ABSTRACT
Glioblastoma Multiforme (GBM) is still one of the most aggressive and resistant brain tumours, and current treatment options provide only minimal improvements in survival. Effective drug delivery is severely hampered by the complexity of GBM, which is defined by its heterogeneity and the Blood-Brain Barrier (BBB). The most recent developments in precision drug delivery technology are examined in this study to address these issues and enhance treatment outcomes for GBM patients. We discuss various innovative approaches, including nanoparticle-based systems, bioconjugates, ligand-targeted therapies, and gene delivery platforms. These technologies are specifically engineered to enhance drug penetration across the BBB, target tumour-specific pathways, and minimise off-target effects, thereby potentially revolutionizing GBM treatment. The review also addresses the challenges associated with clinical translation, including regulatory hurdles, safety concerns, and the need for scalable manufacturing processes. This review endeavours to offer a comprehensive overview of the potential of precision drug delivery to facilitate more personalised and effective GBM therapies in the near future by emphasising the most promising strategies and emergent trends in the field.
INTRODUCTION
Glioblastoma Multiforme (GBM) is the predominant and deadliest primary brain tumour in adults, comprising almost 80% of all brain tumours and close to 60% of malignant brain tumours.1 GBM, distinguished by its fast growth, invasiveness, and resistance to standard therapy, poses a difficult challenge in neuro-oncology. The average survival rate for patients diagnosed with GBM is dismally low, typically ranging from 12 to 15 months, even with aggressive treatment.2 This grim prognosis is primarily due to the tumor’s highly infiltrative nature, making complete surgical resection nearly impossible and leading to inevitable recurrence. The World Health Organisation classifies GBM as a grade IV astrocytoma, which indicates its high aggressiveness.3 The histological hallmarks of the tumour include angiogenesis, the growth of aberrant blood vessels, and necrotic regions surrounded by densely packed tumour cells, known as pseudo palisading necrosis.4 These characteristics and genetic and molecular heterogeneity contribute to the tumor’s resilience against standard therapies.5 The current recommended treatment for GBM involves extensive surgical removal of the tumour, followed by simultaneous administration of radiation therapy and chemotherapy using Temozolomide (TMZ). Several intrinsic constraints contribute to the poor prognosis for GBM, even with current therapies. Surgical resection, while critical for reducing tumor burden, cannot eradicate the infiltrative tumor cells that extend into surrounding healthy brain tissue, leading to frequent local recurrences.6 Radiation therapy aimed at controlling the residual tumor is often hindered by the radio resistance of GBM cells. At the same time, TMZ, the most commonly used chemotherapeutic agent, is only moderately effective and is usually met with tumor resistance over time.7
Moreover, the BBB, a selective and protective barrier formed by endothelial cells lining the brain’s capillaries, poses a significant obstacle to effective chemotherapy. The BBB impedes the passage of most drugs into the brain, constraining the effective dosage of chemotherapeutic treatments within the tumour microenvironment. This challenge is exacerbated by the molecular heterogeneity of GBM, with different tumor regions exhibiting distinct genetic and phenotypic profiles, leading to varied responses to treatment and further complicating therapeutic efforts.8,9 In light of the constraints of existing treatment options, there is an immediate requirement for innovative drug delivery methods that can surmount the obstacles presented by the biology of GBM. One of the most significant hurdles in treating GBM is the BBB, which not only limits drug delivery but also plays a role in maintaining the tumor’s microenvironment, contributing to therapy resistance. It is imperative to develop innovative drug delivery systems that bypass or penetrate the BBB to enhance the therapeutic outcomes for GBM patients.10
The heterogeneity of GBM, both at the genetic and cellular levels, further underscores the need for precision medicine approaches in drug delivery. Precision medicine is adapting treatment to the specific characteristics of each patient’s tumour, including its molecular and genetic makeup. Precision medicine can maximise therapeutic benefit while reducing side effects by focusing on specific pathways and mechanisms that cause GBM in various patients.11
Innovative drug delivery methods, including liposomes, viral vectors, and nanoparticles, are being investigated to enhance the transport and targeting of therapeutic agents to the GBM site. These systems can be designed to penetrate the Blood-Brain Barrier (BBB) and specifically transport medications to cancerous cells while avoiding damage to healthy brain tissue and minimising overall toxicity. Additionally, advances in imaging and diagnostic technologies are aiding in the precise characterization of GBM, enabling more accurate targeting of therapies.12,13
Interdisciplinary partnerships among neuroscientists, oncologists, bioengineers, and material scientists propel the fast-evolving field of precise medication delivery for GBM. This review attempts to give a thorough overview of the advancements made in targeting GBM and the possible influence these developments may have on bettering patient outcomes in the future by looking at the state-of-the-art and developing technologies. This review critically analyzes the strengths and limitations of various precision drug delivery approaches, evaluates their performance in preclinical and clinical studies, and discusses the challenges that must be overcome to translate these promising technologies into clinical practice. By synthesizing the latest research and identifying key trends, we hope to offer insights into the future direction of GBM treatment and inspire further innovation in this critical area of neuro-oncology.
CHALLENGES IN BRAIN DRUG DELIVERY
Blood-Brain Barrier
The Blood-Brain Barrier (BBB) is a discerning partition that segregates the flowing blood from the extracellular fluid in the brain. From a structural standpoint, it consists of endothelial cells closely connected by tight junctions. Pericytes, astrocyte end feet, and a basement membrane surround these cells. The primary purpose of the BBB is to safeguard the brain against potentially detrimental compounds in the bloodstream while permitting the flow of vital nutrients and gases. Nevertheless, this characteristic of selective permeability presents a considerable obstacle to the transportation of drugs to the brain. Several therapeutic treatments, particularly those that are giant molecules and hydrophilic medicines, cannot penetrate the (BBB), which restricts their effectiveness in treating brain tumours such as GBM. The BBB employs various mechanisms to limit drug delivery, including efflux transporters such as P-glycoprotein (P-gp) and Multidrug Resistance-associated Proteins (MRPs), which actively pump out drugs that manage to penetrate the endothelial cells. Additionally, the BBB’s high expression of metabolic enzymes further degrades therapeutic agents before they can reach their target within the brain.14,15 One of the main goals of GBM research is to overcome the BBB. Techniques such as focused ultrasound-assisted BBB rupture, drug administration via nanoparticles, and the creation of BBB-penetrating peptides are all being investigated.16 Despite these efforts, achieving consistent and safe drug delivery across the BBB remains a formidable challenge.
Tumor Microenvironment
The Tumour Microenvironment (TME) in GBM plays a critical role in the resistance to therapy and the failure of drug treatments. It is distinguished by a dense extracellular matrix, hypoxia, and immunological suppression, all of which make treatment of this malignancy more challenging. Hypoxia, or low oxygen levels within the tumor, is a common feature of GBM and promotes resistance to both chemotherapy and radiotherapy. Hypoxic conditions lead to the activation of Hypoxia-Inducible Factors (HIFs), which in turn upregulate survival pathways in tumor cells and alter drug metabolism, further diminishing the efficacy of treatments. Moreover, hypoxia-induced angiogenesis contributes to the abnormal vasculature of GBM, exacerbating the difficulty of drug delivery.17,18
The immune-suppressive environment within GBM also hinders therapeutic efforts. Tumour-associated macrophages and Myeloid-Derived Suppressor Cells (MDSCs) within the TME facilitate immune evasion and tumour proliferation. Additionally, the release of immunosuppressive cytokines and the presence of regulatory T cells create a barrier to effective immunotherapy. The dense Extracellular Matrix (ECM) in GBM, composed of collagen and hyaluronic acid proteins, also acts as a physical barrier to drug penetration. This ECM can trap therapeutic agents, preventing them from reaching the tumor cells, and contribute to drug resistance through interactions with cellular receptors that activate survival pathways.19,20
Heterogeneity of Glioblastoma
The remarkable genetic and phenotypic heterogeneity of GBM is recognised both within the same tumour (intra-tumoral heterogeneity) and between distinct tumours (inter-tumoral heterogeneity).21,22 This diversity poses a significant challenge for targeted drug delivery. Genetic heterogeneity in GBM includes variations in key signalling pathways, such as the PI3K/AKT/mTOR, p53, and RAS/MAPK pathways, which can lead to differing responses to treatment among patients. Even within a single tumor, different regions may exhibit distinct genetic profiles, contributing to varied drug sensitivities and the development of resistant subclones.23,24 Phenotypic heterogeneity further complicates treatment, as GBM cells can transition between different states, such as the proneural, mesenchymal, and classical subtypes. This plasticity allows tumor cells to adapt to therapeutic pressures, leading to treatment failure and recurrence.25 The implications of GBM heterogeneity for drug delivery are profound. Targeted therapies that may be effective against one tumor subtype or genetic variant may be ineffective against others, necessitating combination therapies or the development of personalized treatment approaches. However, the challenge lies in accurately identifying and targeting the diverse and evolving populations of tumor cells within a patient’s GBM.
CONVENTIONAL THERAPIES
Surgery, radiation therapy, and chemotherapy have traditionally been the primary methods used to treat glioblastoma. Surgical resection is a procedure that seeks to eliminate the tumour by extracting as much of it as feasible. In contrast, radiation therapy employs powerful beams of energy to obliterate cancer cells. Chemotherapeutic agents, administered systemically, seek to inhibit tumour cells’ rapid division and growth. Though these conventional approaches have seen some success, they often fall short of effectively eradicating the entirety of glioblastoma, leading to recurrence and poor long-term outcomes. The non-specific targeting of radiation and chemotherapy, as well as the intrusive nature of surgery, can cause serious side effects that negatively affect a patient’s quality of life. As researchers continue to delve deeper into the complex biology of glioblastoma, new, more targeted therapeutic strategies are emerging that hold promise for improving the prognosis for those battling this devastating disease.26,27
LIMITATIONS OF CONVENTIONAL THERAPIES
Conventional cancer therapies, such as chemotherapy and radiation, often fall short when it comes to treating glioblastoma. These approaches struggle to target the elusive tumor cells effectively, leading to suboptimal outcomes. Furthermore, the systemic toxicity associated with many anti-cancer drugs can cause debilitating side effects, compromising the patient’s quality of life. Equally concerning is the growing problem of drug resistance, where glioblastoma cells develop the ability to evade the cytotoxic effects of these treatments, rendering them increasingly ineffective over time.28,29 To improve the prognosis for individuals battling this devastating form of brain cancer and surmount the limitations of conventional therapies, it is evident that new and innovative strategies are required.
INNOVATIVE DRUG DELIVERY TECHNOLOGIES FOR GLIOBLASTOMA
The need for precision drug delivery in the treatment of GBM, an aggressive form of brain cancer, has become increasingly apparent. A targeted approach to treatment holds the promise of improved therapeutic efficacy, allowing for more effective elimination of tumor cells while minimizing the detrimental impact on healthy surrounding tissues. By delivering drugs directly to the site of the tumor, the risk of systemic side effects can be significantly reduced, enhancing the patient’s quality of life and increasing the chances of successful outcomes. This targeted approach is a significant breakthrough in the battle against this incurable illness, providing renewed hope to glioblastoma patients.
Nanoparticles are a viable solution for improving the delivery of therapeutic medicines to glioblastoma tumours. These nano-scale carriers can encapsulate a diverse array of drugs, facilitating more precise delivery and sheltering them from degradation.30
Nanoparticles can be preferentially accumulated within the tumour by utilising the Enhanced Permeability and Retention (EPR) effect, resulting in superior tumour targeting and increased drug concentrations at the disease site.31 This targeted approach helps minimize off-target toxicity, potentially improving the therapeutic index and patient outcomes. Several categories of nanoparticles, such as liposomes, polymeric nanoparticles, and metallic nanoparticles, have been investigated to treat GBM. Figure 1 depicts the schematic portrayal of different drug delivery carriers.
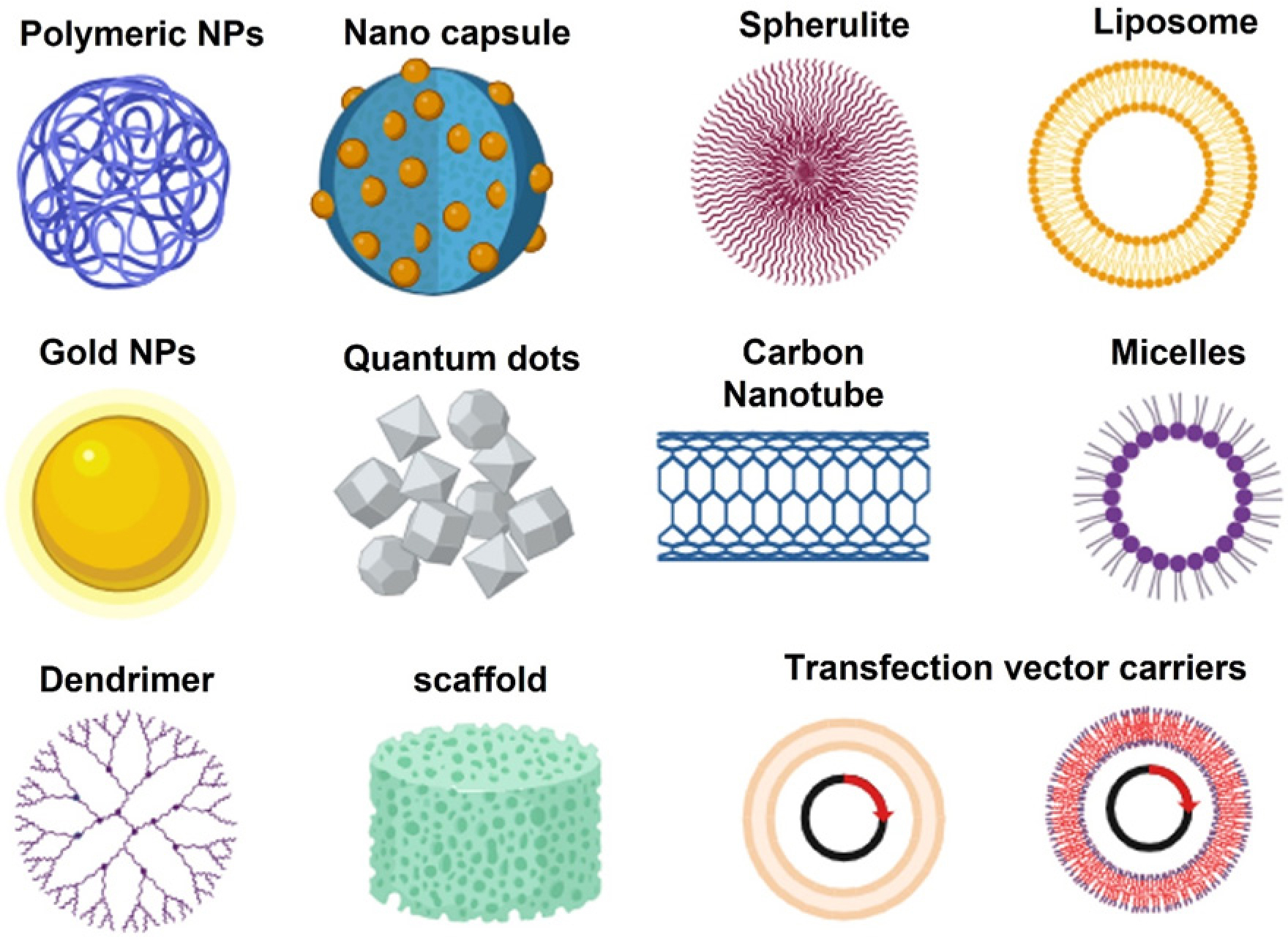
Figure 1:
Schematic representation of different types of drug delivery carriers.
Polymeric Nanoparticles
Polymeric Nanoparticles (PNPs) have garnered significant attention as sophisticated nanocarriers capable of addressing the formidable challenges posed by gliomas, particularly in enhancing the precision and efficacy of therapeutic interventions. Among the myriad polymeric structures, Poly (Lactic-co-Glycolic Acid) (PLGA) nanoparticles stand out due to their biodegradability and biocompatibility.32 PLGA-based systems facilitate the encapsulation of diverse therapeutic moieties, ensuring controlled release kinetics, thus minimizing off-target effects and systemic toxicity.33 Maria et al. developed transferrin-conjugated PLGA nanoparticles for the co-delivery of temozolomide and bortezomib to GBM cells. Dual drug combination promoted a synergistic effect, and the conjugation of transferrin enhanced the targeting ability of the nanoparticle.34 Conversely, chitosan-based nanoparticles leverage their intrinsic mucoadhesive properties and ability to transiently disrupt tight junctions within the BBB, thus augmenting CNS penetration.35 Polyethylene Glycol (PEG)-coated nanoparticles, through the process of PEGylation, exhibit prolonged systemic circulation by evading the mononuclear phagocyte system, thereby enhancing their propensity to accumulate within the tumour microenvironment.36 Polysorbate 80-coated nanoparticles, meanwhile, exploit receptor-mediated endocytosis mechanisms, thus facilitating BBB translocation, which is pivotal in achieving efficacious glioma targeting.37
The burgeoning field of personalised nanomedicine will likely influence the future trajectory of polymeric nanoparticles in glioma therapy. Advances in genomic and proteomic profiling of gliomas may pave the way for the development of tailor-made nanoparticle systems customised to individual tumours’ molecular and phenotypic characteristics. This could potentially enhance therapeutic outcomes by aligning treatment strategies with the unique biological context of each patient’s tumor.
Additionally, the integration of imaging modalities with therapeutic nanoparticles could yield theranostic platforms that treat and provide real-time feedback on therapeutic efficacy, thereby enabling dynamic adjustments to treatment regimens. Such innovations could herald a new era of precision oncology in glioma management, offering hope for improved patient outcomes in this notoriously challenging malignancy.
Lipid-based nanoparticles for Drug Delivery
Liposomes and Solid Lipid Nanoparticles (SLNs) have emerged as promising drug delivery systems in treating gliomas, offering innovative solutions to the challenges posed by the BBB and the need for targeted therapeutic interventions. Liposomes are nanoscale vesicles characterized by one or more phospholipid bilayers encapsulating an aqueous core, capable of incorporating both hydrophilic and hydrophobic drugs.38 Their surface can be functionalized with targeting ligands such as monoclonal antibodies, peptides, or aptamers, facilitating receptor-mediated endocytosis by glioma cells. Liposomes enhance BBB permeation and accomplish tumour-specific targeting due to their structural versatility, which improves drug accumulation at the tumour site while minimising systemic toxicity.39 For instance, Cen et al. devised a liposomal delivery vector with dual-targeting capabilities specifically designed to transport the anticancer drug doxorubicin for treating glioma. SS31, a peptide of tiny size, has exhibited the capacity to target mitochondria and effectively cross the BBB selectively.40 However, liposomes face challenges such as physicochemical instability in the systemic circulation, which can lead to premature drug release, and the complexities involved in their large-scale manufacturing.
On the other hand, SLNs are composed of a solid lipid core stabilized by surfactants, providing a protective matrix for the encapsulated drug, particularly lipophilic agents. SLNs offer advantages such as enhanced stability and controlled drug release, maintaining therapeutic drug levels over extended periods. Their biocompatibility, owing to the use of physiological lipids, further underscores their potential as safer alternatives for repeated administration. Nevertheless, SLNs are constrained by limitations in drug loading capacity, especially for hydrophilic drugs, and the risk of burst release, where a significant fraction of the drug is rapidly released, potentially causing toxicity.41,42 Both liposomes and SLNs have been extensively investigated for delivering chemotherapeutic agents, small interfering RNA (siRNA), and other therapeutic molecules to glioma cells.43
Their ability to enhance drug accumulation within the tumor microenvironment, coupled with the reduction of systemic side effects associated with traditional therapies, positions these nanocarriers as promising candidates in glioma management. However, to fully harness their clinical potential, ongoing research must address challenges related to optimizing stability, drug loading efficiency, and targeting specificity, thereby paving the way for more effective glioma therapies.
Gold and Metal-Based Nanoparticles
The dual functionality of gold and other metal-based nanoparticles in drug delivery and imaging is currently being investigated. These nanoparticles can be engineered to deliver therapeutic agents directly to GBM cells and to function as contrast agents for imaging techniques such as CT or MRI scans.44 Metal nanoparticles’ unique optical and electrical capabilities allow for accurate imaging and real-time monitoring of medication distribution in the brain. Metal-based nanoparticles (silver, iron oxide zinc oxide, and platinum nanoparticles) offer several advantages in targeting GBM and overcoming drug resistance. Their surface can be functionalized with targeting ligands, such as antibodies or peptides, to enhance their specificity for GBM cells.45
Metal nanoparticles can also be utilised to deliver drugs that block particular resistance mechanisms, like DNA repair pathways or efflux pumps. These nanoparticles also have the potential to be used in combination with other therapies, such as photothermal or photodynamic therapy, to improve treatment outcomes.46 The future of metal-based nanoparticles for glioma treatment lies in their potential for personalized medicine, combining enhanced targeting and multifunctionality with improved safety profiles to overcome challenges like BBB penetration and toxicity.
Bioconjugates and Ligand-Targeted Delivery
A targeted method to treating GBM, Antibody-Drug Conjugates (ADCs) combine the cytotoxicity of chemotherapy drugs with the specificity of monoclonal antibodies. The mechanism involves the binding of the ADC to particular antigens on the surface of GBM cells, followed by internalisation and release of the cytotoxic drugs. ADCs are designed to minimize off-target effects and enhance the therapeutic index of anticancer drugs.47
Several ADCs are under investigation for GBM therapy. For example, Depatux-M, an Antibody-Drug Conjugate (ADC) that specifically targets the EGFR VIII mutation frequently observed in GBM, has demonstrated encouraging results in both preclinical and initial clinical investigations.48 Other ADCs targeting integrins and other GBM-specific antigens are also being developed, with the goal of improving drug delivery to the tumor while sparing healthy brain tissue.
Peptide and Aptamer-Based Targeting
Aptamers and peptides are tiny compounds that have a high affinity and selectivity for binding to particular antigens or receptors on GBM cells. In order to deliver medications directly to the tumour, these targeting ligands can be conjugated to nanoparticles or used as standalone agents. Peptide-based targeting has the advantage of being relatively easy to synthesize and modify, while aptamers offer high binding specificity and stability.49 Peptide and aptamer-based targeting systems have shown promise in preclinical studies for GBM treatment. Peptide-conjugated nanoparticles have been used to deliver drugs like temozolomide and doxorubicin, with enhanced targeting and reduced toxicity. Aptamer-based systems are being explored for the delivery of siRNA and other therapeutic agents, offering a highly specific approach to targeting GBM cells and overcoming the challenges of drug resistance.50
Gene and RNA-Based Delivery Systems
Small interfering RNA (siRNA) and microRNA (miRNA) are powerful tools for gene silencing, capable of targeting and downregulating specific oncogenes involved in GBM progression. Nanoparticles designed to deliver siRNA or miRNA can cross the BBB and specifically target tumor cells, reducing the expression of oncogenic proteins and inhibiting tumor growth. siRNA and miRNA delivery systems have shown promising results in preclinical models of GBM. For example, nanoparticles delivering siRNA against genes like EGFR or VEGF have demonstrated significant tumor regression and prolonged survival in animal models. The ability to target multiple pathways involved in GBM progression makes siRNA and miRNA delivery a promising strategy for combination therapy.51–53
CRISPR/Cas9 Delivery
A revolution in the field of genetic engineering has been brought about by the CRISPR/Cas9 gene-editing technology, which has the ability to fix genetic mutations or disrupt oncogenes in Glio Blastoma Multiforme syndrome (GBM). The distribution of CRISPR/Cas9 components to the brain presents a number of important problems; nevertheless, nanoparticles and viral vectors are now being developed to assist efficient delivery and gene editing in GBM cells. Recent advances in CRISPR/Cas9 delivery systems have shown potential for GBM treatment. For instance, nanoparticles encapsulating CRISPR/Cas9 components have been used to knock out oncogenes like IDH1 and PTEN in GBM models, leading to tumor regression. While still in the early stages of development, CRISPR/Cas9-based therapies could offer a novel approach to treating GBM by targeting the genetic drivers of the disease.54–56
Exosome and Extracellular Vesicle-Mediated Delivery
Exosomes and Extracellular Vesicles (EVs), naturally occurring nanostructures secreted by cells, possess the intrinsic ability to shuttle bioactive molecules such as proteins, RNA, and lipids between cells. These vesicles can be bioengineered to encapsulate therapeutic cargos, including pharmacological agents, siRNA, and CRISPR/Cas9 constructs, facilitating their traversal across the BBB to specifically target GBM cells. Leveraging exosome and EV-mediated delivery systems presents a novel and sophisticated approach to personalized medicine for GBM. These vesicles, when derived from autologous cells, offer the dual advantages of minimizing immunogenicity and optimizing the delivery of tailored therapies. In preclinical investigations, exosomes laden with anticancer agents or gene-editing components have demonstrated robust antitumor efficacy, underscoring their potential as an avant-garde platform for drug delivery in GBM treatment.57–59
Glioblastoma treatment has the potential to be significantly improved through the development of novel drug delivery systems, which hold a great deal of promise. Nanoparticle-based systems, bioconjugates, ligand-targeted delivery, and gene/RNA-based approaches offer targeted and efficient drug delivery to the tumor site, potentially overcoming the challenges posed by BBB and drug resistance. Continued research and clinical development of these technologies could pave the way for more effective and personalized therapies for GBM, ultimately improving patient outcomes. Table 1 provides an overview of different drug delivery systems, including their targeting mechanisms, advantages, and challenges
Types of drug delivery system | Targeting mechanism | Advantages | challenges |
---|---|---|---|
Polymeric nanoparticles | Passive targeting (EPR effect) | Controlled drug release, reduced systemic toxicity | Heterogeneous EPR effect, potential toxicity |
Lipid nanoparticles | Ligand-receptor targeting | Enhanced drug solubility and stability | Limited brain penetration, potential immune response |
Metal-based nanoparticles | Active targeting (Ligand functionalised) | Targeted delivery, potential for hyperthermia, High surface area | Long-term toxicity, potential accumulation |
Dendrimers | Passive/active | High drug loading capacity, precise targeting | Complex synthesis, potential cytotoxicity |
Bioconjugate/ligand targeted | Ligand-targeted delivery | High specificity, minimal off-target effects | Complex manufacturing, potential for resistance |
Peptide and Aptamer | Ligand-receptor targeting, Nucleic acid aptamers for molecular recognition | High specificity, ability to penetrate cells, low immunogenicity | Short half-life, stability issues, delivery challenges |
Gene and RNA | Viral vector-mediated targeting | Direct correction of genetic mutations | Immune response, integration issues, delivery efficiency, stability of RNA molecules |
CRISPR/Cas9 Delivery | Gene editing, Targeted knockout | Precision targeting, potential for long-term cure | Off-target effects, delivery challenges |
Exosome and Extracellular Vesicle-Mediated Delivery | Natural targeting ability | Biocompatibility, low immunogenicity | Isolation and purification challenges |
CLINICAL TRANSLATION AND CHALLENGES
Novel Drug Delivery Systems (NDDS) for GBM are currently under intense investigation, with multiple clinical trials assessing their efficacy and safety. One of the prominent strategies being explored is the use of nanoparticles, including liposomes, dendrimers, and polymeric nanoparticles, created to bypass the BBB and deliver therapeutic agents directly to the tumor site. Clinical trials like NCT03603379 are evaluating the use of nanoliposomes loaded with doxorubicin, a potent chemotherapeutic agent, showing promising results in preclinical models.60
Another innovative approach involves implantable devices that release drugs over time, such as the Gliadel® wafer, which has shown success in delivering carmustine directly to the post-surgical resection cavity in GBM patients.61 These trials have reported improved survival rates and reduced systemic toxicity compared to traditional delivery methods. However, setbacks such as limited patient recruitment and variations in drug delivery efficiency due to tumor heterogeneity have hindered some trials, highlighting the complexity of translating these technologies from bench to bedside.
FUTURE PERSPECTIVES
As the field of GBM treatment evolves, several emerging trends in drug delivery are poised to revolutionize the therapeutic landscape. Advances in nanotechnology are leading to the development of multi-functional nanoparticles capable of simultaneous diagnosis and therapy, known as theranostic agents. Another promising avenue is the exploration of bioengineered delivery systems, such as exosomes and other extracellular vesicles, which offer a biocompatible and highly targeted means of delivering therapeutics to GBM cells. The possibility to overcome the current constraints of GBM treatment lies in these developments and in the ongoing improvement of currently available technology. Furthermore, the overall safety profile of GBM therapies could be enhanced, and the risk of adverse effects could be reduced through the development of more biocompatible and patient-specific delivery systems.
CONCLUSION
In conclusion, the landscape of drug delivery for GBM is undergoing rapid transformation, driven by advances in nanotechnology, bioengineering, and drug discovery. While significant challenges remain in the clinical translation of these innovations, the potential benefits for patients are substantial. Continued research and collaboration among interdisciplinary teams will be required to overcome the limitations to clinical application and fully realise the potential of these technologies. The future of GBM treatment lies in the successful convergence of these cutting-edge approaches, offering hope for more effective and personalized therapies in the fight against this devastating disease.
Cite this article:
Rajendran AT, Vadakkepushpakath AN. Targeting Glioblastoma: Advances in Precision Drug Delivery. J Young Pharm. 2024;16(4):697-705.
ACKNOWLEDGEMENT
We acknowledge NITTE (Deemed to be University), Mangaluru, for providing all the technical support for the smooth conduction of the research work.
ABBREVIATIONS
GBM | Glioblastoma multiforme |
---|---|
BBB | Blood-brain barrier |
TMZ | Temozolomide |
P-gp | P-glycoprotein |
MRPs | Multidrug resistance-associated proteins |
TME | Tumor-microenvironment |
HIFs | Hypoxia-inducible factors |
MDSCs | Myeloid-derived suppressor cells |
ECM | Extracellular matrix |
EPR | Enhanced permeability and retention |
PNPs | Polymeric nanoparticles |
PLGA | Poly (lactic-co-glycolic acid) |
CNS | Central nervous system |
PEG | Polyethylene glycol |
SLNs | Solid lipid nanoparticles |
CT | Computed tomography |
MRI | Magnetic resonance imaging |
DNA | Deoxyribonucleic acid |
ADCs | Antibody-drug conjugates |
EGFR | Epidermal growth factor receptor |
siRNA | Small interfering RNA |
miRNA | microRNA |
VEGF | Vascular endothelial growth factor |
NDDS | Novel drug delivery systems |
EVs | Extracellular vesicles |
RNA | Ribonucleic acid |
CRISPR/ Cas9 | Clustered regularly interspaced short palindromic repeats |
IDH1 | Isocitrate dehydrogenase 1 |
PTEN | Phosphatase and tensin homolog |
References
- Hanif F, Muzaffar K, Perveen K, Malhi SM, Simjee ShU. Glioblastoma multiforme: a review of its epidemiology and pathogenesis through clinical presentation and treatment. Asian Pac J Cancer Prev. 2017;18(1):3-9. [PubMed] | [CrossRef] | [Google Scholar]
- Luo YH, Wang K, Zhan L, Zeng F, Zheng J, Chen S, et al. β3GNT9 as a prognostic biomarker in glioblastoma and its association with glioblastoma immune infiltration, migration and invasion. Front Oncol. 2023;13:1214413 [PubMed] | [CrossRef] | [Google Scholar]
- Louis DN, Perry A, Wesseling P, Brat DJ, Cree IA, Figarella-Branger D, et al. The 2021 WHO Classification of Tumors of the central nervous system: a summary. Neuro Oncol. 2021;23(8):1231-51. [PubMed] | [CrossRef] | [Google Scholar]
- Kumar P, Li J, Surulescu C. Multiscale modeling of glioma pseudopalisades: contributions from the tumor microenvironment. J Math Biol. 2021;82(6):1-45. [CrossRef] | [Google Scholar]
- Yang K, Wu Z, Zhang H, Zhang N, Wu W, Wang Z, et al. Glioma targeted therapy: insight into future of molecular approaches. Mol Cancer. 2022;21(1):39 [PubMed] | [CrossRef] | [Google Scholar]
- Lee JH, Wee CW. Treatment of adult gliomas: A current update. Brain Neurorehabil. 2022;15(3):e24 [PubMed] | [CrossRef] | [Google Scholar]
- Ali MY, Oliva CR, Noman AS, Allen BG, Goswami PC, Zakharia Y, et al. Radioresistance in glioblastoma and the development of radiosensitizers. Cancers (Basel). 2020;12(9):1-29. [PubMed] | [CrossRef] | [Google Scholar]
- Narsinh KH, Perez E, Haddad AF, Young JS, Savastano L, Villanueva-Meyer JE, et al. Strategies to improve drug delivery across the blood–brain barrier for glioblastoma. Curr Neurol Neurosci Rep. 2024;24(5):123-39. [PubMed] | [CrossRef] | [Google Scholar]
- Liu D, Dai X, Tao Z, Zhou H, Hong W, Qian H, et al. Advances in blood–brain barrier-crossing nanomedicine for anti-glioma. Cancer Nanotechnol. 2023;14(1):1-32. [CrossRef] | [Google Scholar]
- Iyer K, Saini S, Bhadra S, Kulavi S, Bandyopadhyay J. Precision medicine advancements in glioblastoma: A systematic review. Biomedicine. 2023;13(2):1-13. [PubMed] | [CrossRef] | [Google Scholar]
- Mathew EN, Berry BC, Yang HW, Carroll RS, Johnson MD. Delivering therapeutics to glioblastoma: overcoming biological constraints. Int J Mol Sci. 2022;23(3) [PubMed] | [CrossRef] | [Google Scholar]
- Lai G, Wu H, Yang K, Hu K, Zhou Y, Chen X, et al. Progress of nanoparticle drug delivery system for the treatment of glioma. Front Bioeng Biotechnol. 2024;12:1403511 [PubMed] | [CrossRef] | [Google Scholar]
- Li J, Wang W, Wang J, Cao Y, Wang S, Zhao J, et al. Viral gene therapy for glioblastoma multiforme: A promising hope for the current Dilemma. Front Oncol. 2021;11:678226 [PubMed] | [CrossRef] | [Google Scholar]
- Wang Y, Zhang F, Xiong N, Xu H, Chai S, Wang H, et al. Remodelling and treatment of the blood–brain barrier in glioma. Cancer Manag Res. 2021;13:4217-32. [PubMed] | [CrossRef] | [Google Scholar]
- Sharma HS, Muresanu DF, Castellani RJ, Nozari A, Lafuente JV, Tian ZR, et al. Pathophysiology of blood–brain barrier in brain tumor. Novel therapeutic advances using nanomedicine. Int Rev Neurobiol. 2020;151:1-66. [PubMed] | [CrossRef] | [Google Scholar]
- Barzegar-Fallah A, Gandhi K, Rizwan SB, Slatter TL, Reynolds JN. Harnessing ultrasound for targeting drug delivery to the brain and breaching the blood–brain tumour barrier. Pharmaceutics. 2022;14(10):2231 [PubMed] | [CrossRef] | [Google Scholar]
- Feldman L. Hypoxia within the glioblastoma tumor microenvironment: a master saboteur of novel treatments. Front Immunol. 2024;15:1384249 [PubMed] | [CrossRef] | [Google Scholar]
- Park JH, Lee HK. Current understanding of hypoxia in glioblastoma multiforme and its response to immunotherapy. Cancers (Basel). 2022;14(5) [PubMed] | [CrossRef] | [Google Scholar]
- Zhang X, Zhao L, Zhang H, Zhang Y, Ju H, Wang X, et al. The immunosuppressive microenvironment and immunotherapy in human glioblastoma. Front Immunol. 2022;13:1003651 [PubMed] | [CrossRef] | [Google Scholar]
- Lin H, Liu C, Hu A, Zhang D, Yang H, Mao Y, et al. Understanding the immunosuppressive microenvironment of glioma: mechanistic insights and clinical perspectives. J Hematol Oncol. 2024;17(1):31 [PubMed] | [CrossRef] | [Google Scholar]
- Petrilli LL, Fuoco C, Palma A, Pasquini L, Pericoli G, Grabovska Y, et al. Inter and intra-tumor heterogeneity of paediatric type diffuse high-grade gliomas revealed by single-cell mass cytometry. Front Oncol. 2022;12:1016343 [PubMed] | [CrossRef] | [Google Scholar]
- Becker AP, Sells BE, Haque SJ, Chakravarti A. Tumor heterogeneity in glioblastomas: from light microscopy to molecular pathology. Cancers (Basel). 2021;13(4):1-25. [PubMed] | [CrossRef] | [Google Scholar]
- Parker NR, Hudson AL, Khong P, Parkinson JF, Dwight T, Ikin RJ, et al. Intratumoral heterogeneity identified at the epigenetic, genetic and transcriptional level in glioblastoma. Sci Rep. 2016;6(1):22477 [PubMed] | [CrossRef] | [Google Scholar]
- Degl’Innocenti A, Leo di N, Ciofani G. Genetic hallmarks and heterogeneity of glioblastoma in the single-cell omics era. Adv Ther (Weinh). 2020;3(1) [PubMed] | [CrossRef] | [Google Scholar]
- Fayzullin A, Sandberg CJ, Spreadbury M, Saberniak BM, Grieg Z, Skaga E, et al. Phenotypic and expressional heterogeneity in the invasive glioma cells. Transl Oncol. 2019;12(1):122-33. [PubMed] | [CrossRef] | [Google Scholar]
- Rios SA, Oyervides S, Uribe D, Reyes AM, Fanniel V, Vazquez J, et al. Emerging therapies for glioblastoma. Cancers. 2024;16(8):1485 [PubMed] | [CrossRef] | [Google Scholar]
- Karami Fath M, Babakhaniyan K, Anjomrooz M, Jalalifar M, Alizadeh SD, Pourghasem Z, et al. Recent advances in glioma cancer treatment: conventional and epigenetic realms. Vaccines. 2022;10(9) [PubMed] | [CrossRef] | [Google Scholar]
- Angom RS, Nakka NM, Bhattacharya S. Advances in glioblastoma therapy: an update on current approaches. Brain Sci. 2023;13(11):1536 [PubMed] | [CrossRef] | [Google Scholar]
- Cruz JV, Batista C, Afonso BH, Alexandre-Moreira MS, Dubois LG, Pontes B, et al. Obstacles to glioblastoma Treatment Two decades after temozolomide. Cancers (Basel). 2022;14(13):3203 [PubMed] | [CrossRef] | [Google Scholar]
- Marei HE. Multimodal targeting of glioma with functionalized nanoparticles. Cancer Cell Int. 2022;22(1):265 [PubMed] | [CrossRef] | [Google Scholar]
- Caro C, Avasthi A, Paez-Muñoz JM, Pernia Leal M, García-Martín ML. Passive targeting of high-grade gliomas via the EPR effect: a closed path for metallic nanoparticles?. Biomater Sci. 2021;9(23):7984-95. [PubMed] | [CrossRef] | [Google Scholar]
- Madani F, Esnaashari SS, Webster TJ, Khosravani M, Adabi M. Polymeric nanoparticles for drug delivery in glioblastoma: state of the art and future perspectives. J Control Release. 2022;349:649-61. [PubMed] | [CrossRef] | [Google Scholar]
- Caraway CA, Gaitsch H, Wicks EE, Kalluri A, Kunadi N, Tyler BM, et al. Polymeric nanoparticles in brain cancer therapy: a review of current approaches. Polymers (Basel). 2022;14(14) [PubMed] | [CrossRef] | [Google Scholar]
- Ramalho MJ, Torres ID, Loureiro JA, Lima J, Pereira MC. Transferrin-conjugated PLGA nanoparticles for co-delivery of temozolomide and bortezomib to glioblastoma cells. ACS Appl Nano Mater. 2023;6(15):14191-203. [PubMed] | [CrossRef] | [Google Scholar]
- Eslahi M, Dana PM, Asemi Z, Hallajzadeh J, Mansournia MA, Yousefi B, et al. The effects of chitosan-based materials on glioma: recent advances in its applications for diagnosis and treatment. Int J Biol Macromol. 2021;168:124-9. [PubMed] | [CrossRef] | [Google Scholar]
- Gajbhiye KR, Pawar A, Mahadik KR, Gajbhiye V. Pegylated nanocarriers: A promising tool for targeted delivery to the brain. Colloids Surf B Biointerfaces. 2020;187:110770 [PubMed] | [CrossRef] | [Google Scholar]
- Formica ML, Real DA, Picchio ML, Catlin E, Donnelly RF, Paredes AJ, et al. On a highway to the brain: a review on nose-to-brain drug delivery using nanoparticles. Appl Mater Today. 2022;29:101631 [CrossRef] | [Google Scholar]
- Raju R, Abuwatfa WH, Pitt WG, Husseini GA. Liposomes for the treatment of brain cancer—a review. Pharmaceuticals (Basel). 2023;16(8) [PubMed] | [CrossRef] | [Google Scholar]
- Kawak P, Sawaftah NM, Pitt WG, Husseini GA. Transferrin-targeted liposomes in glioblastoma therapy: a review. Int J Mol Sci. 2023;24(17) [PubMed] | [CrossRef] | [Google Scholar]
- Cen J, Dai X, Zhao H, Li X, Hu X, Wu J, et al. Doxorubicin-loaded liposome with the function of “killing two birds with one stone” against glioma. ACS Appl Mater Interfaces. 2023;15(40):46697-709. [PubMed] | [CrossRef] | [Google Scholar]
- Jnaidi R, Almeida AJ, Gonçalves LM. Solid lipid nanoparticles and nanostructured lipid carriers as smart drug delivery systems in the treatment of glioblastoma multiforme. Pharmaceutics. 2020;12(9):1-19. [PubMed] | [CrossRef] | [Google Scholar]
- Ak G, Ünal A, Karakayalı T, Özel B, Selvi Günel N, Hamarat Şanlıer Ş, et al. Brain-targeted, drug-loaded solid lipid nanoparticles against glioblastoma cells in culture. Colloids Surf B Biointerfaces. 2021;206:111946 [PubMed] | [CrossRef] | [Google Scholar]
- Teng XQ, Qu J, Li GH, Zhuang HH, Qu Q. Small interfering RNA for gliomas treatment: overcoming hurdles in delivery. Front Cell Dev Biol. 2022;10:824299 [PubMed] | [CrossRef] | [Google Scholar]
- Norouzi M. Gold nanoparticles in glioma theranostics. Pharmacol Res. 2020;156:104753 [PubMed] | [CrossRef] | [Google Scholar]
- Allen NC, Chauhan R, Bates PJ, O’Toole MG. Optimization of tumor targeting gold nanoparticles for glioblastoma applications. Nanomaterials (Basel). 2022;12(21) [PubMed] | [CrossRef] | [Google Scholar]
- Gawel AM, Singh R, Debinski W. Metal-based nanostructured therapeutic strategies for glioblastoma treatment—an update. Biomedicines. 2022;10(7) [PubMed] | [CrossRef] | [Google Scholar]
- Parakh S, Nicolazzo J, Scott AM, Gan HK. Antibody-drug conjugates in glioblastoma – is there a future for them?. Front Oncol. 2021;11:718590 [PubMed] | [CrossRef] | [Google Scholar]
- Lassman AB, Pugh SL, Wang TJ, Aldape K, Gan HK, Preusser M, et al. Depatuxizumab mafodotin in EGFR-amplified newly diagnosed glioblastoma: A phase III randomized clinical trial. Neuro Oncol. 2023;25(2):339-50. [PubMed] | [CrossRef] | [Google Scholar]
- Doherty C, Wilbanks B, Khatua S, Maher LJ. Aptamers in neuro-oncology: an emerging therapeutic modality. Neuro Oncol. 2024;26(1):38-54. [PubMed] | [CrossRef] | [Google Scholar]
- Nuzzo S, Brancato V, Affinito A, Salvatore M, Cavaliere C, Condorelli G, et al. The role of RNA and DNA aptamers in glioblastoma diagnosis and therapy: A systematic review of the literature. Cancers (Basel). 2020;12(8):1-17. [PubMed] | [CrossRef] | [Google Scholar]
- Sanati M, Afshari AR, Ahmadi SS, Jamialahmadi T, Sahebkar A. Application of RNA-based therapeutics in glioma: a review. Prog Mol Biol Transl Sci. 2024;204:133-61. [PubMed] | [CrossRef] | [Google Scholar]
- Mirzaei S, Mahabady MK, Zabolian A, Abbaspour A, Fallahzadeh P, Noori M, et al. Small interfering RNA (siRNA) to target genes and molecular pathways in glioblastoma therapy: current status with an emphasis on delivery systems. Life Sci. 2021;275:119368 [PubMed] | [CrossRef] | [Google Scholar]
- Teng XQ, Qu J, Li GH, Zhuang HH, Qu Q. Small interfering RNA for gliomas treatment: overcoming hurdles in delivery. Front Cell Dev Biol. 2022;10:824299 [PubMed] | [CrossRef] | [Google Scholar]
- Ruan W, Jiao M, Xu S, Ismail M, Xie X, An Y, et al. Brain-targeted CRISPR/Cas9 nanomedicine for effective glioblastoma therapy. J Control Release. 2022;351:739-51. [PubMed] | [CrossRef] | [Google Scholar]
- Kang X, Wang Y, Liu P, Huang B, Zhou B, Lu S, et al. Progresses, challenges, and prospects of CRISPR/Cas9 gene-editing in glioma studies. Cancers (Basel). 2023;15(2) [PubMed] | [CrossRef] | [Google Scholar]
- Begagić E, Bečulić H, Đuzić N, Džidić-Krivić A, Pugonja R, Muharemović A, et al. CRISPR/ Cas9-mediated gene therapy for glioblastoma: A scoping review. Biomedicines. 2024;12(1):238 [PubMed] | [CrossRef] | [Google Scholar]
- Musatova OE, Rubtsov YP. Effects of glioblastoma-derived extracellular vesicles on the functions of immune cells. Front Cell Dev Biol. 2023;11:1060000 [PubMed] | [CrossRef] | [Google Scholar]
- Indira Chandran V, Gopala S, Venkat EH, Kjolby M, Nejsum P. Extracellular vesicles in glioblastoma: a challenge and an opportunity. npj Precis Oncol. 2024;8(1):103 [PubMed] | [CrossRef] | [Google Scholar]
- Yang S, Sun Y, Liu W, Zhang Y, Sun G, Xiang B, et al. Exosomes in glioma: unraveling their roles in progression, diagnosis, and therapy. Cancers (Basel). 2024;16(4) [PubMed] | [CrossRef] | [Google Scholar]
- Kasenda B, König D, Manni M, Ritschard R, Duthaler U, Bartoszek E, et al. Targeting immunoliposomes to EGFR-positive glioblastoma. ESMO Open. 2022;7(1):100365 [PubMed] | [CrossRef] | [Google Scholar]
- Iuchi T, Inoue A, Hirose Y, Morioka M, Horiguchi K, Natsume A, et al. Long-term effectiveness of Gliadel implant for malignant glioma and prognostic factors for survival: 3-year results of a postmarketing surveillance in Japan. Neurooncol Adv. 2022;4(1):vdab189 [PubMed] | [CrossRef] | [Google Scholar]