2009;38(2):88-94. [PubMed] | [CrossRef] | [Google Scholar]
- Kolawole OM, Lau WM, Khutoryanskiy VV. Chitosan/β-glycerophosphate in situ gelling mucoadhesive systems for intravesical delivery of Mitomycin-C. Int J Pharm X. 2019;1:100007 [PubMed] | [CrossRef] | [Google Scholar]
- Sahakijpijarn S, Moon C, Warnken ZN, Maier EY, DeVore JE, Christensen DJ, et al. Array. Int J Pharm X. 2021;3:100073 [PubMed] | [CrossRef] | [Google Scholar]
- Bernkop-Schnürch A. Mucoadhesive polymers: basics, strategies and Trends. Concise Encyclopedia of Biomedical Polymers and Polymeric. Biomaterials. 2017:941-60. [CrossRef] | [Google Scholar]