ABSTRACT
Background
Gemcitabine (GEM), a Biopharmaceutical Classification System (BCS) class-III drug, holds promise for non-small cell lung cancer treatment. Utilizing lipid-based nanoparticles for targeted delivery has proven effective in cancer therapy. Biotin receptors, overexpressed in various solid tumors, offer a specific target for enhanced chemotherapy efficacy while minimizing side effects. Hence, this study aimed to formulate biotin-coated Nanostructured Lipid Carriers (NLCs) with Gemcitabine can be delivered to specific tumors by electrostatic attraction.
Materials and Methods
Nanostructured Lipid Carrier loaded Gemcitabine was formulated using Oleic acid and Glyceryl monostearate as lipids, with Tetronic 1107 as the surfactant. Formulations were optimized using a 2-factor, 3-level full factorial design approach with Design Expert software. Size of particle, Zeta potential, Polydispersity index, Encapsulation efficiency, Scanning Electron Microscopy, phase contrast microscopy, drug release and in vitro cytotoxicity against A549 cells were assessed for characterization.
Results
The optimized Gemcitabine-loaded Nanostructured Lipid Carrier formulation exhibited a particle diameter of 194.5 nanometer (nm) and Biotin-Gemcitabine- Nanostructured Lipid Carrier showed a size of 203.1 nanometer. In vitro drug release efficiency of about 95.125% during 8 hr for Gemcitabine-Nanostructured Lipid Carrier and 96.04% during 24 hr for Biotin-Gemcitabine-Nanostructured Lipid Carrier, suggesting prolonged circulation for the latter. Cytotoxicity assays revealed significant reduction in cell viability and induction of cytotoxic activity Gemcitabine-loaded Nanostructured Lipid Carrier-biotin at low concentrations in A549 cells.
Conclusion
The developed biotin coated Nanostructured Lipid Carrier present a promising strategy for targeted delivery of Gemcitabine to Non-Small Cell Lung Cancer (NSCLC). These nanocarriers could provide a targeted and efficient delivery platform to tumor cells. Further in vivo investigations are warranted to validate their safety and therapeutic effectiveness.
INTRODUCTION
Lung Cancer (LC) remains a formidable adversary in the realm of oncology, boasting a survival rate of less than a year and a distressingly high mortality rate. Within its classification lie two predominant types: small cell and Non-Small Cell Lung Cancer (NSCLC).1 Although numerous chemotherapeutic agents such as gemcitabine, paclitaxel, docetaxel are accessible, their effectiveness in treating LC has been unreliable at most. The widespread resistance to conventional drugs coupled with their inherent toxicity present formidable challenges in the treatment landscape, urging the need for novel therapeutic avenues.2,3
Gemcitabine (2,2-difluoro-2-deoxycytidine) is a primary drug for treating NSCLC, with FDA approval and extensive clinical application.4 Gemcitabine functions as a cell cycle-specific antimetabolite, exerting its cytotoxic effects by phosphorylating into its active form within DNA. This process inhibits tumor proliferation by arresting cells in the G1/S phase.5 Despite its potential, gemcitabine’s efficacy is curtailed by its nonspecific distribution and limited availability within malignant tissues. This underscores the need to develop innovative delivery strategies to improve therapeutic outcomes.
The advent of nanoparticle technology, with dimensions spanning 1 to 1000 nm, heralds a paradigm shift in therapeutic delivery systems, promising enhanced bioavailability and selectivity of anticancer agents.6,7 Nanostructured Lipid Carriers (NLCs) represent a pinnacle in this domain, featuring a unique solid-liquid matrix encapsulating an aqueous core within a lipid bilayer. This innovative formulation confers superior drug entrapment, stability and loading capacity,8 distinguishing NLCs are leading the way in cancer therapy.9–11
Targeted nanocarrier-based strategies offer a beacon of hope in augmenting drug deposition within tumor microenvironments while mitigating systemic toxicity.12 By leveraging ligand-receptor interactions, active targeting affords precise localization of therapeutic payloads to malignant cells, sparing healthy tissues from collateral damage.13 Biotin, a vital co-enzyme in cellular metabolism, emerges as a promising ligand owing to its high affinity for overexpressed biotin receptors on tumor cell surfaces,14–20 thus facilitating receptor-mediated endocytosis and selective drug delivery.21
Cancer cells often exhibit elevated expression of specific receptors, creating avenues for cytotoxic agents to targeted delivery into tumors.22 Several substances have been used as “targeting moieties” to create drug conjugates that target tumors, including hyaluronic acid, aptamers, polyunsaturated fatty acids, folic acid, transferrin and monoclonal antibodies.23
Cancer cells with high proliferation rates have elevated requirements for specific vitamins, leading to an increase in vitamin receptors on their surface.24,25 These receptors are crucial for tumor imaging, identification and targeted drug delivery. While folate receptors have been extensively studied for these purposes, biotin receptors have recently gained attention. Biotin, known to stimulate cellular growth, is notably more abundant in tumors than in normal tissues.24 Recent research indicates that biotin receptors are significantly more prevalent than folate or vitamin B-12 receptors in various cancer cell types, including leukemia (L1210FR), ovarian (Ov2008, ID8), colon (Colo-26), mastocytoma (P815), lung (M109), renal (RENCA, RD0995) and breast (4T1, JC, MMT06056) cancer cell lines.24,25
This study aimed to engineer NLC formulations utilizing a judicious blend of solid and liquid lipids via high-speed homogenization to deliver gemcitabine to NSCLC. Employing a 23 full factorial design, formulation was optimized and subsequently explored the augmentation of gemcitabine delivery through biotin-coated NLC. Comprehensive analysis, including zeta potential, PdI, particle size, entrapment efficiency, morphological features and release kinetics were conducted to evaluate the effectiveness. By elucidating the synergistic interplay between nanostructured lipid carriers and targeted biotin moiety, this study aims to pave the way for precision NSCLC therapy, offering renewed hope in the battle against this formidable malignancy.
MATERIALS AND METHODS
Materials
Gemcitabine was procured from PVR life sciences (Hyderabad, India). GMS, Biotin were obtained from Loba chemie Pvt. Ltd., (Mumbai, India). Oleic acid is obtained from Rankem Laboratory. Tetronic 1107 was obtained from BASF corporation. Dialysis membrane (MW cut off 12000-14000 DA, 2.4 nm pore size). All other components employed in the experiment were analytical-grade chemicals.
Selection of appropriate lipids
Liquid and solid lipids were chosen based on the maximum solubility of GEM in each type. 2 mL of Eppendorf tubes were taken and filled with 1 mL of liquid lipid (including Ethyl Oleate, Capmul MCM, Oleic Acid, Labrafac PG, Maiscine CC, Soyabean oil and Cinnamon oil) and each tube received an excess of GEM and then agitated in a shaker incubator at 37°C and 100 rpm for 48 hr. Similarly, 1g of melted solid lipid (maintained at 5°C above its melting point) such as Cetyl Palmitate, Cetostearyl alcohol, GMS, Precirol ATO, Palmitic Acid and Stearic Acid were stirred with surplus GEM. Saturation solubility of GEM was determined by observing the mixture’s transparency, followed by centrifugation at 5000 rpm for 15 min. GEM concentration in supernatant was separated and analyzes at 267 nm using UV-visible spectroscopy (UV 1650 PC Shimadzu) after appropriate dilution.26
Determination of Binary Lipid phase
Solid to liquid lipids ratio was determined based on the melting point of the binary lipid mixture. The mixture was melted using a magnetic stirrer at 5°C above the melting point of the solid lipid, stirred for an hour at 200 rpm and then cooled to room temperature to solidify. Various ratios of specific solid and liquid lipids ranging from 90:10 to 10:90 were prepared. The melting points of the solidified lipid mixtures were determined using the capillary method.27
Surfactant screening
The ability of the surfactant to emulsify Solid-Liquid Binary lipid (SLB) was a key factor in its selection for developing NLCs. A solution containing 5% surfactant was mixed with 100 mg of SLB diluted in 3 mL of methylene chloride, with magnetic stirring. Distilled water was then added to the solution after removing the organic phase at 40°C. Transmittance percentage of the samples was measured in UV-visible spectroscopy at 510 nm.28
Statistical experimental design for optimization
Thorough experimental design is crucial for improving nanoparticle-based formulations to avoid errors and repetition. Utilizing a design of experiments approach with statistical considerations helps maximize reliable and objective data.29 A 23 factorial experimental design was used to refine formulation ingredients, exploring the impact of three independent variables (surfactant concentration, lipid ratio, homogenization speed) at two levels (high and low) on dependent variables (globule size, zeta potential, polydispersity index and encapsulation efficiency) are presented in Table 1. This strategy was used for the assessment of primary components and their interaction effects.
Factors | Low level | High level |
---|---|---|
Lipid ratio (%) | 2 | 3 |
Surfactant concentration (%) | 2 | 4 |
Homogenization speed (rpm) | 10000 | 15000 |
The experimental design includes eight runs, are detailed in Table 2. Optimization was carried out by evaluating solutions which meets predefined criteria, such as maintaining factors within specified ranges, minimizing PDI, particle size and maximizing zeta potential, EE. Design Expert software Version 13.0 was employed for optimization, guided by the desirability factor aiming for values close to 1.
Formulation | Factor1 | Factor2 | Factor3 | Response1 | Response2 | Response3 | Response4 |
---|---|---|---|---|---|---|---|
Lipid ratio (%) | Concentration of Surfactant (%) | Homogenization speed (rpm) | Particle size (nm) | PDI | Zeta potential (mV) | Entrapment efficiency (%) | |
1 | 3 | 4 | 15000 | 255.4 | 0.458 | -23.8 | 97.32 |
2 | 2 | 2 | 10000 | 363.5 | 0.745 | -18.4 | 91.62 |
3 | 2 | 4 | 10000 | 210.1 | 0.376 | -22.6 | 95.78 |
4 | 2 | 2 | 15000 | 248.3 | 0.896 | -14.4 | 96.86 |
5 | 3 | 4 | 10000 | 287.3 | 0.385 | -21.9 | 97.16 |
6 | 3 | 2 | 10000 | 208.4 | 0.437 | -11.3 | 92.35 |
7 | 3 | 2 | 15000 | 320.1 | 0.512 | -16.3 | 96.43 |
8 | 2 | 4 | 15000 | 194.5 | 0.368 | -23.2 | 97.87 |
Development of Nanostructured lipid carrier of Gemcitabine
The NLC dispersion of Gemcitabine was prepared using high-shear homogenization, employing a modified version of the approach as outlined by Pallavi Chand et al. (2021). It had an 80:20 solid-to-liquid lipid ratio, with the solid lipid (GMS) and liquid lipid (oleic acid) melted at a temperature exceeding their respective melting points of 75°C. Gemcitabine was dissolved in the aqueous phase. The aqueous phase with Tetronic 1107 surfactants was slowly added to the lipid phase, followed by stirring with a magnetic stirrer for 60 min. To achieve stable NLCs, the pre-emulsion underwent high-shear homogenization (using an Omni GLH 850 homogenizer) for fifteen mins, with three cycles of 5 min each, at various rpm.30
Functionalization of NLC with Biotin
Biotin coated NLCs were formulated using the electrostatic attraction method. The optimized formulation was added gradually to a 0.5% w/v biotin solution with vigorously stirring at 5,000 rpm. The formulation was stirred under a magnetic stirrer for overnight in order to coat its surface with biotin.31 and the formulation was lyophilized for further analysis.
Characterization
Fourier transform infrared spectroscopy analysis
Drug excipient compatibility was evaluated using FT-IR Spectrophotometer (8400 S SHIMADZU) with the KBr pellet technique. With the use of dried KBr as a baseline correction, the spectra of dry drug combinations containing with and without excipients were recorded. Samples have been compressed at a rate of 6 ton/nm2 to produce KBr pellets. The samples were scanned at wavelength range of 500-4000 cm-1 to record the IR spectra.32
Differential Scanning Calorimetry (DSC)
DSC analysis of the lyophilized formulation was performed using a Mettler DSC 3. Seven mg of formulation were placed in an aluminum pan and scanned under a nitrogen atmosphere from 30°C to 300°C at a constant rate of 10°C/min. An empty, sealed aluminum pan served as a reference.
Particle size and Polydispersity index (PDI) analysis
Particle size, PDI of prepared Gemcitabine NLC and Biotin-GEM- NLC were assessed using a Zeta sizer Nano ZS-90 instrument (Malvern Instruments, Malvern, UK), which detects fluctuations in light scattering due to the Brownian motion of particles. For each sample, 1 mL of nano-carrier dispersion was diluted with 9 mL of water into a quartz cuvette positioned at 90° from the incident beam, maintained at 25°C.33 Each measurement was performed thrice to ensure accuracy.
Zeta potential analysis
The assessment of the electrostatic potential surrounding nanoparticles in solution is referred to as the zeta potential, which characterizes the electrical double layer around the particles. 1 mL of nano-carrier dispersion was diluted with 9 mL of distilled water into a quartz cuvette maintained at 25°C, with the electrode immersed in the solution. The zeta potential of the samples was measured using Nano ZS 90 Zetasizer (Malvern, United Kingdom).
Entrapment Efficiency (EE) analysis
The EE was assessed using centrifugal ultrafiltration tubes through ultrafiltration method. 2 mL of the Gemcitabine NLC dispersion was added to the centrifugal tube and centrifuged at 10,000 rpm for 30 min at room temperature using an Eppendorf centrifuge 5810. Thereafter, amount of unentrapped Gemcitabine in the filtrate was determined at 267 nm by UV-visible spectroscopy.34 The following equation was used to determine the EE:
Morphological Examination
SEM analysis
A scanning electron microscopy (ZEISS EVO 18 model, Germany) was used to examine the surface morphology. The sample was attached to a metallic stub by placing it on a glass cover slip that had previously been wrapped in bio-adhesive carbon tape. Before being examined, the sample was coated to produce a conducting surface and let air dry. Finally, a vacuum Scanning Electron Microscope (SEM) was employed to investigate the substance.
Phase contrast microscopy analysis
Phase contrast microscopy relies on the ability to convert minute alterations in the phase of light rays, caused by variations in the thickness and refractive index across an object, into variations in brightness or light intensity. In this study, phase contrast images of GEM NLC opt, biotin-GEM-NLC were captured using a Sony digital camera and subjected to analysis.
In vitro drug release
In vitro release of Gemcitabine-NLC, Biotin-GEM-NLC were assessed by using Open ended cylinder method using 7.4 pH phosphate buffer as the dissolution medium. A dialysis membrane was connected to one end of a glass cylinder. 2 mL of biotin-coated, gemcitabine-loaded NLC were accurately loaded into the cylinder and placed in 200 mL of dissolution medium at 37±5°C. It was then secured to a stand and the membrane was slightly raised to the surface of the receptor media. The dissolution medium was slowly stirred using a magnetic stirrer. Samples were periodically withdrawn from the receiver compartment and a 5 mL portion was replaced with fresh medium at specified intervals to maintain sink condition. The absorbances of the withdrawn samples was measured using a UV-Visible spectrophotometer at a wavelength of 267 nm.35
Drug release kinetics
Different kinetic release models such as first order, zero order, Korsmeyer-Peppas, Weibull, Hixson-Crowell’s, Higuchi were utilized to analyze the release of GEM-NLC-opt and Biotin-GEM- NLC. The selection of most suitable release kinetic model was based on the R2 parameter, employing a DD solver software.
In vitro cytotoxicity study
This study assessed the cytotoxicity and effect on cell proliferation of the test compound in A549 cells using a colorimetric assay. Formazan crystals catalyzed the reduction of the water-soluble tetrazolium dye MTT. Biotin-coated GEM-NLC was incubated with concentrations ranging from 0.78 to 12.5 μg/mL for 24 hr at 37°C in a 5% CO2 atmosphere. Following incubation, to achieve a final concentration of 0.5 mg/mL, MTT reagent was added and the experiment continued for 3 hr. Then, 100 μL of DMSO solubilization solution was added and gentle stirring facilitated dissolution. Cell viability was quantified spectrophotometrically at 570 nm.36 Cell viability percentage was calculated using the formula:
Stability assessment
Stability analysis was conducted to assess the stability of the optimized formulation in compliance with the guidelines outlined in International Conference on Harmonization (ICH, Q1A). The samples were stored over two months at a refrigerated temperature of 4±0.5°C and characterized for Zeta Potential (ZP) and Particle Size (PS), Polydispersity Index (PDI) at specified intervals of 0, 30 and 60 days.37
Statistical analysis
All experiment results were reported as the mean±Standard deviation. The statistical analysis was carried out utilizing version 10 of the graph pad prism software, p<0.05 was referred as minimum significance.38
RESULTS
Screening of lipids
The choice of lipids for the production of Gemcitabine (GEM)- loaded Nanostructured Lipid Carriers (NLCs) was determined by taking into account the solubility profile of GEM. Figure 1A illustrates the solubility of GEM in several solid lipids, with glyceryl monostearate exhibiting the highest solubility, followed by stearic acid, cetostearyl alcohol, cetyl palmitate, palmitic acid and Precirol ATO, in descending order. Conversely, Figure 1B illustrates the GEM solubility in different liquid lipids, with oleic acid demonstrating the highest solubility, succeeded by Labrafenac PG, Capmul MCM, cinnamon oil, ethyl oleate, Maiscine CC and soybean oil. Among the solid lipids evaluated, glyceryl monostearate exhibited the highest solubility of GEM, measured at 19.8 mg/100 mg. Following closely, oleic acid demonstrated a solubility of 8.4 mg/mL, indicating its significant potential as a liquid lipid for GEM in NLC formulations. These findings underscore the importance of selecting appropriate liquid lipids with high solubilizing capacity for GEM to ensure optimal drug loading and stability in NLCs.
Binary Lipid mixture selection
The ratio of solid to liquid lipid (80:20) was selected to align with the melting point of the solid lipid, which ranges from approximately 57°C to 65°C.
Surfactant screening
Surfactant selection for the production of NLCs was based on the emulsification capacity of the lipids. Emulsification efficiency of various surfactants was assessed by measuring the percentage transmittance of Gemcitabine (GEM) in lipid-surfactant solutions. The percentage transmittance of GEM in different surfactants was represented in Figure 1C revealing Tetronic 1107 to exhibit the highest transmittance, followed by Tween 80, Tetronic 1307 and Pluronic F 68. Notably, Tetronic 1107 a non-ionic surfactant demonstrated the highest percentage transmittance of GEM, reaching 96.572%, emerged as the optimal choice for NLC development due to its superior emulsification capacity and biocompatibility. This surfactant is characterized by its hydrophilic nature, possessing a high Hydrophilic-Lipophilic Balance (HLB) value of 18, which enhances its ability to stabilize lipid-based emulsions effectively.39 Moreover, Tetronic 1107 is known for its safety profile, posing no physiological harm in humans. These attributes strongly justify the selection of Tetronic 1107 an ideal candidate for facilitating the emulsification of lipids and the encapsulation of GEM in NLC formulations.
Optimization of NLC containing Gemcitabine
A 23 a factorial design was executed using Version 13.0 of the Design Expert® software by State Ease was used to acquire the optimized formulation. This design comprised eight formulations, where three factors- ratio of lipid (A), concentration of surfactant (B) and homogenization speed (C) were varied, while globule size, zeta potential, PDI, entrapment efficiency were monitored as responses are outlined in Table 2. The association between independent and dependent factors was assessed through actual-predicted plot evaluation, model statistics and ANOVA analysis to determine significance and severity. Utilizing a regression model, a 3D surface was generated to evaluate the signal-to-noise ratio, aiming for a value exceeding 4. The proposed model exhibited remarkable accuracy and responsiveness within the defined parameters. The anticipated R2 value, indicative of response variable predictability, was computed and compared to observed and predicted R2 values, showing satisfactory consistency with a difference of less than 0.2. These results underscore the statistical validity and strong alignment with the provided dataset, reflecting a robust fit. Summary statistics and ANOVA analysis outcomes are represented in Tables 3 and 4.
Response | R2 | Adjusted R2 | Predicted R2 | Adequate precision |
---|---|---|---|---|
Particle size | 0.9630 | 0.9136 | 0.7368 | 12.1603 |
PDI | 0.9219 | 0.8633 | 0.6875 | 8.6253 |
Zeta potential | 0.8682 | 0.8155 | 0.6627 | 9.0854 |
Entrapment efficiency | 0.9842 | 0.9724 | 0.9370 | 27.132 |
Response | Sum of squares | Mean squares | F value | p value | Status |
---|---|---|---|---|---|
Particle size | 24681.45 | 6170.36 | 19.51 | 0.0174 | Significant |
PDI | 0.2020 | 0.0673 | 15.73 | 0.011 | Significant |
Zeta potential | 107.50 | 53.75 | 16.47 | 0.0063 | Significant |
Entrapment efficiency | 3.15 | 1.05 | 83.32 | 0.0005 | Significant |
Impact of Independent variables over size of the particle
The Response Y1 model, regarding particle size, demonstrated a substantial F-value of 19.51, confirming the model significance according to the ANOVA table.
A-Total lipid ratio (%), B-Concentration of Surfactant, C-Speed of Homogenization.
The symbols “+” and “-” indicate respectively increasing and decreasing effects on the response variable concerning the input variable. An adequate precision of 12.1603 signified a satisfactory signal. Particle diameters in all eight Gemcitabine loaded NLC dispersions ranged from 194.5 nm to 363.5 nm. Higher lipid content led to larger NLC particle sizes due to increased viscosity, diminished surfactant emulsification capacity and heightened interfacial tension causing particle agglomeration. Conversely, a reduction in particle size observed with increase in surfactant ratio, attributed to surfactant adsorption at the interface, which reduced interfacial tension and facilitated aqueous-lipid phase separation.40,41
Impact of Independent variables over Polydispersity index
The Response Y2 model, assessing PDI, demonstrated a significant F-value of 15.73. The regression equation for PDI was expressed as
A-Total lipid ratio (%), B-Surfactant Concentration, C-Homogenization speed.
An adequate precision of 8.625 denoted a satisfactory signal. Higher lipid concentrations were associated with lower Polydispersity Index (PDI), indicating a more uniform particle size distribution attributed to denser core packing. Similarly, increasing surfactant concentration reduced PDI.42
Impact of Independent variables over Zeta potential
The Response Y3 model, assessing zeta potential, demonstrated a significant F-value of 16.47. The regression equation for zeta potential was expressed as,
A- Total lipid ratio (%), B- Surfactant Concentration, C- Homogenization speed.
Adequate precision of 9.0854 confirmed a sufficient signal. Lipid ratio and surfactant concentration exerted a negative effect on zeta potential, while the combination of total lipid and homogenization speed yielded a positive effect. This negative influence was attributed to the presence of negatively charged lipids, while the minimal effect was attributed to the use of a non-ionic surfactant and the positively charged drug.43
Impact of Independent variables over Entrapment Efficiency
The Response Y4 model, concerning entrapment efficiency, exhibited a highly significant F-value of 83.32. The regression equation for entrapment efficiency was formulated as:
A-Total lipid ratio (%), B-Surfactant Concentration, C-Homogenization speed.
Adequate precision of 27.132 indicated a sufficient signal. Increased lipid content led to higher entrapment efficiency by providing more space for drug lodging and reducing drug escape to the exterior phase. Similarly, increased surfactant content enhanced entrapment efficiency by increasing the aqueous phase viscosity, reducing drug dispersion into the external phase. Additionally, higher homogenization speed resulted in increased entrapment efficiency as particle size decreased.44
Optimization and Validation of Results
Utilizing Design Expert software, a 23 full factorial design point prediction table (Table 5) identified the optimum formulation for gemcitabine-loaded NLC. The factors yielding the best results, with a desirability value of 0.795, were determined to be lipid ratio, surfactant concentration and homogenization speed. The selected optimized formulation, characterized by a particle size measuring 194.5 nm, zeta potential of -23.2 mV, PDI of 0.368, entrapment efficiency of 97.87%, underwent further investigation. The software predicted that the optimal formulation would possess a particle diameter of 230.83 nm, ZP of -21.091 mV, PDI of 0.453 and EE of 97.23% as depicted in Table 5. Statistical significance was demonstrated by a 95% confidence interval for the response variables (Y1, Y2, Y3, Y4) and a p-value less than 0.05. Response surface plots, including 3D plots and contour plots, were employed to analyze the combined influence of independent factors on the responses (Figure 2).
Variables | Optimized values | Responses | Predicted value | Observed values |
---|---|---|---|---|
Lipid ratio (%) | 2 | Particle size (nm) | 230.8 | 194.5 |
Surfactant concentration (%) | 4 | PDI | 0.453 | 0.368 |
Homogenization speed (rpm) | 15000 | Zeta potential (mV) | -21.09 | -23.2 |
Entrapment efficiency (%) | 97.235 | 97.87 |
These findings elucidate the intricate relationship between independent variables and their impact on the critical parameters governing the formulation of gemcitabine-loaded NLCs, thereby providing valuable insights for optimizing NLC formulations for enhanced drug delivery efficacy.
Functionalization of Biotin with NLC
Biotin coated NLC were prepared by the principle of electrostatic attraction. The formulation was coated with 0.5% biotin solution. Sufficient time was provided to make sure the coating of biotin onto the surface of NLC. The coating solution of biotin was to be coated onto the surface of NLC formulation by electrostatic attraction. The Biotin solution carries positive charge which gets attracted towards NLC which carries negative charge in it. The surface coating of biotin was confirmed by SEM and Phase contrast microscopy.
Characterization
Particle size, Polydispersity index
The average particle diameter and Polydispersity Index (PDI) for GEM-NLC and Biotin-GEM-NLC are shown in Figure 3. The mean particle sizes for Biotin-GEM-NLC and optimized GEM-NLC are 203.1 nm and 194.5 nm, respectively. The PDI values are 0.368 for the optimized NLC and 0.486 for the Biotin-GEM-NLC.
Zeta potential
Zeta potential measurements were conducted to assess the stability and surface potential of the colloidal dispersion. The zeta potential values for optimized GEM-NLC and Biotin-GEM-NLC were found to be -23.2 mV and 20.4 mV respectively. The negative sign indicates the electrostatic repulsion between particles. Figure 4 represents the zeta potential graphs for both the formulation.
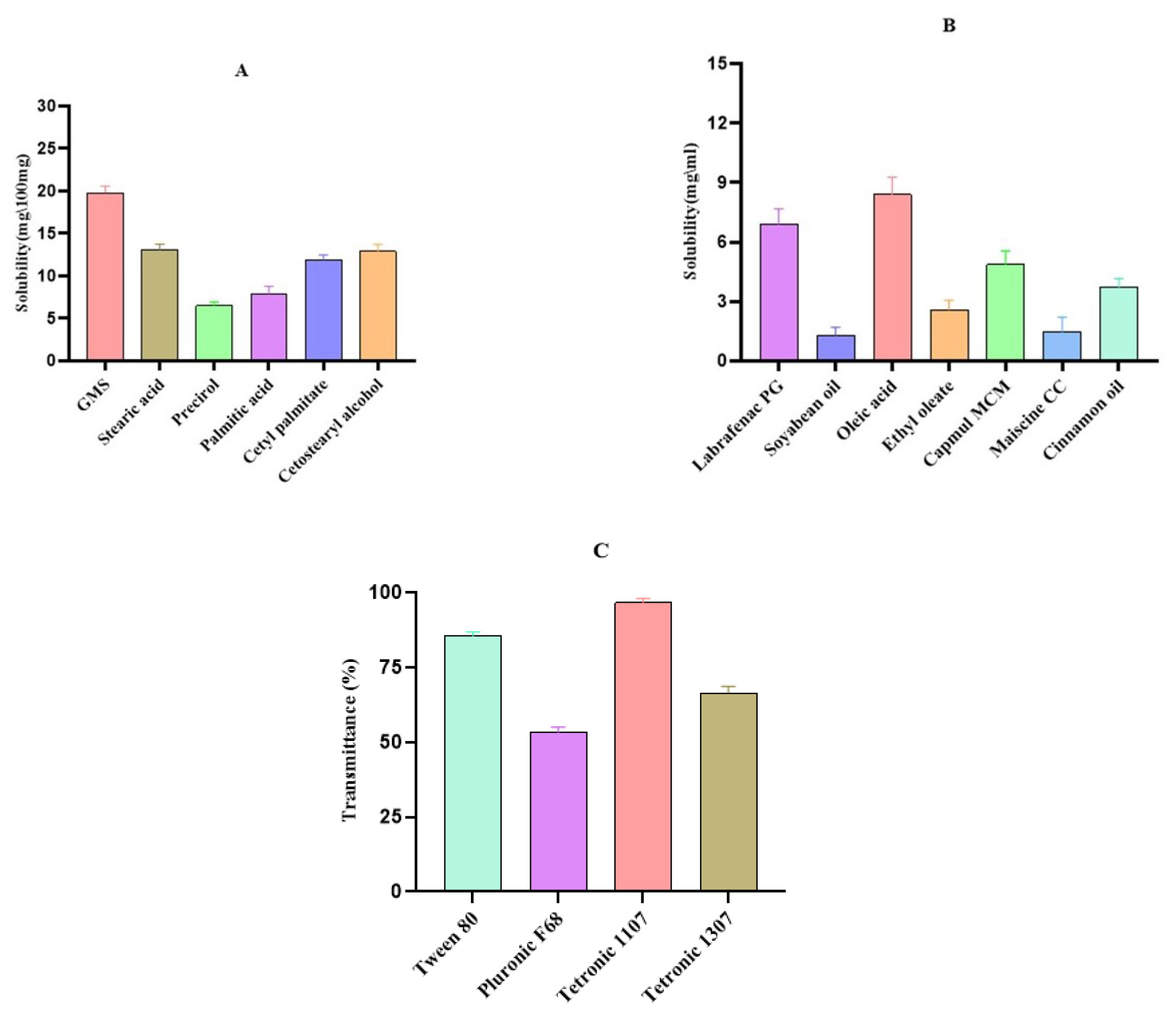
Figure 1:
GEM’s solubility profile in A) Solid lipid B) Liquid lipid C) Transmittance percentage of Surfactant. The results were presented as mean±SD (standard deviation), based on three replicates (n=3).
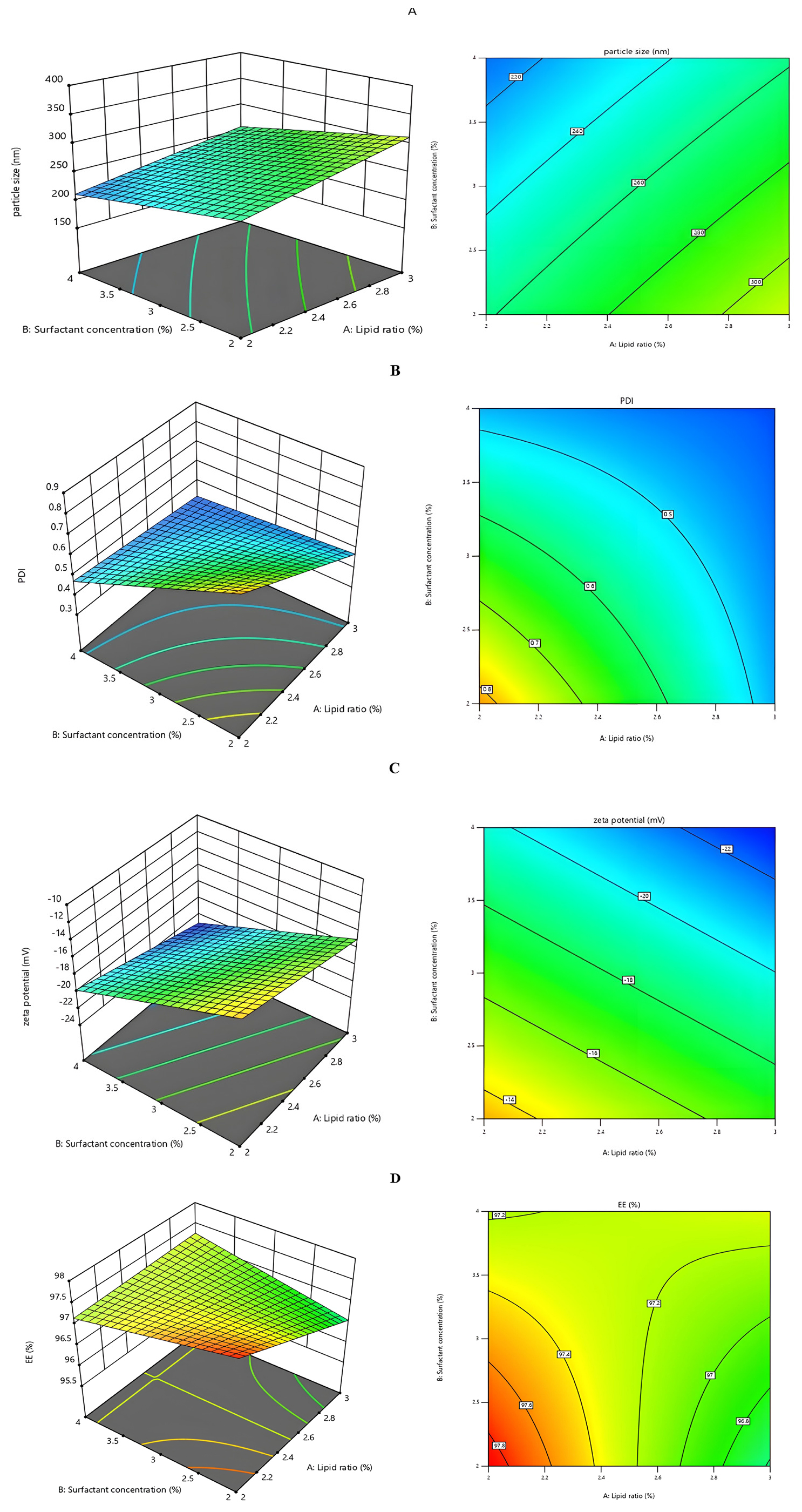
Figure 2:
Response surface (3D) and contour plot of A) Particle size B) PDI C) ZP D) Entrapment efficiency.
Entrapment efficiency
The EE can be calculated by measuring the amount of unentrapped drug in supernatant can be determined by indirect method. The % EE of formulations lies in the range of 91.62-97.87%. The entrapment value for final optimized formulation was found to be 97.87% and found to be significant using one sample t test (<0.0001).
Differential scanning colorimetry
The DSC thermogram of pure Gemcitabine displayed a single endothermic peak at 257.55°C (Figure 5A). The thermogram of final lyophilized formulation (Figure 5B) exhibited a peak at 51.26°C, attributed to Glyceryl monostearate,45 without a discernible endothermic peak for Gemcitabine. These findings suggest no interaction between the constituents of the NLCs and confirm the entrapment of constituents within the NLC structure.
FTIR studies
The physical mixture shows the amine functional group inherent in gemcitabine, revealed as an absorption peak at 3514.42 cm-1 in the FT-IR spectrum. This distinctive peak corresponds to the stretching vibration of the N-H bond within the gemcitabine molecule. The absorption peak at 1113.92 cm-1 represents the presence of ether functional group with the stretching of C-O-C that present in Tetronic 1107 molecule. The absorption peaks at 1350.22 cm-1 (C-O bond stretching), 1614.47 cm-1 (C=C bond stretching) of glyceryl monostearate and oleic acid as displayed in Figure 6A. Hence the drug and mixture were known to be compatible with each other and it can be further formulated.
Figure B illustrates the spectrum of Biotin-GEM-NLC. Biotin coated is confirmed by the presence of a COOH group, demonstrated by characteristic absorption peaks at 1684.88 cm-1.
Morphological examination
Scanning Electron Microscopy
Figure 7 represents the SEM picture of Biotin-GEM-NLC. The image depicts a smooth-surfaced, spherically shaped particle, which illustrates that there was no aggregation between the particles.
Phase Contrast Microscopy
The biotin-coated NLC was examined using phase contrast microscopy and given in Figure 8. The image which was produced using the blue filter in PCM, confirms the biotin surface coating on NLC surface. Under PCM, the surface-coated biotin appeared to be visible, with a coating appearing on the NLC surface.
In vitro Gemcitabine Release Study
The cumulative release of Gemcitabine (GEM) from both Biotin-coated NLC and Optimized NLC formulations over a specified duration, as illustrated in Figure 9. A biphasic drug release profile was observed for both NLC dispersions, characterized by an initial rapid release followed by a sustained release. During the first hour, approximately 26.2% of GEM was rapidly released from the NLC dispersions, indicating the release due to the drug molecules present near the surface of the NLCs. Subsequently, a longer release phase ensued, approximately 95.13% of GEM released over an 8 hr period. These suggestions indicate that efficient encapsulation of the majority of GEM is within the solid core of NLCs, are tightly integrated with the lipid matrix.46 Biotin-coated NLCs displayed a comparable biphasic release profile as compared to Optimized NLCs, albeit with a lower cumulative release during the prolonged release of approximately 96.04% over 24 hr period. These findings suggest that potential interactions exist between the biotin coating and NLC polymers, resulting in a slower release rate.47 Indeed, previous studies have reported that polymer coated onto the surface of NLCs can impede drug release, leading to sustained release kinetics. Statistical analysis using one-way ANOVA revealed that the release rate of GEM from Optimized NLC and Biotin-GEM-NLC formulation was significantly lower (p<0.0001). This emphasizes the influence of surface modification on drug release kinetics. These results showcase the promise of Biotin-coated NLCs as an effective drug delivery system, providing controlled release of GEM with improved stability and effectiveness. Further exploration of the mechanisms dictating drug release kinetics is essential for enhancing therapeutic results.
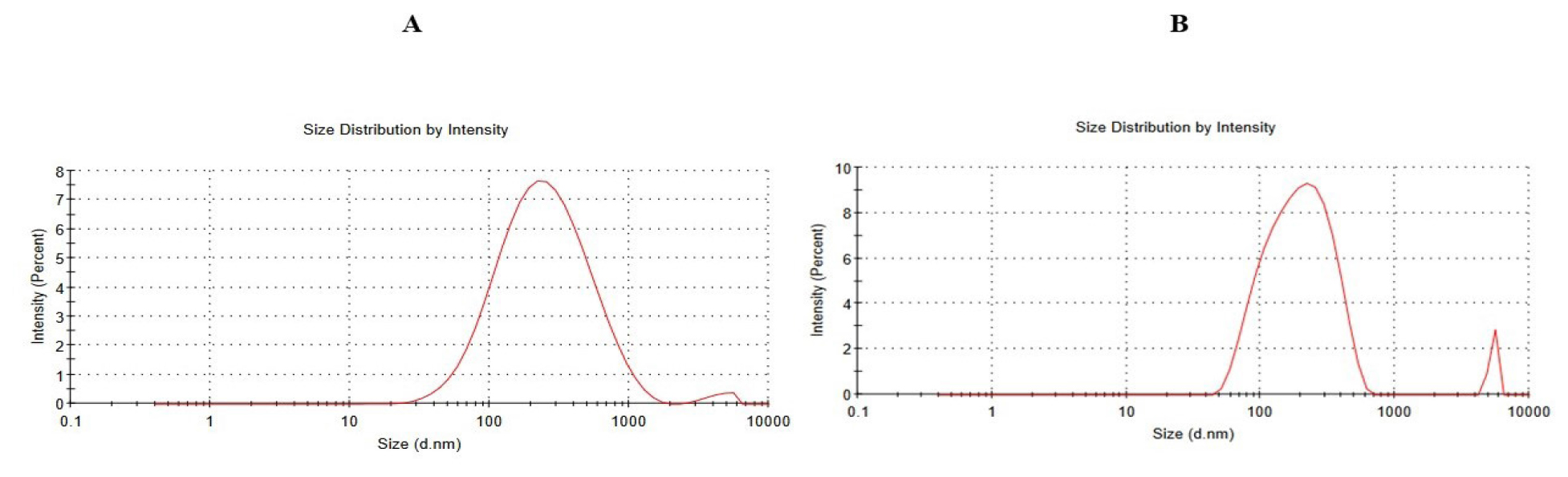
Figure 3:
Particle size of A) Optimized GEM-NLC B) Biotin-GEM-NLC.
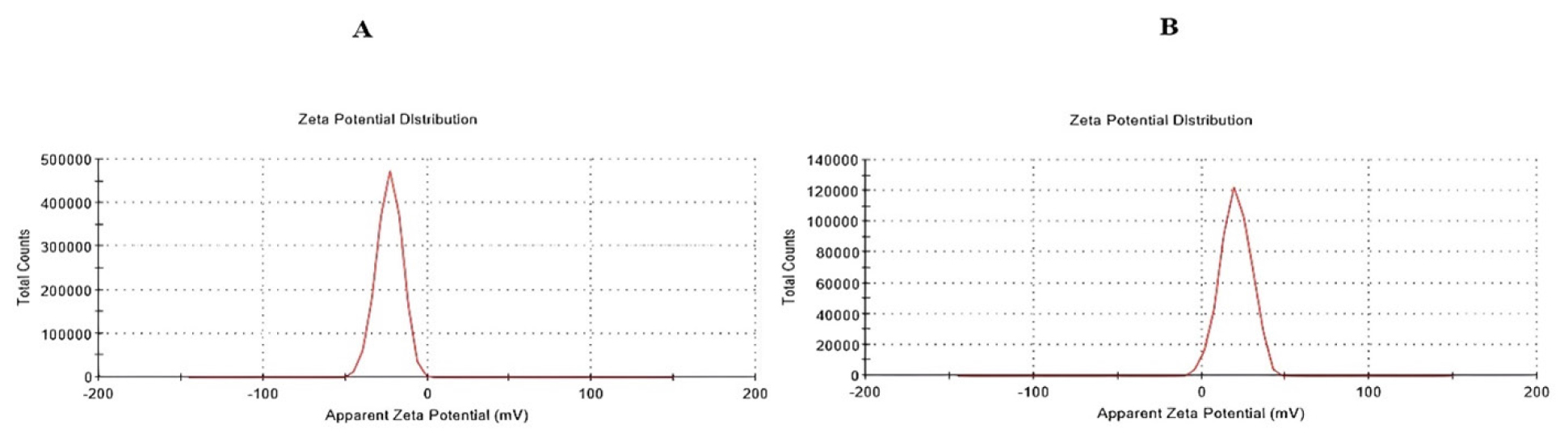
Figure 4:
Zeta potential of A) Optimized GEM NLC B) Biotin-GEM-NLC.
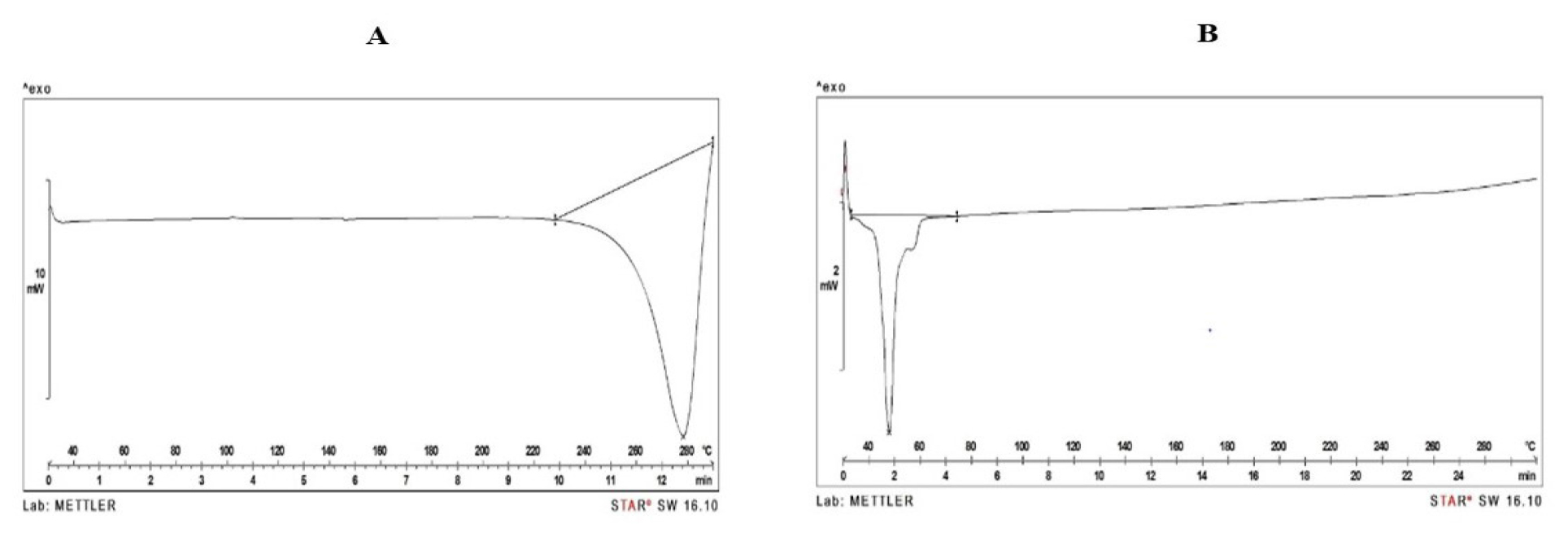
Figure 5:
DSC thermograms of A) Pure Gemcitabine B) Final lyophilized formulation.
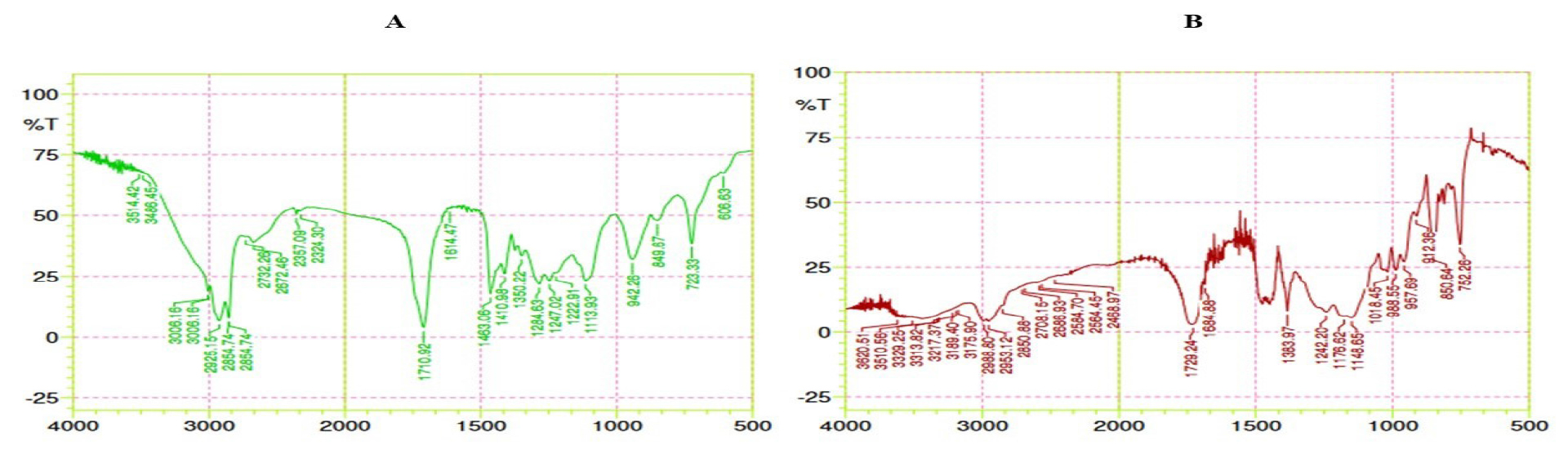
Figure 6:
FT IR spectrum of A) Physical mixture B) Final formulation.
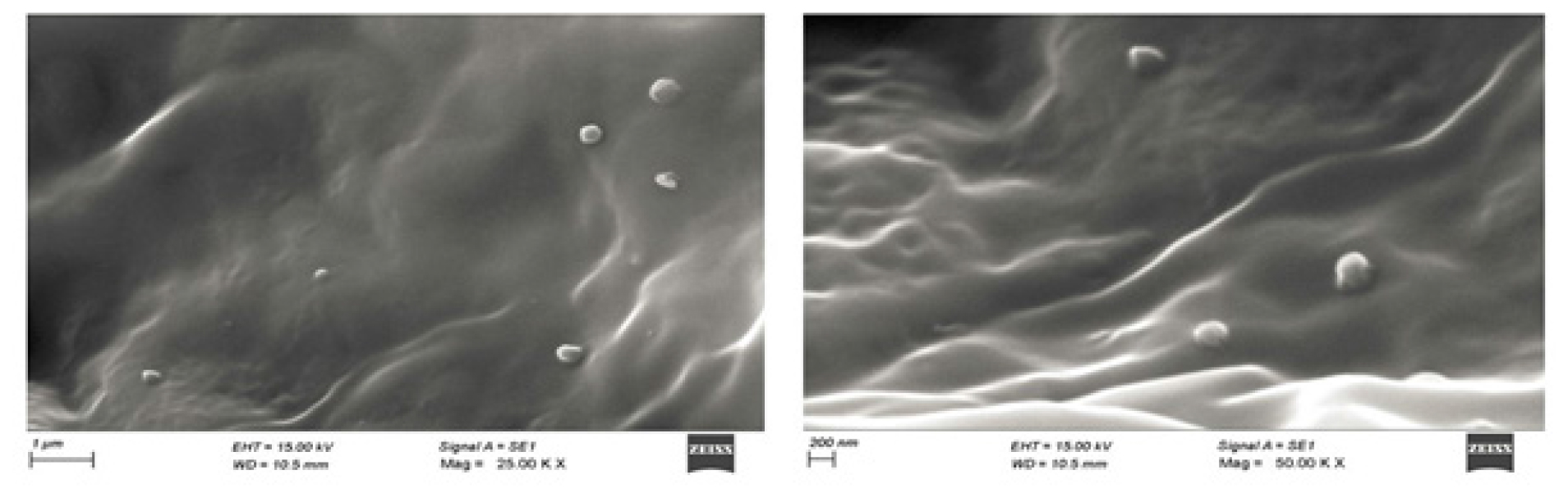
Figure 7:
SEM images of Biotin coated GEM-NLC.
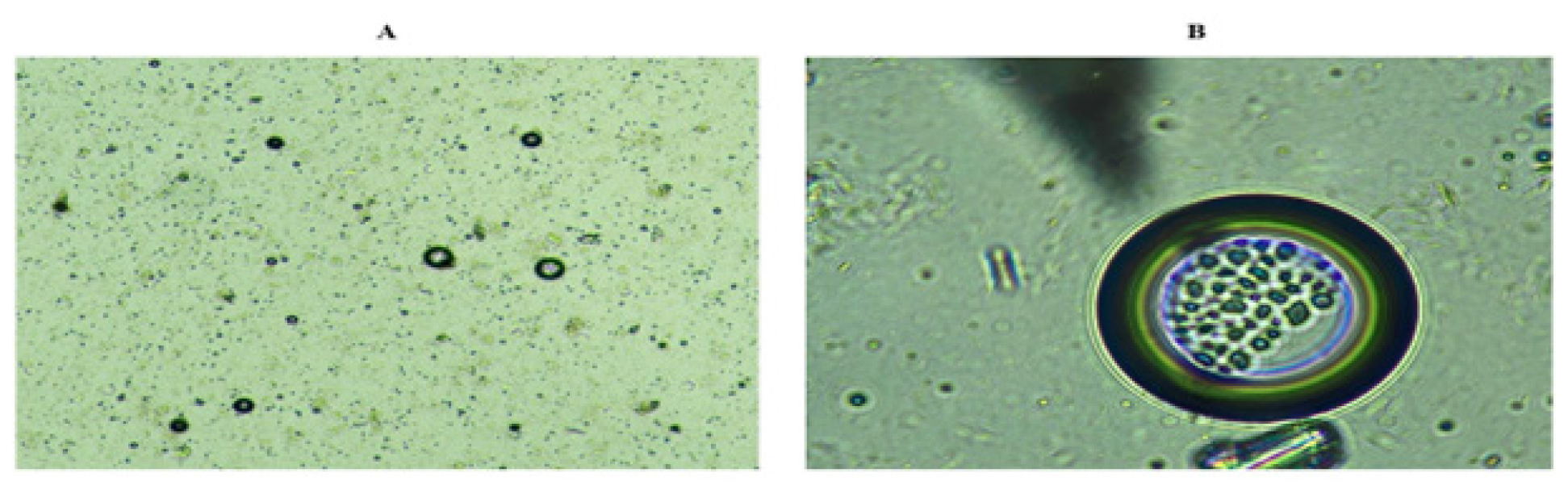
Figure 8:
Phase contrast microscopy of A) Optimized GEM NLC B) Biotin-GEM-NLC.
Release kinetics
Drug release pattern from the formulation was ascertained by the utilization of DD Solver, an add-on application for Microsoft Excel and the kinetic data was represented in Table 6. The GEM-NLC opt and Biotin-GEM-NLC exhibit first-order release. The Weibull model offers the accurate fit for both the formulations with R2 value of 0.9879 and 0.9990. When the β value exceed 0.75, it indicates, the combined release mechanism such as swelling of lipids, matrix erosion, drug diffusion occurring concurrently.48
Release kinetics model | Optimized NLC formulation | Biotin coated GEM-NLC |
---|---|---|
Zero order | 0.9531 | 0.8806 |
First order | 0.9927 | 0.9959 |
Hixson Crowell’s kinetics | 0.9679 | 0.9949 |
Higuchi model | 0.9576 | 0.9728 |
Korsmeyer Peppas model | 0.9650 | 0.9851 |
n value | n=0.555 | n=0.483 |
Weibull model | 0.9879 | 0.9990 |
In vitro cytotoxicity study
The cytotoxic efficacy of Biotin-coated Nanostructured Lipid Carriers (NLCs) was assessed using the MTT assay on A549 cell lines, comparing control and formulation-treated samples. IC50 value of Biotin-GEM-NLC against A549 cells was determined to be 0.88 μg/mL. Following a 24 hr incubation period, Biotin-GEM- NLC exhibited notable cytotoxic effects on A549 cells, eliciting a dose-dependent cell viability reduction as displayed in Figures 10, 11. Concentration of Biotin-GEM-NLC (12.5 μg/mL) demonstrates significant cytotoxicity of 97.92%, with a recorded cell viability of 2.08% as depicted in Table 7. This dose-dependent cytotoxic response underscores the potent anti-proliferative activity of Biotin-GEM-NLC against A549 cancer cells. Notably, the most pronounced cytotoxicity was observed at lower concentrations, indicative of the targeting moiety’s ability to facilitate specific drug delivery to cancer cells. The inclusion of biotin as a targeting moiety enhances the localization and internalization of the medication within cancer cells, thereby reducing the risk of systemic toxicity while augmenting the cytotoxic response.
Sl. No. | Concentration (μg/ mL) | Biotin NLC | |||
---|---|---|---|---|---|
% Cytotoxicity | % Cell viability | Cytotoxic activity | IC50 value | ||
1 | 0.78 | 43.99 | 56.01 | Moderate | 0.88 μg/mL |
2 | 1.56 | 64.69 | 35.31 | Moderate | |
3 | 3.125 | 75.07 | 24.93 | Severe | |
4 | 6.25 | 85.08 | 14.92 | Severe | |
5 | 12.5 | 97.92 | 2.08 | Severe |
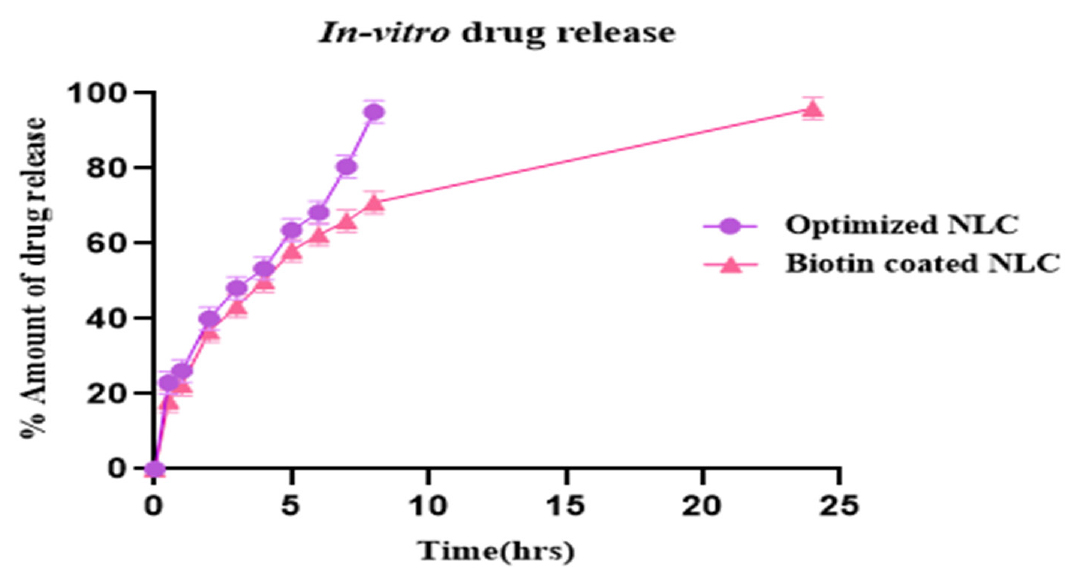
Figure 9:
In vitro drug release profile. The results were presented as mean±SD (standard deviation), based on three replicates (n=3).
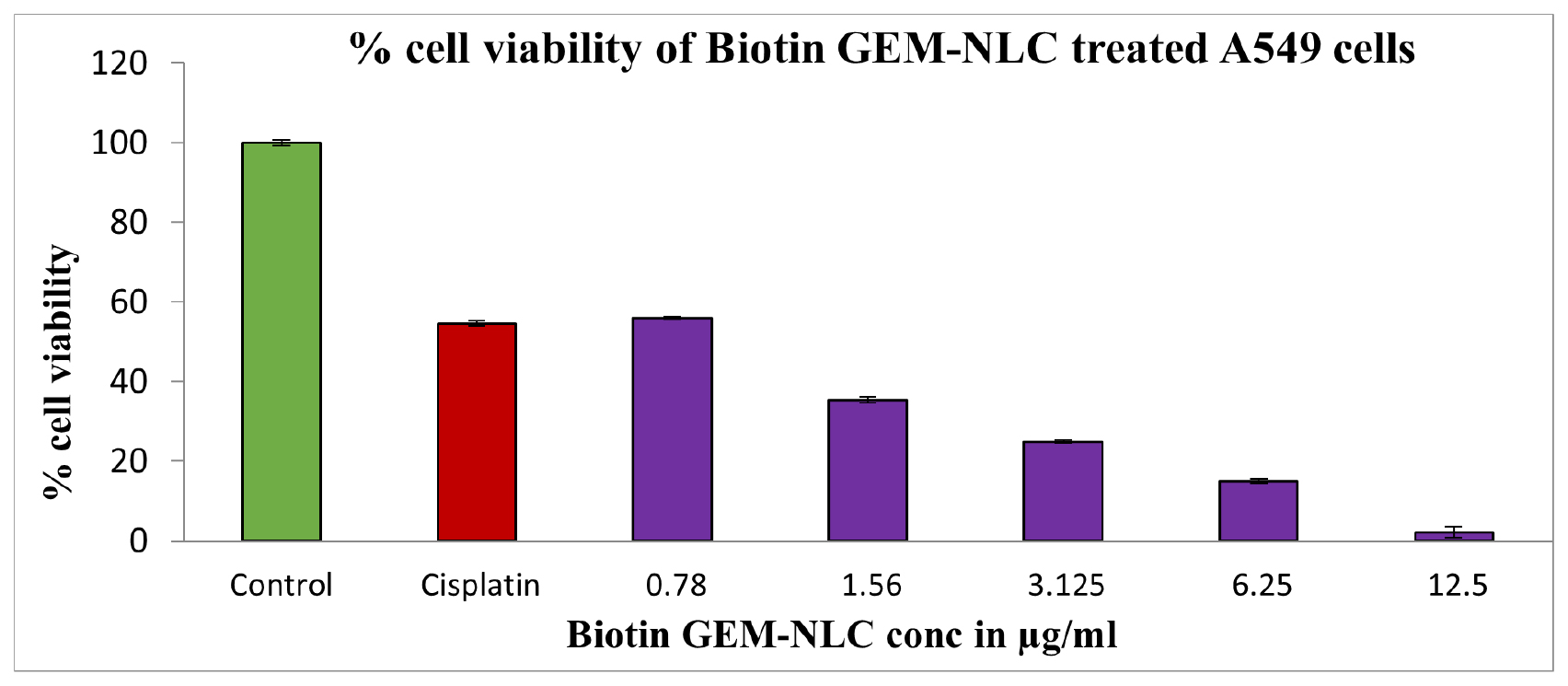
Figure 10:
Concentration vs percentage cell viability. The results were presented as mean±SD (standard deviation), based on three replicates (n=3).
Stability studies
Stability testing was done to determine the quality of formulation. The stability studies were conducted at refrigerated temperature for the period of 60 days. During the stability studies, there is no significant difference in the particle size, ZP, PDI which indicates the formulations were stable at the end of 2 months. The findings were given in Table 8.
Formulation | Duration | Particle size (nm) | PDI | Zeta potential (%) |
---|---|---|---|---|
Optimized formulation | Initial | 194.5±1.54 | 0.368±0.34 | -23.2±0.25 |
30 days | 213.7±2 | 0.474±0.67 | -21.9±0.78 | |
60 days | 219.4±1.2 | 0.505±0.42 | -21.6±0.46 | |
Biotin coated NLC | Initial | 203.1±0.97 | 0.486±0.2 | 20.4±0.15 |
30 days | 208±1.35 | 0.409±0.39 | 18.3±0.96 | |
60 days | 121.6±1.7 | 0.376±0.14 | 17.9±0.62 |
DISCUSSION
Nanostructured Lipid Carriers (NLCs) effectively deliver Gemcitabine into tumor cells. GMS and Oleic acid were selected as solid, liquid lipids based on Gemcitabine’s solubility profile. The emulsification efficiency of various surfactants was assessed by measuring the percentage transmittance of Gemcitabine (GEM) in lipid-surfactant solutions. Tetronic 1107, a non-ionic surfactant, displayed the highest emulsification efficiency (96.572%), making it the optimal choice for NLC development due to its superior emulsification capacity and biocompatibility.
Statistical tools are pivotal for optimizing formulation and process variables, ensuring quality while meeting specified criteria. Design of Experiments (DOE) is instrumental, which systematically adjust the variables to enhance formulation effectiveness and efficiency by minimizing the process variance. Employing a 23-factorial design, the impact of lipid ratio (A), homogenization rate (C) and surfactant concentration (B) on various responses were investigated. These responses, including particle size, Zeta potential, PDI, EE were analyzed using polynomial equations, revealing their significant influence. The interactions among these variables on the responses are illustrated in Figure 2 through response surface graphs and contour plots.
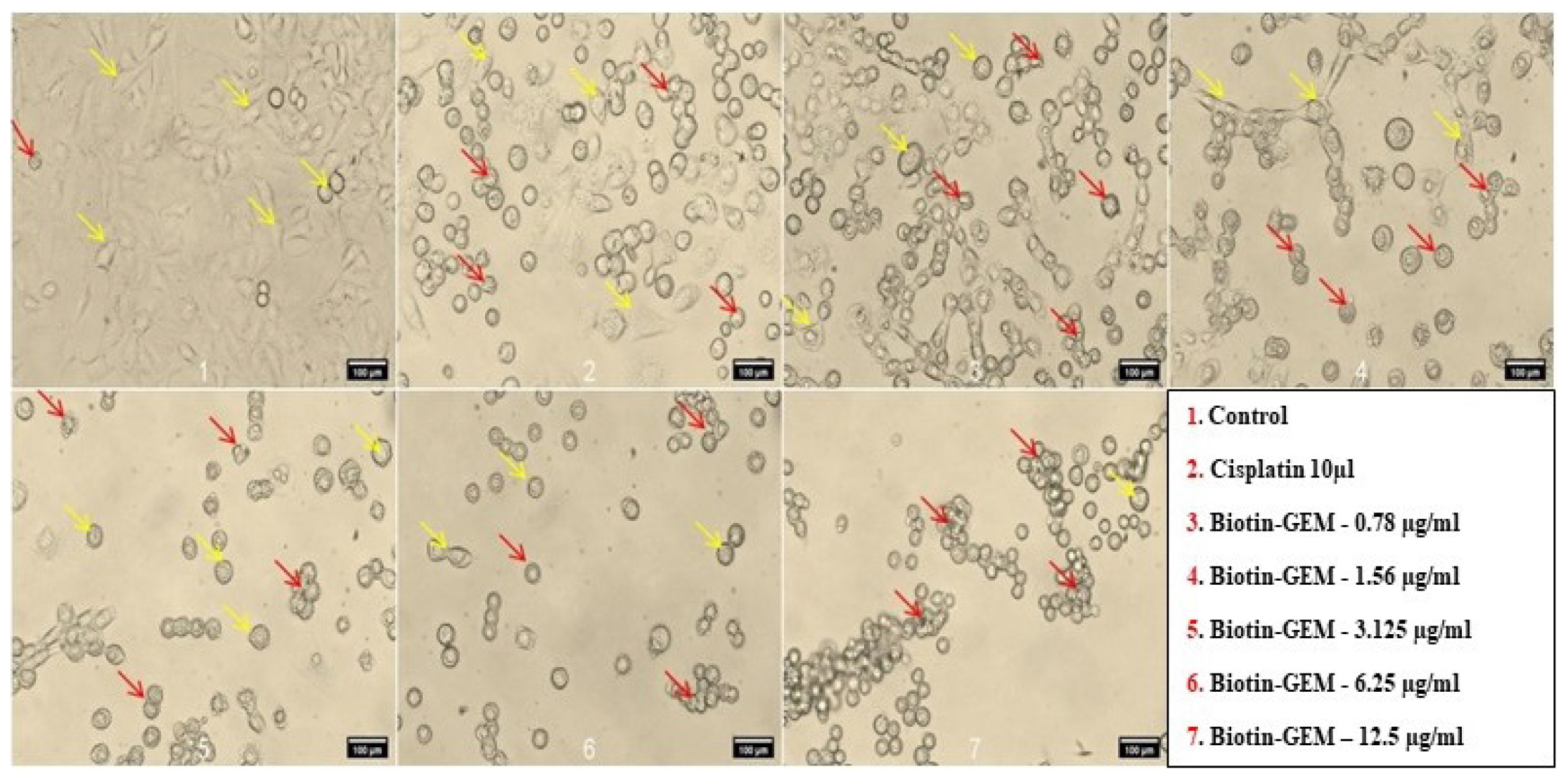
Figure 11:
Cytotoxic effect of formulation in A549 cell line at different concentration in which yellow arrow represents Healthy or viable cells and Red arrow represents dead or damaged cells.
Particle size is crucial in nanotherapeutic system development, influenced by formulation composition (lipids and surfactants) and process parameters like homogenization speed. Particularly, Nanostructured Lipid Carriers (NLCs) impact the uptake of chemotherapeutic agents by tumor cells and their targeting to tumors.49 Particle sizes within 50-200 nm range is vital for successful delivery of chemotherapeutic agents, with nanocarriers smaller than 200 nm demonstrating effectiveness in targeting tumor tissues via EPR mechanisms.50
Based on the findings, Gemcitabine-loaded Nanostructured Lipid Carriers (GEM-NLCs) exhibited a particle size of 194.5±2.76 nm. However, when these GEM-NLCs were coated with Biotin, there was a noticeable increase in particle size to 203.1±4.98 nm illustrated in Figure 3. The Polydispersity Index (PDI) indicates particle aggregation within nano systems. A PDI close to zero signifies uniform particle size distribution (monodisperse), while values above 0.5 indicate polydispersity with varying particle sizes. Monodisperse systems show less particle aggregation compared to polydisperse system.51 The Polydispersity Index (PDI) of GEM-NLC and Biotin coated NLC were determined to be 0.368 and 0.486, respectively, indicating monodisperse properties.
Zeta potential is crucial for both short and long-term nanoparticle stability, primarily due to the repulsive forces between charged molecules. Particles with surfaces close to neutral zeta potential tend to aggregate more rapidly. Sterically stabilizing surfactants, like non-ionic surfactants, contribute to particle stability through steric hindrance. Their use enables good colloidal stability even with lower zeta potential values.52 The zeta potential for GEM NLC and biotin NLCs were found to be -23.2 mV and 20.4 mV. This positive charge of Biotin-GEM-NLC was attributed onto the NLCs surface. Due to the negatively charged surface of cancer cell membranes, the positive charge on Biotin NLCs facilitates their interaction with cancer cells.53 The thermogram of final lyophilized formulation suggest no interaction between the constituents of the NLC. The FTIR studies of physical mixtures retained all the important functional groups of the drug and excipient, affirming the absence of any interaction between them. The Biotin-GEM-NLC spectra indicated the presence of Carboxyl group (COOH) on the Nanostructured Carrier (NLC). Gemcitabine encapsulation efficiency varied due to changes in lipid and surfactant concentrations. However, optimized Gemcitabine NLCs demonstrated a high encapsulation efficiency of 97.87±5.29%. Figure 7 showed smooth surface and spherical Biotinylated Gemcitabine NLC by the SEM results.
The drug release pattern, as already mentioned, exhibits a dual-release pattern. The early rapid drug release might be the dispersion of drug on the lipid core of NLC, succeeded by a gradual and steady release. When compared to GEM-NLC opt, Biotin-GEM-NLC shows a slower release at the end of 24 hr. This might be related to the existence of a Biotin layer on the outermost layer of the lipid carrier, which contributes to its prolonged release over 24 hr. The drug release kinetics were explored using several mathematical approaches, GEM-NLC and Biotin-GEM-NLC exhibited a Weibull release model., either through erosion of the lipid core or by diffusion.
Cytotoxicity study was performed to indicate GEM incorporated in NLC could enhance rate of cell death in cancer cells due to its equipped with Biotin. Biotin-GEM-NLC showed highest toxicity compared to control and standard drug. Biotin-GEM-NLC was assessed at different concentrations. The results showed that Biotin-GEM-NLC showed a dose-dependent cytotoxic efficacy on the A549 cells within 24 hr. Moreover, Biotin-GEM-NLC significantly increased inhibition on the proliferation of A549 cells in all concentrations showed in Figure 10. The IC50 value of Biotin-GEM-NLC (0.88±2.10) μg/mL in 24 hr treatment. These results indicated that Biotin-GEM-NLC had a considerable antiproliferation effect on carcinoma cells (A459)in-vitro. The stability testing was done to determine the quality of formulation. The stability studies concluded that after 60 days, there were no significant differences in particle size, PDI, ZP indicating that the formulations were stable.
CONCLUSION
Developing safe and efficient delivery systems for chemotherapy drugs to selectively target tumor cells while minimizing systemic adverse effects is a pivotal objective in cancer research. In pursuit of enhancing the efficacy of Gemcitabine (GEM) against A549 cancer cells, GEM-loaded Nanostructured Lipid Carriers (NLCs) targeted with biotin were synthesized. NLCs represent a novel class of lipid-based nanoparticles increasingly employed in cancer treatment strategies. Utilizing the high shear homogenization technique, GEM-loaded NLCs were successfully formulated. A comprehensive optimization process was conducted using a 23 full factorial design, considering various parameters to achieve the optimal formulation. Statistical analysis was employed to evaluate the results, encompassing measurements of particle size, zeta potential, PDI, entrapment efficiency, morphology, in vitro release kinetics. The findings demonstrated favorable entrapment efficiency and consistent particle size distribution in both Biotin-GEM-NLC and Optimized NLC formulations. Furthermore, drug release exhibited a biphasic profile, characterized by gradual and sustained release over an extended duration. Notably, Biotin-GEM-NLC displayed enhanced cellular uptake efficiency in A549 cells, attributed to endocytosis mediated by the biotin receptor. Cytotoxicity studies revealed a significant anti-cancer effect of Biotin-GEM- NLC on A549 lung cells compared to standard drug (Cisplatin). Overall, the study underscores the potential of nano lipid carriers to enhance the effectiveness of GEM against A549 cancer cells. This novel delivery system presents a promising alternative to conventional methods, offering improved targeting and efficacy while minimizing systemic toxicity. Further investigation through in vivo studies is warranted to comprehensively evaluate the clinical feasibility and therapeutic potential of this system, ultimately paving the way for clinical trials.
Cite this article:
Sureshkumar R, Muniswamy N. Biotin-Functionalized Lipid Nanoparticles: A Promising Approach for Gemcitabine Delivery in Non-Small Cell Lung Cancer Treatment. J Young Pharm. 2024;16(3):520-34.
ACKNOWLEDGEMENT
The PSG College of Pharmacy administration at Peelamedu, Coimbatore, is greatly appreciated by the authors for providing the facilities required for carrying out this research and we acknowledge.
ABBREVIATIONS
UV | Ultraviolet |
---|---|
GEM-NLC | Gemcitabine nanostructured lipid carrier |
Biotin-GEM-NLC | Biotin Gemcitabine Nano lipid carrier |
n | Release exponent |
R2 | Regression coefficient |
SEM | Scanning Electron Microscopy |
PCM | Phase Contrast Microscopy |
PS | Particle size |
FTIR | Fourier Transform Infrared Spectroscopy |
EE | Entrapment efficiency |
PDI | Polydispersity Index |
MTT | (3-[4,5-dimethylthiazol-2-yl]-2,5 diphenyl tetrazolium bromide). |
References
- Mottaghitalab F, Farokhi M, Fatahi Y, Atyabi F, Dinarvand R. New insights into designing hybrid nanoparticles for lung cancer: diagnosis and treatment. J Control Release. 2019;295:250-67. [PubMed] | [CrossRef] | [Google Scholar]
- Kumar K, Chawla R. Nanocarriers-mediated therapeutics as a promising approach for treatment and diagnosis of lung cancer. J Drug Deliv Sci Technol. 2021;65:102677 [CrossRef] | [Google Scholar]
- Wang XB, Zhou HY. Molecularly targeted gemcitabine-loaded nanoparticulate system towards the treatment of EGFR overexpressing lung cancer. Biomed Pharmacother. 2015;70:123-8. [PubMed] | [CrossRef] | [Google Scholar]
- Li Y, Liu Y, Chen Y, Wang K, Luan Y. Design, synthesis and antitumor activity study of a gemcitabine prodrug conjugated with a HDAC6 inhibitor. Bioorg Med Chem Lett. 2022;72:128881 [PubMed] | [CrossRef] | [Google Scholar]
- Jiang Z, Zhao Y, Zhao Y, Liu Y, Tao L. Pristimerin synergizes with gemcitabine through abrogating Chk1/53BP1-mediated DNA repair in pancreatic cancer cells. Food Chem Toxicol. 2021;147:111919 [PubMed] | [CrossRef] | [Google Scholar]
- Bor G, Mat Azmi ID, Yaghmur A. Nanomedicines for cancer therapy: current status, challenges and future prospects. Ther Deliv. 2019;10(2):113-32. [PubMed] | [CrossRef] | [Google Scholar]
- Miele E, Spinelli GP, Miele E, Di Fabrizio E, Ferretti E, Tomao S, et al. Nanoparticle-based delivery of small interfering RNA: challenges for cancer therapy. Int J Nanomedicine. 2012;7:3637-57. [PubMed] | [CrossRef] | [Google Scholar]
- Müller RH, Radtke M, Wissing SA. Nanostructured lipid matrices for improved microencapsulation of drugs. Int J Pharm. 2002;242(1-2):121-8. [PubMed] | [CrossRef] | [Google Scholar]
- Shao Z, Shao J, Tan B, Guan S, Liu Z, Zhao Z, et al. Targeted lung cancer therapy: preparation and optimization of transferrin-decorated nanostructured lipid carriers as novel nanomedicine for co-delivery of anticancer drugs and DNA. Int J Nanomedicine. 2015;10:1223-33. [PubMed] | [CrossRef] | [Google Scholar]
- Cao C, Wang Q, Liu Y. Lung cancer combination therapy: doxorubicin and β-elemene co-loaded, pH-sensitive nanostructured lipid carriers. Drug Des Dev Ther. 2019;13:1087-98. [PubMed] | [CrossRef] | [Google Scholar]
- Rawal S, Patel B, Patel M. Fabrication, optimization and evaluation of docetaxel and curcumin Co-loaded nanostructured lipid carriers for improved antitumor activity against non-small cell lung carcinoma (Sep 10.1080/02652048.2020. 1823498, 2020). J Microencapsul. 2020;37(8):640 [CrossRef] | [Google Scholar]
- Kumar K, Rani V, Mishra M, Chawla R. New paradigm in combination therapy of siRNA with chemotherapeutic drugs for effective cancer therapy. Curr Res Pharmacol Drug Discov. 2022;3:100103 [PubMed] | [CrossRef] | [Google Scholar]
- Yao Y, Zhou Y, Liu L, Xu Y, Chen Q, Wang Y, et al. Nanoparticle-based drug delivery in cancer therapy and its role in overcoming drug resistance. Front Mol Biosci. 2020;7:193 [PubMed] | [CrossRef] | [Google Scholar]
- Ren WX, Han J, Uhm S, Jang YJ, Kang C, Kim JH, et al. Recent development of biotin conjugation in biological imaging, sensing and target delivery. Chem Commun (Camb). 2015;51(52):10403-18. [PubMed] | [CrossRef] | [Google Scholar]
- Uram Ł, Filipowicz A, Misiorek M, Pieńkowska N, Markowicz J, Wałajtys-Rode E, et al. Biotinylated PAMAM G3 dendrimer conjugated with celecoxib and/or Fmoc-l-Leucine and its cytotoxicity for normal and cancer human cell lines. Eur J Pharm Sci. 2018;124:1-9. [PubMed] | [CrossRef] | [Google Scholar]
- Liu M, Wang B, Guo C, Hou X, Cheng Z, Chen D, et al. Novel multifunctional triple folic acid, biotin and CD44 targeting pH-sensitive nano-actiniaes for breast cancer combinational therapy. Drug Deliv. 2019;26(1):1002-16. [PubMed] | [CrossRef] | [Google Scholar]
- Luo J, Meng X, Su J, Ma H, Wang W, Fang L, et al. Biotin-modified polylactic-co-glycolic acid nanoparticles with improved antiproliferative activity of 15, 16-dihydrotanshinone I in human cervical cancer cells. J Agric Food Chem. 2018;66(35):9219-30. [PubMed] | [CrossRef] | [Google Scholar]
- Maiti S, Paira P. Biotin conjugated organic molecules and proteins for cancer therapy: a review. Eur J Med Chem. 2018;145:206-23. [PubMed] | [CrossRef] | [Google Scholar]
- Chen S, Zhao X, Chen J, Chen J, Kuznetsova L, Wong SS, et al. Mechanism-based tumor-targeting drug delivery system. Validation of efficient vitamin receptor-mediated endocytosis and drug release. Bioconjug Chem. 2010;21(5):979-87. [PubMed] | [CrossRef] | [Google Scholar]
- Tripodo G, Mandracchia D, Collina S, Rui M, Rossi D. New perspectives in cancer therapy: the biotin-antitumor molecule conjugates. Med Chem. 2014;8:1-4. [CrossRef] | [Google Scholar]
- Vadlapudi AD, Vadlapatla RK, Mitra AK. Sodium dependent multivitamin transporter (SMVT): a potential target for drug delivery. Curr Drug Targets. 2012;13(7):994-1003. [PubMed] | [CrossRef] | [Google Scholar]
- Jaracz S, Chen J, Kuznetsova LV, Ojima I. Recent advances in tumor-targeting anticancer drug conjugates. Bioorg Med Chem. 2005;13(17):5043-54. [PubMed] | [CrossRef] | [Google Scholar]
- Chen S, Zhao X, Chen J, Chen J, Kuznetsova L, Wong SS, et al. Mechanism-based tumor-targeting drug delivery system. Validation of efficient vitamin receptor-mediated endocytosis and drug release. Bioconjug Chem. 2010;21(5):979-87. [PubMed] | [CrossRef] | [Google Scholar]
- Russell-Jones G, McTavish K, McEwan J, Rice J, Nowotnik D. Vitamin-mediated targeting as a potential mechanism to increase drug uptake by tumours. J Inorg Biochem. 2004;98(10):1625-33. [PubMed] | [CrossRef] | [Google Scholar]
- Russell-Jones G, McEwan J. inventors; Access Pharmaceuticals Australia Pty Ltd, assignee. Amplification of biotin-mediated targeting. United States patent application US. 2006;10(535):269 [PubMed] | [CrossRef] | [Google Scholar]
- Anwar W, Dawaba HM, Afouna MI, Samy AM. Screening study for formulation variables and characterization of candesartan cilexetil loaded nanostructured lipid carriers. Pharm Res. 2019;4:8-19. [CrossRef] | [Google Scholar]
- Cortesi R, Valacchi G, Muresan XM, Drechsler M, Contado C, Esposito E, et al. Nanostructured lipid carriers (NLC) for the delivery of natural molecules with antimicrobial activity: production, characterisation and in vitro studies. J Microencapsul. 2017;34(1):63-72. [PubMed] | [CrossRef] | [Google Scholar]
- Khan SA, Rehman S, Nabi B, Iqubal A, Nehal N, Fahmy UA, et al. Boosting the brain delivery of atazanavir through nanostructured lipid carrier-based approach for mitigating neuroaids. Pharmaceutics. 2020;12(11):1059 [PubMed] | [CrossRef] | [Google Scholar]
- Subramaniam B, Siddik ZH, Nagoor NH. Optimization of nanostructured lipid carriers: understanding the types, designs and parameters in the process of formulations. J Nanopart Res. 2020;22(6):1-29. [CrossRef] | [Google Scholar]
- Chand P, Kumar H, Badduri N, Gupta NV, Bettada VG, Madhunapantula SV, et al. Design and evaluation of cabazitaxel loaded NLCs against breast cancer cell lines. Colloids Surf B Biointerfaces. 2021;199:111535 [PubMed] | [CrossRef] | [Google Scholar]
- Qu CY, Zhou M, Ying-wei C, Chen Mei-mei, Shen Feng, Xu Lei-Ming, et al. Engineering of lipid prodrug-based, hyaluronic acid-decorated nanostructured lipid carriers’ platform for 5-fluorouracil and cisplatin combination gastric cancer therapy. Int J Nanomedicine. Array:3911-20. [CrossRef] | [Google Scholar]
- Budhiraja M, Zafar S, Akhter S, Alrobaian M, Rashid MA, Barkat MA, et al. Mupirocin-loaded chitosan microspheres embedded in Piper betle extract containing collagen scaffold accelerate wound healing activity. AAPS PharmSciTech. 2022;23(3):77 [PubMed] | [CrossRef] | [Google Scholar]
- Yang G, Wu F, Chen M, Jin J, Wang R, Yuan Y, et al. Formulation design, characterization and and evaluation of nanostructured lipid carriers containing a bile salt for oral delivery of gypenosides. Int J Nanomedicine. 2019;14:2267-80. [PubMed] | [CrossRef] | [Google Scholar]
- Sakellari GI, Zafeiri I, Batchelor H, Spyropoulos F. Formulation design, production and characterisation of solid lipid nanoparticles (SLN) and nanostructured lipid carriers (NLC) for the encapsulation of a model hydrophobic active. Food Hydrocoll Health. 2021;1:None [PubMed] | [CrossRef] | [Google Scholar]
- Dandagi PM, Dessai GA, Gadad AP, Desai VB. Formulation and evaluation of nanostructured lipid carrier (NLC) of lornoxicam. Int J Pharm Pharm Sci. 2014;6(2):73-7. [PubMed] | [CrossRef] | [Google Scholar]
- Taymouri S, Alem M, Varshosaz J, Rostami M, Akbari V, Firoozpour L, et al. Biotin decorated sunitinib loaded nanostructured lipid carriers for tumor targeted chemotherapy of lung cancer. J Drug Deliv Sci Technol. 2019;50:237-47. [CrossRef] | [Google Scholar]
- Badhwar R, Singh R, Popli H. Implementation of quality by design (qbd) approach in development of qct-smedds with combination of agnps for diabetic foot ulcer management. Indian J Pharm Educ Res. 2021;55:1207-23. [CrossRef] | [Google Scholar]
- Elmowafy M, Samy A, Raslan MA, Salama A, Said RA, Abdelaziz AE, et al. Enhancement of bioavailability and pharmacodynamic effects of thymoquinone via nanostructured lipid carrier (NLC) formulation. AAPS PharmSciTech. 2016;17(3):663-72. [PubMed] | [CrossRef] | [Google Scholar]
- Anarjan N, Tan CP. Effects of selected polysorbate and sucrose ester emulsifiers on the physicochemical properties of astaxanthin nanodispersions. Molecules. 2013;18(1):768-77. [PubMed] | [CrossRef] | [Google Scholar]
- Thapa C, Ahad A, Aqil M, Imam SS, Sultana Y. Formulation and optimization of nanostructured lipid carriers to enhance oral bioavailability of telmisartan using Box-Behnken design. J Drug Deliv Sci Technol. 2018;44:431-9. [CrossRef] | [Google Scholar]
- Ameeduzzafar ANK, Alruwaili NK, Imam SS, Alotaibi NH, Alhakamy NA, Alharbi KS, et al. Formulation of chitosan polymeric vesicles of ciprofloxacin for ocular delivery: box-Behnken optimization, in vitro characterization, HET-CAM irritation and antimicrobial assessment. AAPS PharmSciTech. 2020;21(5):167 [PubMed] | [CrossRef] | [Google Scholar]
- Danaei MR, Dehghankhold M, Ataei S, Hasanzadeh Davarani F, Javanmard R, Dokhani A, et al. Impact of particle size and polydispersity index on the clinical applications of lipidic nanocarrier systems. Pharmaceutics. 2018;10(2):57 [PubMed] | [CrossRef] | [Google Scholar]
- Nagaich U, Gulati N. Nanostructured lipid carriers (NLC) based controlled release topical gel of clobetasol propionate: design and characterization. Drug Deliv Transl Res. 2016;6(3):289-98. [PubMed] | [CrossRef] | [Google Scholar]
- Alam T, Khan S, Gaba B, Haider MF, Baboota S, Ali J, et al. Adaptation of quality by design-based development of isradipine nanostructured-lipid carrier and its evaluation for gut permeation and solubilization fate. J Pharm Sci. 2018;107(11):2914-26. [PubMed] | [CrossRef] | [Google Scholar]
- Gardouh AR, Gad S, Ghonaim HM, Ghorab MM. Design and characterization of glyceryl monostearate solid lipid nanoparticles prepared by high shear homogenization. Br J Pharm Res. 2013;3(3):326-46. [CrossRef] | [Google Scholar]
- Soldati PP, Polonini HC, Paes CQ, Restrepob JA, Creczynksi-Pasa TB, Chaves MG, et al. Controlled release of resveratrol from lipid nanoparticles improves antioxidant effect. IFAC-PapersOnLine. 2018;51(27):16-21. [CrossRef] | [Google Scholar]
- Luo Y, Teng Z, Li Y, Wang Q. Solid lipid nanoparticles for oral drug delivery: chitosan coating improves stability, controlled delivery, mucoadhesion and cellular uptake. Carbohydr Polym. 2015;122:221-9. [PubMed] | [CrossRef] | [Google Scholar]
- Corsaro C, Neri G, Mezzasalma AM, Fazio E. Weibull modeling of controlled drug release from Ag-PMA nanosystems. Polymers. 2021;13(17):2897 [PubMed] | [CrossRef] | [Google Scholar]
- Mozafari MR, Pardakhty A, Azarmi S, Jazayeri JA, Nokhodchi A, Omri A, et al. Role of nanocarrier systems in cancer nanotherapy. J Liposome Res. 2009;19(4):310-21. [PubMed] | [CrossRef] | [Google Scholar]
- Danaei MR, Dehghankhold M, Ataei S, Hasanzadeh Davarani F, Javanmard R, Dokhani A, et al. Impact of particle size and polydispersity index on the clinical applications of lipidic nanocarrier systems. Pharmaceutics. 2018;10(2):57 [PubMed] | [CrossRef] | [Google Scholar]
- Eggersdorfer ML, Pratsinis SE. The structure of agglomerates consisting of polydisperse particles. Aerosol Sci Technol. 2012;46(3):347-53. [PubMed] | [CrossRef] | [Google Scholar]
- Teeranachaideekul V, Souto EB, Junyaprasert VB, Müller RH. Cetyl palmitate-based NLC for topical delivery of Coenzyme Q10-Development, physicochemical characterization and in vitro release studies. Eur J Pharm Biopharm. 2007;67(1):141-8. [PubMed] | [CrossRef] | [Google Scholar]
- Wang Y, Li P, Kong L. Chitosan-modified PLGA nanoparticles with versatile surface for improved drug delivery. AAPS PharmSciTech. 2013;14(2):585-92. [PubMed] | [CrossRef] | [Google Scholar]