ABSTRACT
Magnetic Nanoparticles (MNPs) are now being utilized more frequently in experimental research on cancer treatments, which has become one of the most difficult problems in medical science. The fundamental objective of this research is to use nanotechnology to effectively treat and eradicate advanced or metastatic cancer with the fewest possible negative effects. The fact that MNPs can be bound to chemotherapeutic medications, nucleic acids, synthesized antibodies, or radionuclide compounds is taken into account by drug delivery methods. Under the influence of an external magnetic field, MNPs can be guided and various treatment modalities can be used. This research examines the function of MNPs in cancer imaging and diagnostics as well as several MNP manufacturing techniques. In addition to all of these, information on the use of MNPs for the treatment of breast malignancies from clinical trials and scholarly prospection has been compiled.
INTRODUCTION
Breast cancer is a common diagnosis among women. When it comes to the glandular tissue of the breast, the epithelium lining the ducts accounts for 85% of breast cancer cases and the lobules for 15%. In its early stages, while it is still contained inside the duct or lobule (“in situ”), the malignant growth is usually asymptomatic and poses little danger of metastasis. Stage 0 tumors can progress to invasive breast cancer if they infect nearby breast tissue and metastasis can occur either locally (to nearby lymph nodes) or far (to other organs) from the original site of the tumor.1,2 When caught early, breast cancer treatment has a good chance of success. Breast cancer patients often undergo a multi-pronged approach to treatment, including hormone therapy, chemotherapy, targeted biological therapy, radiation therapy and surgical excision of the tumor. This allows doctors to address the microscopic cancer that has spread via the blood from the tumor. By halting the progression of cancer, this therapy has the potential to save lives.3,4
While 2.3 million women were diagnosed with breast cancer in 2020, 6,85,000 peoples lost their lives to the disease globally. By 2020, 7.8 million women will have survived a breast cancer diagnosis during the past five years, making it the most frequent malignancy globally shows that this causes just 6.9% of cancer-related fatalities, which is encouraging.5,6 The breast cancer prevalence fell from 1 in 11 in 1975 to 1 in 8 in 2022.7 When compared to other cancers, breast cancer is the leading cause of Disability-Adjusted Life Years (DALYs) for females throughout the world. Breast cancer in women after puberty is on the rise globally and this trend holds true across all age groups.8 Many of these tumors are known as triple-negative breast cancers because they do not overexpress the oestrogen, progesterone, or human epidermal growth factor receptors.9,10 Such malignancies can be effectively treated with chemotherapy, although chemotherapy is almost always accompanied by a variety of adverse effects.11–14 One of the most significant scientific research endeavors today is nanoscience.
Scientists, engineers, chemists and doctors are beginning to be able to operate at the molecular and cellular levels thanks to nanotechnology, which is allowing them to make enormous advances in healthcare and the life sciences. There are several advantages to employing Nanoparticle [NP] materials because of their unique size and physicochemical properties. The wide range of applications for Magnetic Nanoparticles (MNPs) in areas such as biotechnology, biomedicine, engineering, materials science and environmental protection has sparked significant interest in their production.15?
Magnetic nanoparticles
Magnetic Nanoparticles (MNPs) offer immense potential in medical applications owing to their unique properties. Their small size, quantum characteristics and high surface-to-volume ratio enable them to transport other compounds effectively.16–19 Ferritin, the predominant iron storage protein, contains thousands of ferric ions in a paramagnetic oxyhydroxide core, utilized by migratory animals for geomagnetic navigation. MNPs are ubiquitous in biological systems, exhibiting magnetic characteristics derived from electron orbital and spin movements. Interactions between magnetic moments yield magnetic order below a critical temperature. MNPs, being smaller, exhibit superparamagnetic properties, where thermal energy randomizes magnetic moments, resulting in zero overall magnetization in the absence of an external field. However, MNPs can amplify magnetic moments significantly compared to paramagnetic materials, maintaining colloidal stability due to absence of remanent magnetization.
MNPs with a hydrodynamic diameter below 5 nm facilitate rapid blood circulation and can evade opsonization by reticuloendothelial system, enhancing permeability and retention effects in tumors. Sized between 10 to 100 nm, MNPs accumulate at tumor sites due to leaky vasculature and inefficient lymphatic drainage, making them suitable for localized diagnostic and imaging applications. In cancer treatment, MNPs enable tailored therapies, reducing systemic side effects of chemotherapy and radiation therapy, minimizing invasiveness of surgeries and improving detection of malignant lesions.20–23 MNPs thus pave the way for personalized and targeted oncological interventions, offering a promising avenue for improved patient outcomes and reduced adverse effects in cancer treatment.
Properties of MNPS
It is generally believed that MNPs exhibit ferrimagnetic, ferromagnetic, or superparamagnetic behavior. Superparamagnetic particles below a certain nanoparticle size that depends on the nature of material are known as MNPs (zero magnetic moment) when an external magnetic field is not present. Magnetic Nanoparticles (MNPs) undergo rapid magnetic moment growth and orientation perpendicular to the applied magnetic field.24
Superparamagnetic Iron Oxide Nanoparticles (SPIONs) are crucial in medical applications such as magnetic drug targeting, MRI and cancer therapies. These nanoparticles exhibit no magnetic properties once the external magnetic field is eliminated, effectively preventing the formation of aggregates. The use of superparamagnetic nanoparticles in medical applications provides a significant level of control as a result of their strong response to external fields. The characterization of MNPs involves the determination of several key parameters. These parameters include the maximum induced Magnetization (Ms), which represents the highest level of magnetization achieved by the MNPs. Another important parameter is the remanent Magnetization (Mr), which is the magnetization that remains in the MNPs after the magnetic field, is removed. Lastly, the coercivity (Hc) is a measure of the magnetic field strength required to demagnetize the MNPs. Superparamagnetic materials exhibit a sigmoidal dependence M(H) and do not experience hysteresis.25
Ferrimagnetic and ferromagnetic materials demonstrate a superparamagnetic behavior once they reach a specific temperature called the blocking temperature. The saturation magnetization is determined by the temperature. The manner in which this behavior is altered when distinguishing between resting time and measuring time is as follows: If the relaxation time is less than the measurement time, the particles are considered to be in a superparamagnetic state. Otherwise, they are operating under ferrimagnetic or ferromagnetic conditions.26 During the synthesis process, if the diameter of the MNPs is maintained below a specific threshold, they exhibit a single domain structure. At this minimum diameter, the MNPs exist in a superparamagnetic state. Particles exhibit a higher likelihood of generating a multi-domain magnetic structure once they surpass a critical diameter. The diameter at which superparamagnetic activity first manifests itself (Ds) is around 25 nm, while the critical diameter for the mono-domain magnetic structure (Dc) for Fe3O4 particles is 82 nm. The values for Ds and Dc in the case of Fe2O32 are 30 nm and 90 nm, respectively.27
Whether MNPs exhibit magnetic properties is dependent on the number of unpaired valence electrons linked to the metal atoms or ions. Chemical composition is a crucial factor that depends on the concentration of the precursor, the synthesis process and the type of dopant. Since the solvent may have an impact on the magnetic state, it was noted in that Mn-doped ZnO turned paramagnetic when benzyl alcohol was employed, whereas Co-doped MNPs displayed ferromagnetic characteristics.28
Role of MNPS in Imaging and Diagnosis on Cancer
Pancreas
Due to its late manifestation, pancreatic cancer is one of the deadliest tumours in the world.29 Early diagnosis will thereby boost the percentage of people who recover. The MNPs function-alized with ASON result in specific localisation in pancreatic cancers when chitosan-coated MNPs and Survivin Antisense Oligonucleotides (ASON) are coupled to create Sur MNPs.30 MRI could detect pancreatic cancers using survivin targeted NPs. The production of biodegradable Nanoparticles (NPs) involved the utilization of recombinant human serum albumin and Iron Oxide (maghemite, Fe2O3) NPs. This was done with the consideration of advancing diagnostic tools that are more sensitive, allowing for early medical imaging. The utilization of SPECT CT and MRI demonstrated enhanced targeting and imaging capabilities in mice.
Breast
In order to improve the microwave imaging of breast cancer, Bucci et al study’s31 presented certain parameters for the construction of the imaging apparatus for MNPs. The results indicate that MNP-enhanced microwave imaging has the ability to accurately detect cancer lesions, even when using low complexity setups, as long as it is developed in accordance with the established criteria. The study conducted by Kato et al.32 found that the use of liposome encapsulation significantly improved the transport and retention of Superparamagnetic Iron Oxide Nanoparticles (SPION) in breast tumors. Additionally, the targeted delivery of SPION liposomes resulted in a substantial increase in their accumulation within breast cancers. These findings suggest that SPION liposomes are the most suitable option for identifying breast tumors using Magnetic Resonance Imaging (MRI). In their study, Sun et al.33 demonstrated that there was a higher accumulation of Fluorescent Magnetic Nanoparticles (FMNPs) containing the peptide cyclic arginine Glycine aspartic acid (RGD) around the tumors compared to FMNPs. The FMNPs refer to magnetic nanoclusters that are coated with ruthenium (II) complexes that have been doped with silica. The results indicate that RGD FMNPs have the potential to serve as a molecular targeting probe for the detection of breast cancer using MRI and OI. Bevacqua and Scapaticci34 proposed a compressive sensing methodology for three-dimensional microwave imaging of breast cancer with the aim of enhancing the accuracy and precision of breast cancer diagnosis. The authors of this work developed a compressive sensing algorithm by leveraging their understanding of the highest concentration of MNPs that can be targeted in human tissues.
Prostate
Magnetic Resonance Imaging (MRI) is considered the optimal imaging modality for patients diagnosed with prostate cancer.35 This is due to its exceptional ability to provide detailed visualization of soft tissues, making it an essential tool in the management of prostate cancer. The evaluation of Sentinel Lymph Nodes (SLN) in prostate cancer patients often involves the use of lymphoscintigraphy following the injection of radiolabelled tracers.36 Prior to and one day after the administration of Superparamagnetic Iron Oxide Nanoparticles (SPION), patients underwent Magnetic Resonance Imaging (MRI) using a 1.5 Tesla scanner. The MRI scans included T1-weighted, T2-weighted and T2-weighted sequences. The present study introduced a Novel Technique Involving the Intraprostatic Injection of Superparamagnetic Iron Oxide nanoparticles (SPIONs) for the purpose of visualizing Sentinel Lymph Nodes (SLNs) using Magnetic Resonance Imaging (MRI) in patients diagnosed with prostate cancer. The study conducted by Winter et al. provides evidence that the use of transrectal intraprostatic injection of Superparamagnetic Iron Oxide Nanoparticles (SPIONs) for magnetic labeling of prostate cancer is a viable, secure and efficient approach to detect Sentinel Lymph Nodes (SLNs) and lymph node metastases in most patients. according to research by Winter et al.37 The Gleason score, a histological indicator of prostate cancer aggressiveness, can sometimes correlate with the sensitivity of Diffusion Weighted (DW) MRI to water diffusion in tissues. Utilizing DW MRI-based prostate cancer stratification and surveillance may enhance the accuracy of preclinical studies involving mice with transgenic mouse prostate adenocarcinomas.38
Lung
The sensitivity of lung cancer metastases detection was enhanced by Wan et al. through the utilization of immunological Superparamagnetic Iron Oxide Nanoparticles (SPIONs) for magnetic resonance immune imaging. The Superparamagnetic Iron Oxide Nanoparticles (SPIONs) underwent a coating process using oleic acid and carboxymethyl dextran. Subsequently, they were connected to a monoclonal antibody specific to Cluster of Differentiation (CD) 44v6 in mice.39 The synthesized Superparamagnetic Iron Oxide Nanoparticles (SPIONs) have the potential to be utilized in the detection and localization of lung tumors. The utilization of targeted pulmonary inhalation aerosol-based delivery enables the controlled administration of medications directly to the lungs, offering various advantages.37 The viability of using Magnetic Particle Imaging (MPI) for pulmonary imaging and measuring mucociliary clearance in the lung was examined by Nishimoto et al. through small animal experiments. Nebulized Magnetic Nanoparticles (MNPs) were utilized for this purpose.40
Therapeutic strategies
Therapeutic strategies employing Magnetic Nanoparticles (MNPs) in the treatment of breast cancer hold significant promise and are a subject of extensive research. These strategies leverage the unique properties of MNPs to target cancer cells specifically while minimizing damage to healthy tissues, thereby improving treatment efficacy and reducing side effects. Below are several therapeutic approaches utilizing MNPs in breast cancer treatment:
Magnetic Hyperthermia
MNPs can generate heat when exposed to an alternating magnetic field. This property is utilized in magnetic hyperthermia, where MNPs are selectively delivered to tumor sites and activated with an external magnetic field to induce localized hyperthermia. The elevated temperatures selectively kill cancer cells, making this approach an effective treatment option for breast cancer.
Drug Delivery
MNPs can serve as carriers for chemotherapeutic drugs, allowing for targeted drug delivery to tumor sites. Functionalized MNPs can be loaded with anticancer drugs and guided to the tumor site using an external magnetic field. This approach enhances drug accumulation at the tumor site while minimizing systemic toxicity, improving treatment outcomes.
Gene Therapy
MNPs can also be employed for targeted gene delivery to breast cancer cells. Functionalized MNPs can carry therapeutic genes or RNA interference molecules to the tumor site, enabling selective gene modulation within cancer cells. This approach holds promise for the development of innovative gene-based therapies for breast cancer.
Magnetic Resonance Imaging (MRI) Contrast Agents
MNPs possess magnetic properties that make them excellent contrast agents for MRI. In breast cancer diagnosis and monitoring, MNPs can be functionalized and injected into the patient to enhance MRI imaging of tumor tissues. This enables early detection, accurate staging and monitoring of treatment response in breast cancer patients.
Combination Therapies
MNPs can be integrated into multimodal treatment approaches for breast cancer, combining magnetic hyperthermia with chemotherapy, radiation therapy, or immunotherapy. These combination therapies synergistically enhance treatment efficacy by targeting cancer cells through multiple mechanisms while minimizing adverse effects on healthy tissues.41
Overall, therapeutic strategies utilizing MNPs offer promising avenues for the treatment of breast cancer. Continued research and development in this field hold the potential to revolutionize breast cancer therapy, leading to improved patient outcomes and quality of life.
Applications of MNPS in Drug Delivery System
Figure 1 shows various methods for treating cancer that combine MNPs or SPIONs with chemotherapy, radiation, immunotherapy and gene therapy. MNPs offer the chance to create medication delivery systems that are specialised for their target. The therapeutic potential of current chemotherapy regimens is constrained by nonspecific toxicities. The mechanisms for optimising MNPs for selective, targeted medication delivery have been the subject of numerous investigations. There are a number of factors that need to be taken into account while dealing with MNP. Factors such as medication loading capacity, target release profile, water dispersion stability, biocompatibility with cells and tissues and magnetic feature retention after polymer or chemical reaction modification are important.
There have been several investigations into how chemotherapeutic medicines release in vitro. The goal of these efforts has been to develop MNP conjugates that enable precise targeting and have antiproliferative cancer effects. In vitro success has been shown in this research. A unique formulation of iron oxide magnetic nanoparticles coated in Oleic Acid (OA) and Pluronic was created by Jain et al.42 that could be easily loaded with large amounts of chemotherapeutic medicines that are not water soluble. They demonstrated that DOX-loaded nanoparticles were able to be internalized by MCF-7 cells, where they exhibited antiproliferative effectiveness that was dose dependent and exhibited long-lasting intracellular retention. Kohler et al.43 developed a drug-nanoparticle conjugate by affixing Methotrexate (MTX) to the iron oxide nanoparticle. They prevented intravenous MTX release from the nanoparticles by altering their surface using a peptide link. The conditions within the lysosomal compartment cause the amide bond to be broken. With its low pH and lysozymes, this environment is representative of what’s found inside the target cells. Research has demonstrated that the overexpression of folate receptors on tumor cells, as compared to healthy cells, is responsible for the increased absorption of MTX-conjugated nanoparticles.
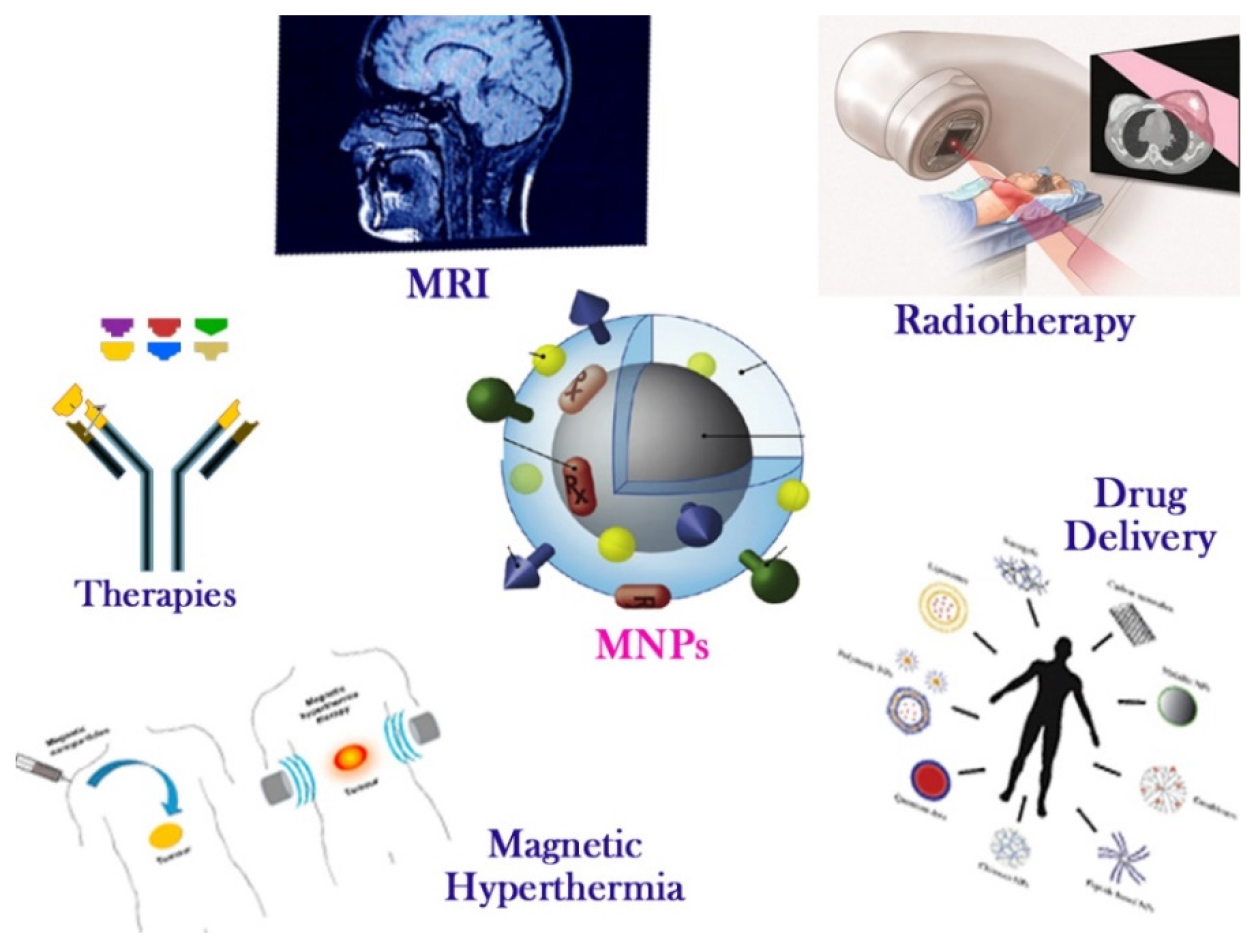
Figure 1:
Schematic representation of magnetic nanoparticles’ applications in oncology.
Munnier et al.44 produced SPIO aqueous suspensions by coprecipitating ferric and ferrous chlorides in an alkaline solution, then oxidizing the surface with ferric nitrate and treating it with citrate ions. A pre-formed DOX-Fe2+ combination were used to introduce the DOX to the ferrofluids. We were able to get loading levels of up to 14% using this strategy. Even at low doses, the DOX-loaded SPIO exhibited cytotoxicity against the MCF-7 breast cancer cell line in vitro, which was on par with or higher than that of solution-based DOX. Benyettou et al.45 combined zoledronate with SPIO for its administration. The therapeutic target was shifted from promoting Zoledronate buildup in bone to improving its absorption by the intestines once it was attached to the nanoparticles’ surface. The conjugate’s antiproliferative effectiveness was further established in in vitro testing against MD-MB 231. Using an iron oxide core coated in Oleic Acid (OA) and subsequently with OA-PEG (polyethylene glycol), Yallapu et al.46 synthesized MNPs that could be dissolved in water. The process of doxorubicin hydrochloride being converted into a water-insoluble base allowed it to partition into the OA layer. The antiproliferative effects were dosage dependent, according to in vitro tests conducted on the MCF-7 breast cancer cell line.
Synthesis Methods for MNPS
There are certain significant limitations that apply to biological applications that must be considered. Specialized and dedicated nanoparticles are needed for every medical inquiry and treatment. The correlation between nanoparticle size and shape and magnetic properties, as well as surface effects and crystallinity grade, must be considered.
Right now, a magnetic nanoparticle’s optimal shape is spherical or quasi-spherical. The aggregation condition of the precursors allows for the division of the synthesis process into three main types (gaseous, aqueous, or solid). As a result of the process type, another classification can be carried out (chemical, physical, or biological). Co-precipitation, the sol-gel method and hydrothermal synthesis are the three most significant synthesis techniques described in the literature.47 New techniques were also created, including microemulsion, thermal breakdown, sonolysis and biosynthesis.48 The primary synthesis techniques that are applied in practise are shown in Figure 2. Co-precipitation is one of the most frequently used chemical processes. Both an open and a closed environment can be employed to create the nanoparticles used in this procedure.49–51 Some of the steps that make up the open medium include gaseous pathways, solid chemical reactions, co-precipitation and the usage of heated organic solvents. The closed media, on the other hand, makes use of microemulsions in either oil or water with organic or inorganic porous templates.52
Scholarly prospection
The findings of a scientific prospectus from 2012 to 2022 that was created using the PubMed database with the search keywords “(magnetic nanoparticles [Title/Abstract]) AND (breast cancer [Title/Abstract])” and “(magnetic nanoparticles [Title/Abstract])”.
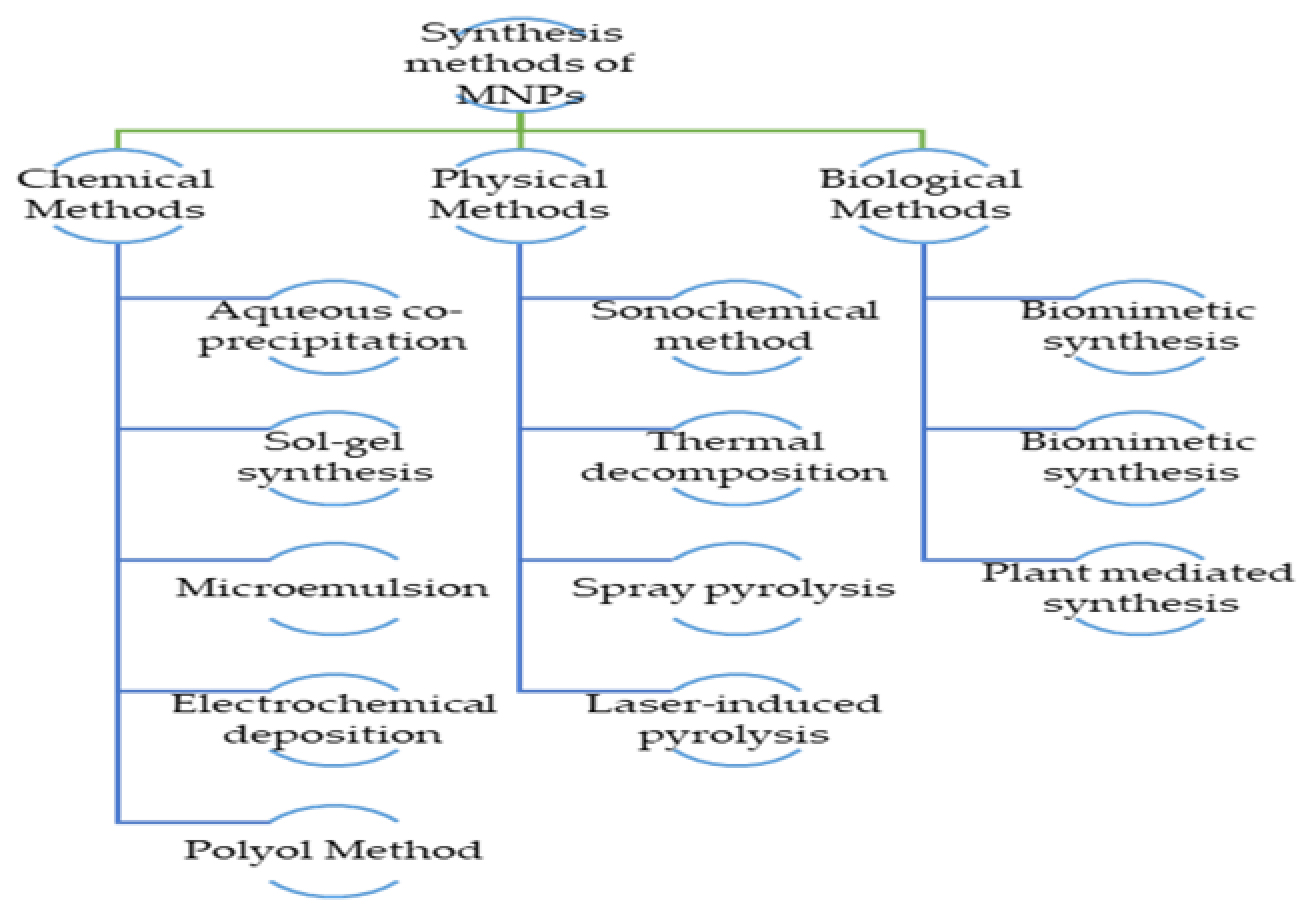
Figure 2:
The primary synthesis techniques that are applied in practice.
Results showed 288 articles on magnetic nanoparticles and breast cancer. Over the same time span, 9446 papers were identified that made reference to magnetic nanoparticles. There have been just eight articles concerning the use of MNPs for breast malignancies since 2012. However, since 2015, the figures have risen significantly shown in Figure 3. There have been 19 manuscripts so far in 2022 and by year’s conclusion that number will have risen to 40. The overall number of manuscripts on MNPs in 2012 was just 547, yet this trend was rather fascinating. In the years following 2018, the number of publications skyrockets, reaching 1043 in 2019, 1161 in 2020, 1125 in 2021 and 612 in 2022. This is proven by the vast amount of research conducted on MNPs, which is justified by their relevance and widespread use in several sectors such as pharmaceuticals, biomedical materials science, microbiology, immunology, food and biotechnology and many more.53
Clinical Trials Information on Application of Mnps for Treatment of Breast Cancers
The researchers are always in line to invent new drug moieties or technologies for the efficient treatment of breast cancers. We found three studies by search using- “Breast Cancer” in condition or disease and “magnetic nanoparticles” in other terms. A detailed description of clinical trials is portrayed in (Table 1).
Sl. No. | Title | Status | Study Results | Conditions | Interventions | Locations |
---|---|---|---|---|---|---|
1 | Sentinel Node Localization and Staging with Low Dose Superparamagnetic Iron Oxide. | Recruiting Clinical trial No: NCT05359783. | No Results Available. | Breast Cancer| Sentinel Lymph Node. | Drug: Superparamagnetic Iron Oxide. | Sahlgrenska University Hospital, Gothenburg, Sweden. |
2 | Phase II NCT (Neoadjuvant Chemotherapy) w/ Weekly Abraxane in Combination with Carboplatin and Bevacizumab in Breast Cancer. | Completed Clinical trial No: NCT00675259. | Has Results | Breast Cancer | Drug: bevacizumab| Drug: carboplatin| Drug: nab-paclitaxel| Procedure: Surgery| Drug: Adjuvant chemotherapy. | Ohio State University Medical Center, Columbus, Ohio, United States. |
3 | Magseed and Magtrace Localization for Breast Cancer. | Recruiting Clinical trial No: NCT05161507. | No Results Available. | Breast Carcinoma|Breast Carcinoma Metastatic in Lymph Node. | Procedure: Magseed| Procedure: Magtrace|Procedure: Axillary lymph node metastasis-needle biopsy. | University Hospital Ostrava, Ostrava, Moravian-Silesian Region, Czechia. |
Treatment decisions for breast cancer patients are greatly influenced by the Sentinel Lymph Node (SLN) status. The current gold standard for SLN detection is a dual method utilizing Blue Dye (BD) and Technetium 99m (Tc99). But SPIO nanoparticles, which are superparamagnetic iron oxide, have shown the same reliability. The feasibility of SLN detection with an ultra-low dose SPIO was the primary objective of this work. Phase Ib/II interventional trial with a single arm that aims to increase the dosage. Doses of SPIO will be escalted from 0.1 mL to 0.5 mL, with a minimum of 5 patients each step. Once four or more operations have been successfully completed, no further dosage escalation will be carried out. We will include 30 individuals with the lowest effective dosage. Secondary aim A will evaluate the feasibility of using SPIO-enhanced axillary MRI to map and stage sentinel lymph nodes; it will involve 20 patients who received the least effective dosage. We will include extra 20 patients with the least effective dose of SPIO to see if low doses of SPIO induce any SPIO-related abnormalities on breast MRI after breast conserving surgery. Both Objective A and Objective B are optional components of this study and patients will be specifically asked about their involvement in both. During the course of the research, all individuals will have their skin stains checked for.
A clinical trial named Phase II NCT (Neoadjuvant Chemotherapy) with Weekly Abraxane in Combination with Carboplatin and Bevacizumab in Breast Cancer (ClinicalTrials.gov Identifier: NCT00675259) is being supported by the Ohio State University Comprehensive Cancer Center. Carboplatin and paclitaxel albumin-stabilized nanoparticle formulation are two examples of chemotherapy medications that limit tumor cell development. These treatments have several mechanisms of action, including cell death and growth inhibition. Different mechanisms exist for how monoclonal antibodies, like bevacizumab, can stop the growth of tumours. Some people locate tumour cells and aid in their destruction or deliver cancer-curing agents to them. Others prevent tumour cells from proliferating and spreading. By preventing blood supply to the tumour, bevacizumab may help slow the spread of breast cancer. Before surgery, bevacizumab plus combination chemotherapy may shrink the tumour, minimising the amount of healthy tissue that needs to be removed. Any tumour cells that are still present after surgery may be eliminated by bevacizumab treatment. For women having breast cancer surgery for stages II or III, this phase II trial is looking at how well paclitaxel albumin-stabilized nanoparticle formulation, carboplatin and bevacizumab work.
Tc99 was aimed at being compared to the Sentimag localization device and its tracer Magtrace, which consists of superparamagnetic iron oxide nanoparticles, in a single-center prospective trial known as ClinicalTrial-NCT05161507. It also aims to explain why and how skin darkening that occurs after a Magtrace injection fades. Injecting the Magtrace is done before to surgery. We will do sentinel node biopsies and compare the two methods’ detection rates. Second, in the trial, tiny, non-radioactive seeds called Magseed will be placed into the invisible breast cancer tumors. The surgical site of the seed can be pinpointed with the use of the Sentimag probe. Another part of the study involves implanting Magseed in the positive axillary lymph nodes of patients who will undergo neoadjuvant systemic treatment and have clinically positive lymph nodes. Prior to neoadjuvant systemic treatment, it is essential to document the positive lymph node(s) so that they may be later identified for accurate assessment of the breast and axilla responses. The accuracy of Sentinel Lymph Node Biopsy (SLNB) might be compromised if systemic treatment has a negative impact on lymphatic outflow. Targeted axillary dissection, which combines SLNB with the removal of the previously positive target lymph node, makes the operation far more accurate and reduces patient morbidity. Another part of the study is to make sure that the breast tumor labeling with Magseed remains stable between the start of neoadjuvance and the procedure that follows the patient receiving oncological preparation. Traditional Tc99 detection will be performed on all patients’ sentinel lymph nodes. Under ultrasound supervision, the Magseed marker is injected into a lymph node or a breast cancer lesion that is not yet visible. Our surgical clinic follows up with patients six to thirty days following their procedure for check-ups once the trial is over.
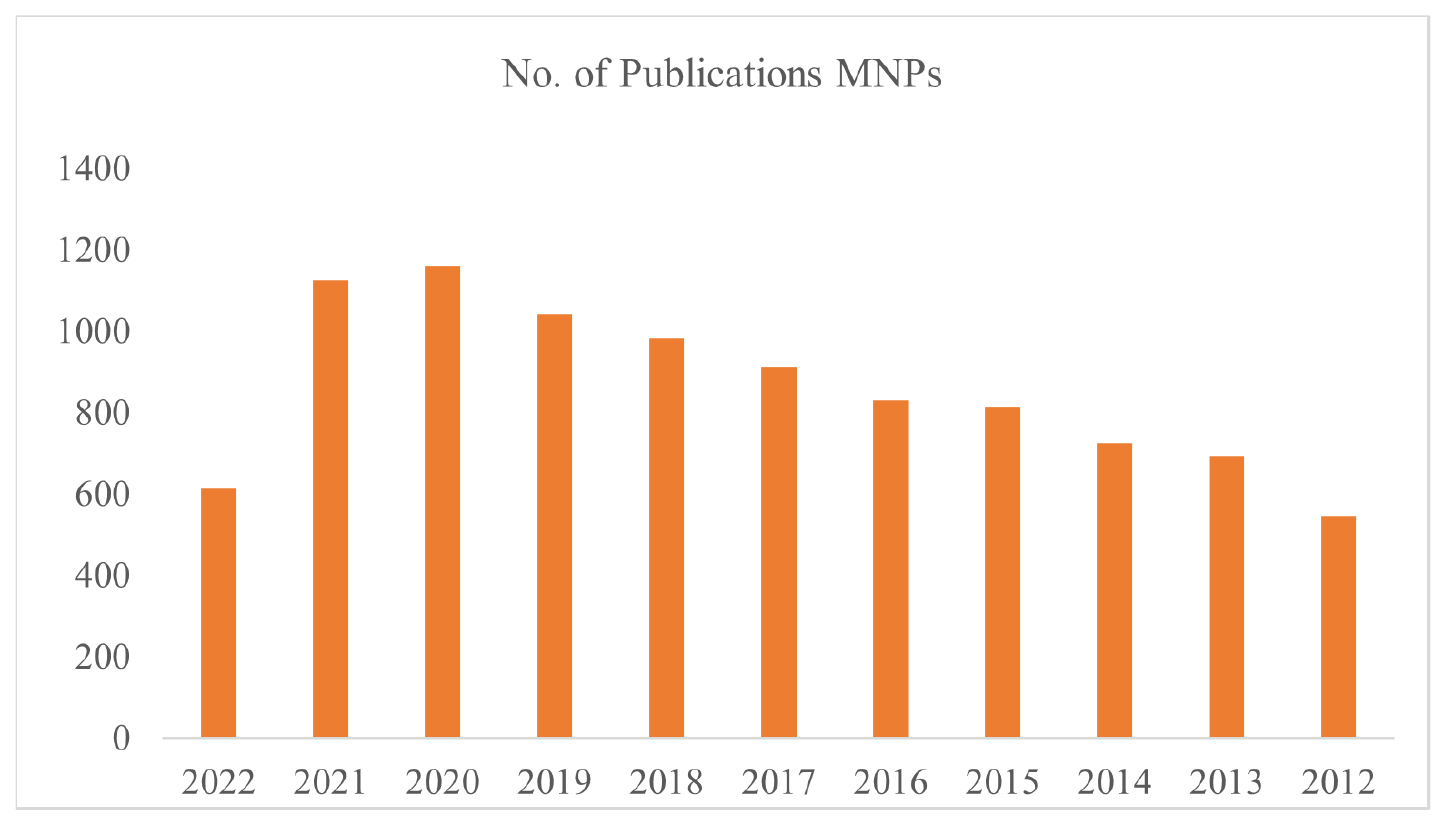
Figure 3:
Publications trend for MNPs from 2012 to till date.
FUTURE PROSPECTIVE AND CONCLUSION
MNPs as imaging contrast agents have a lot of potential for adjuvant therapy. The usage of MNPs has been subject to numerous restrictions, nevertheless, because of certain toxicity connected with them. MNPs’ surface coatings are known to affect both their potential toxicity and beneficial properties. According to research, the amount of MNPs that accumulate in vivo increases with MNP size. Controlling MNP size and surface coatings could thereby lessen toxicity and enhance magnetic properties. MNPs have been applied in a variety of methods for imaging, medication administration and magnetic hyperthermia to locate nonpalpable lesions. These fields are still developing quickly. However Recently, SLNB has made surgical use of MNPs to treat breast cancer and malignant melanoma. The purpose of this future preclinical study is to refine techniques of localizing nonpalpable breast lesions using ferromagnetic clip technology loaded with MNPs and portable magnetometers, so that the surgeon may more easily excise these lesions. Herceptin, targeted Photodynamic Therapy (PDT) and magnetic hyperthermia are still viable options for solid tumors such as breast cancer.?
ACKNOWLEDGMENT
Author Contributions: The final manuscript was prepared with the help of all contributors. Compliance with Ethical Standards.
ABBREVIATIONS
MNPs | Magnetic nanoparticles |
---|---|
SPION | Superparamagnetic iron oxide nanoparticles |
FMNPs | Fluorescent Magnetic Nanoparticles |
MPI | Magnetic Particle Imaging |
OA | Oleic acid |
polyethylene glycol | OA-PEG |
Tc99 | Technetium 99m |
SLNB | Sentinel lymph node biopsy |
PDT | Photodynamic therapy |
References
- Siegel R, Ward E, Brawley O, Jemal A. Cancer statistics, 2011: the impact of eliminating socioeconomic and racial disparities on premature cancer deaths. CA Cancer J Clin. 2011;61(4):212-36. [PubMed] | [CrossRef] | [Google Scholar]
- Carey L, Winer E, Viale G, Cameron D, Gianni L. Triple-negative breast cancer: disease entity or title of convenience?. Nat Rev Clin Oncol. 2010;7(12):683-92. [PubMed] | [CrossRef] | [Google Scholar]
- Carey LA. Triple-negative (basal-like) breast cancer: a new entity. Breast Cancer Res. 2007;9(Suppl 1):S13 [CrossRef] | [Google Scholar]
- McGurk R, Fallowfield L, Winters Z. Information provision for patients by breast cancer teams about the side-effects of hormone treatments. Eur J Cancer. 2006;42(12):1760-7. [PubMed] | [CrossRef] | [Google Scholar]
- Saber A, Hussien AG, Awad WA, Mahmoud A, Allakany A. Adapting the pre-trained convolutional neural networks to improve the anomaly detection and classification in mammographic images. Sci Rep. 2023;13(1):14877 [PubMed] | [CrossRef] | [Google Scholar]
- Adiraju RV, Elias S. A quantitative analysis of imaging features in lung CT images using the RW-T hybrid segmentation model. Multimedia Tool Appl. 2023;83(13):39479-502. [CrossRef] | [Google Scholar]
- 2024 Available from: http://www.stopbreastcancer.org
- Sjövall K, Strömbeck G, Löfgren A, Bendahl PO, Gunnars B. Adjuvant radiotherapy of women with breast cancer – information, support and side-effects. Eur J Oncol Nurs. 2010;14(2):147-53. [PubMed] | [CrossRef] | [Google Scholar]
- Moore S. Managing treatment side effects in advanced breast cancer. Semin Oncol Nurs. 2007;23(Array):S23-30. [PubMed] | [CrossRef] | [Google Scholar]
- Metzger Filho O, Saini KS, Azim HA, Awada A. Prevention and management of major side effects of targeted agents in breast cancer. Crit Rev Oncol Hematol. 2011;15(Suppl 1) [PubMed] | [CrossRef] | [Google Scholar]
- Tartaj P, Morales MP, Veintemillas-Verdaguer S, Gonzlez-Carreo T, Serna CJ. The preparation of magnetic nanoparticles for applications in biomedicine. J Phys D: Appl Phys. 2003;36(13):R182-97. [CrossRef] | [Google Scholar]
- Faraji M, Yamini Y, Rezaee M. Magnetic nanoparticles: synthesis, stabilization, functionalization, characterization and applications. J Iran Chem Soc. 2010;7(1):1-37. [CrossRef] | [Google Scholar]
- Niemeyer CM. Nanoparticles, Proteins, and Nucleic Acids: Biotechnology Meets Materials Science. Angew Chem Int Ed. 2001;40(22):4128-58. [CrossRef] | [Google Scholar]
- Davaran S, Entezami AA. Synthesis and hydrolysis of modified poly vinyl alcohols containing ibuprofen pendent groups. Iran Polym J. 1996;5(3):188-91. [CrossRef] | [Google Scholar]
- Akbarzadeh A, Samiei M, Davaran S. Magnetic nanoparticles: preparation, physical properties, and applications in biomedicine. Nanoscale Res Lett. 2012;7(1):144 [CrossRef] | [Google Scholar]
- Alexiou C, Jurgons R, Seliger C, Iro H. Medical applications of magnetic nanoparticles. J Nanosci Nanotechnol. 2006;6(9-10):2762-8. [PubMed] | [CrossRef] | [Google Scholar]
- Gillis P, Koenig SH. Transverse relaxation of solvent protons induced by magnetized spheres: application to ferritin, erythrocytes and magnetite. Magn Reson Med. 1987;5(4):323-45. [PubMed] | [CrossRef] | [Google Scholar]
- Martin D. Magnetism in solids. 1967 [PubMed] | [CrossRef] | [Google Scholar]
- Colombo M, Carregal-Romero S, Casula MF, Gutiérrez L, Morales MP, Böhm IB, et al. Biological applications of magnetic nanoparticles. Chem Soc Rev. 2012;41(11):4306-34. [PubMed] | [CrossRef] | [Google Scholar]
- Longmire M, Choyke PL, Kobayashi H. Clearance properties of nano-sized particles and molecules as imaging agents: considerations and caveats. Nanomedicine (Lond). 2008;3(5):703-17. [PubMed] | [CrossRef] | [Google Scholar]
- Maeda H. The enhanced permeability and retention (EPR) effect in tumor vasculature: the key role of tumor-selective macromolecular drug targeting. Adv Enzyme Regul. 2001;41:189-207. [PubMed] | [CrossRef] | [Google Scholar]
- Jain RK. Lessons from multidisciplinary translational trials on anti-angiogenic therapy of cancer. Nat Rev Cancer. 2008;8(4):309-16. [PubMed] | [CrossRef] | [Google Scholar]
- Ahmed M, Douek M. The role of magnetic nanoparticles in the localization and treatment of breast cancer. Biomed Res Int. 2013;2013:281230 [PubMed] | [CrossRef] | [Google Scholar]
- Gavrila H, Chiriac H, Ciureanu P, Ionita V, Yelon A. Editura Academiei. 2000 [PubMed] | [CrossRef] | [Google Scholar]
- Kolhatkar AG, Jamison AC, Litvinov D, Willson RC, Lee TR. Tuning the magnetic properties of nanoparticles. Int J Mol Sci. 2013;14(8):15977-6009. [PubMed] | [CrossRef] | [Google Scholar]
- Knobel M, Nunes WC, Socolovsky LM, Biasi E, Vargas JM, Denardin JC, et al. Super paramagnetism and other magnetic features in granular materials: a review on ideal and real systems. Nanosci Nanotechnol. 2008;8:2836-57. [PubMed] | [CrossRef] | [Google Scholar]
- Ferreira M, Sousa J, Pais A, Vitorino C. The role of magnetic nanoparticles in cancer Nanotheranostics. Materials (Basel). 2020;13(2):266 [PubMed] | [CrossRef] | [Google Scholar]
- Manescu Paltanea V, Paltanea G, Antoniac I, Vasilescu M. Magnetic nanoparticles used in oncology. Materials (Basel). 2021;14(20):5948 [PubMed] | [CrossRef] | [Google Scholar]
- Karelia D, Pandey MK, Irby R, Amin S, Sharma AK. Suppression of pancreatic cancer cell growth by NISC6 through activation of Par4 and death receptor 5. Exp. Mol Ther. 2013(73) Abstract 3439: [PubMed] | [CrossRef] | [Google Scholar]
- Wang Z, Tong M, Chen X, Hu S, Yang Z, Zhang Y, et al. Survivintargeted nanoparticles for pancreatic tumor imaging in mouse model. Nanomedicine. 2016;12(6):1651-61. [PubMed] | [CrossRef] | [Google Scholar]
- Bucci OM, Crocco L, Scapaticci R. On the optimal measurement configuration for magnetic nanoparticlesenhanced breast cancer microwave imaging. IEEE Trans Bio Med Eng. 2015;62(2):407-14. [PubMed] | [CrossRef] | [Google Scholar]
- Kato Y, Zhu W, Backer MV, Neoh CC, Hapuarachchige S, Sarkar SK, et al. Noninvasive imaging of liposomal delivery of superparamagnetic iron oxide nanoparticles to orthotopic human breast tumor in mice. Pharm Res. 2015;32(11):3746-55. [PubMed] | [CrossRef] | [Google Scholar]
- Sun J, Teng ZG, Tian Y, Wang JD, Guo Y, Kim DH, et al. Targeted fluorescent magnetic nanoparticles for imaging of human breast cancer. Int J Clin Exp Med. 2014;7(12):4747-58. [PubMed] | [Google Scholar]
- Bevacqua MT, Scapaticci R. A Compressive sensing approach for 3D breast cancer microwave imaging with magnetic nanoparticles as contrast agent. IEEE Trans Med Imaging. 2016;35(2):665-73. [PubMed] | [CrossRef] | [Google Scholar]
- Guneyli S, Erdem CZ, Erdem LO. Magnetic resonance imaging of prostate cancer. Clin Imaging. 2016;40(4):601-9. [PubMed] | [CrossRef] | [Google Scholar]
- Winter A, Kowald T, Paulo T, Goos P, Engels S, Gerullis H, et al. 1060 Magnetic resonance sentinel lymph node imaging in prostate cancer using intraprostatic injection of superparamagnetic iron oxide nanoparticles: the first inhuman results. Eur Urol Suppl. 2016;15(3):e1060 [CrossRef] | [Google Scholar]
- Winter A, Woenkhaus J, Wawroschek F. 979 Magnetic marking and intraoperative detection of primary draining lymph nodes in prostate cancer using intraprostatic injection of superparamagnetic iron oxid nanoparticles. Eur Urol Suppl. 2015;14(2):e979 [CrossRef] | [Google Scholar]
- Hill DK, Kim E, Teruel JR, Jamin Y, Widerøe M, Søgaard CD, et al. Diffusionweighted MRI for early detection and character-ization of prostate cancer in the transgenic adenocarcinoma of the mouse prostate model. J Magn Reson Imaging. 2016;43(5):1207-17. [PubMed] | [CrossRef] | [Google Scholar]
- Wan X, Song Y, Song N, Li J, Yang L, Li Y, et al. The preliminary study of immune superparamagnetic iron oxide nanoparticles for the detection of lung cancer in magnetic resonance imaging. Carbohydr Res. 2016;419:33-40. [PubMed] | [CrossRef] | [Google Scholar]
- Stocke NA, Meenach SA, Arnold SM, Mansour HM, Hilt JZ. Formulation and characterization of inhalable magnetic nanocomposite Microparticles (MnMs) for targeted pulmonary delivery via spray drying. Int J Pharm. 2015;479(2):320-8. [PubMed] | [CrossRef] | [Google Scholar]
- Nishimoto K, Mimura A, Aoki M, Banura N, Murase K. Application of magnetic particle imaging to pulmonary imaging using nebulized magnetic nanoparticles. Open J Med Imag. 2015;05(2):49-55. [CrossRef] | [Google Scholar]
- Jain TK, Morales MA, Sahoo SK, Leslie-Pelecky DL, Labhasetwar V. Vinod Labhasetwar Iron oxide nanoparticles for sustained delivery of anticancer agents. Mol Pharm. 2005;2(3):194-205. [PubMed] | [CrossRef] | [Google Scholar]
- Kohler N, Sun C, Wang J, Zhang M. Methotrexate modified superparamagnetic nanoparticles and their intracellular uptake into human cancer cells. Langmuir. 2005;21(19):8858-64. [PubMed] | [CrossRef] | [Google Scholar]
- Munnier E, Cohen-Jonathan S, Linassier C, Douziech-Eyrolles L, Marchais H, Soucé M, et al. Novel method of doxorubicin-SPION reversible association for magnetic drug targeting. Int J Pharm. 2008;363(1-2):170-76. [PubMed] | [CrossRef] | [Google Scholar]
- Benyettou F, Lalatonne Y, Sainte-Catherine O, Monteil M, Motte L. Superparamagnetic nanovector with anticancer properties: ?Fe2O3@Zoledronate. I nt J Pharm. 2009;379(2):324-27. [PubMed] | [CrossRef] | [Google Scholar]
- Yallapu MM, Foy SP, Jain TK, Labhasetwar V. PEG-functionalized magnetic nanoparticles for drug delivery and magnetic resonance imaging applications. Pharm Res. 2010;27(11):2283-95. [PubMed] | [CrossRef] | [Google Scholar]
- Hosu O, Tertis M, Cristea C. Implication of magnetic nanoparticles in cancer detection, screening and treatment. Magnetochemistry. 2019;5(4):55 [CrossRef] | [Google Scholar]
- Chen Z, Wu C, Zhang Z, Wu W, Wang X, Yu Z, et al. Synthesis, functionalization and nanomedical applications of functional magnetic nanoparticles. Chin Chem Lett. 2018;29(11):1601-8. [CrossRef] | [Google Scholar]
- Cristea C, Tertis M, Galatus R. Magnetic nanoparticles for antibiotics detection. Nanomaterials. 2017;7(6):119 [CrossRef] | [Google Scholar]
- Ebadi M, Bullo S, Buskaran K, Hussein MZ, Fakurazi S, Pastorin G, et al. Dual-Functional Iron Oxide nanoparticles coated with polyvinyl Alcohol/5-Fluorouracil/ Zinc-aluminium-layered double hydroxide for a simultaneous drug and target delivery system. Polymers. 2021;13(6):855 [PubMed] | [CrossRef] | [Google Scholar]
- Hayashi K, Tokuda A, Nakamura J, Sugawara-Narutaki A, Ohtsuki C. Tearable and fillable composite sponges capable of heat generation and drug release in response to alternating magnetic field. Materials (Basel). 2020;13(16):3637 [PubMed] | [CrossRef] | [Google Scholar]
- Rao GK, Gowthami B, Naveen NR, Samudrala PK. An updated review on potential therapeutic drug candidates, vaccines and an insight on patents filed for COVID-19. Curr Res Pharmacol Drug Discov. 2021;1(2):100063 [PubMed] | [CrossRef] | [Google Scholar]
- Islam MM, Naveen NR, Anitha P, Goudanavar PS, Rao GS, Fattepur S, et al. The race to replace PDE5i: recent advances and interventions to treat or manage erectile dysfunction: evidence from patent landscape (2016-2021). J Clin Med. 2022;11(11):3140 [PubMed] | [CrossRef] | [Google Scholar]