ABSTRACT
A potential development in medication administration, transferosomes are lipid-based vesicles that have been designed with edge activators, especially for transdermal and transmucosal uses. Transferosomes, which are mostly made of phospholipids enhanced with surfactants, are remarkably flexible and facilitate the effective passage of both hydrophilic and lipophilic medicines across biological barriers. Because of their deformability, drugs can more easily pass through microscopic pores and intercellular gaps. A wide variety of pharmaceuticals may be encapsulated in transferosomes, which have benefits include prolonged release patterns, defence against enzymatic degradation, and the ability to encapsulate both lipophilic and hydrophilic compounds at the same time. They are useful for systemic and topical drug administration due to their capacity to evade hepatic first-pass metabolism; they may also find use in targeted treatment and extended-release formulations. Despite their benefits, transferosomes have drawbacks, such as the high expense of Chemical instability and natural phospholipids. Exact control over vesicle properties is possible by a variety of production techniques, including as centrifugation, sonication, and thin film hydration. size analysis, entrapment efficiency, deformability, and stability assessment are all included in characterization approaches. Transferosomes are useful for delivering tiny molecules, proteins, and peptides. They can also be used to improve oral bioavailability, improve topical medication administration, and perhaps transform vaccine delivery and ocular applications.
INTRODUCTION
As a means of delivering drugs, lipid-based vesicles or carriers known as transferosomes have been created.1 Like liposomes, transferosomes are composed of phospholipids, but with the addition of edge activators that increase the deformability and flexibility of the vesicle. Because of this special property, transferosomes are especially useful for transdermal and transmucosal drug delivery.2 Transferosomes major goal is to overcome the drawbacks of conventional drug delivery penetration.3 By driving them through tiny pores and intercellular spaces and increasing their ability to deliver drugs past biological barriers, transferosomes can drastically deform when edge activators are added.4 Typically, surfactants or penetration enhancers like sodium cholate, tween 80, or span 80 are used as edge activators in transferosomes.5 These edge activators break up the vesicle’s lipid bilayers, lowering interfacial tension and boosting lipid membrane fluidity.6 Due to their great deformability and elasticity, transferosomes can encapsulate a variety of medicines, including hydrophilic, lipophilic, and macromolecular substances.7 Due to their bilayer shape, transferosomes have the unique capacity to concurrently encapsulate both hydrophilic and lipophilic medicines. They are versatile carriers for a range of medicinal drugs because of this characteristic. Transferosomes can also enhance the stability of the encapsulated medicines, prevent degradation, and create a sustained release profile.8
One of the main uses for transferosomes is the administration of medications via the skin. By bypassing the digestive tract and avoiding first-pass metabolism, they can effectively carry medicines past the skin barriers and into the systemic circulation. This method of administration has a number of advantages, such as extended drug release, fewer side effects, better patient compliance and the potential for localised drug delivery to particular areas.9
ADVANTAGES
Given their architecture, which combines hydrophobic and hydrophilic elements10 transferosomes may hold drugs with different solubilities and can bend and pass through small openings with little loss, therefore enclosing them.11
They protect the medication inside the capsule from enzymatic and metabolic deterioration.12
They can be used to deliver medications systemically and topically.13
They can serve as depot formulations to gradually release the medication they contain.14
This system’s great deformability makes it possible for intact vesicles to penetrate more effectively. Both low and high-molecular-weight medications, such as insulin, albumin, sex hormones, corticosteroids, analgesics, and anaesthetics, can be transported by them.15
They are similar to liposomes in that they are formed of natural phospholipids, making them biocompatible and biodegradable.16
When it comes to lipophilic medications, their entrapment efficiency is about 90%.17
Because they prevent needless usage or ingredients that are undesirable for use in pharmaceuticals, they are simple to scale up.18
It boosts bioavailability by bypassing hepatic first-pass metabolism. 19
It has a long shelf life and can be used for medications with a short half-life, a small therapeutic window, and poor oral absorption.20
LIMITATIONS
Transferosomes cannot be widely used as drug delivery vehicles because of the high expense of formulations resulting from the natural phospholipids’ purity requirements.21
The oxidative damage-prone chemical instability of transferosomes can be greatly reduced by degassing and removing inert gases such as nitrogen and argon from the aqueous media.
The difficulty of obtaining pure natural phospholipids is another barrier to using transferosomes as a drug delivery mechanism. As a result, synthesised phospholipids may be employed as substitutes.22
Transferosome formulation costs are largely determined by the cost of lipid excipients and production equipment. Phosphatidylcholine is the most commonly used lipid component because of its low cost.23
MECHANISM OF ACTION
Made of amphiphilic molecules arranged in a bilayer configuration, vesicles are a flexible drug delivery system that can hold both hydrophilic and hydrophobic drugs.24 Transferosomes are a promising method for targeted drug delivery across biological barriers because of their exceptional deformability and self-optimizing nature.25 They also work well at bridging the epidermal barrier. By disrupting intercellular lipids in the stratum corneum, they get past the skin’s internal sealing lipids and may improve medication delivery.26 After penetration, transferosomes serve as complete drug delivery systems, therefore the carrier must proliferate and elude hydrophilic skin pores.27 Similar to traditional endocytosis, intracellular drug administration may employ the diffusion of vesicle-lipid bilayers with cell membranes, utilising contemporary mechanical and osmotic force ideas.28
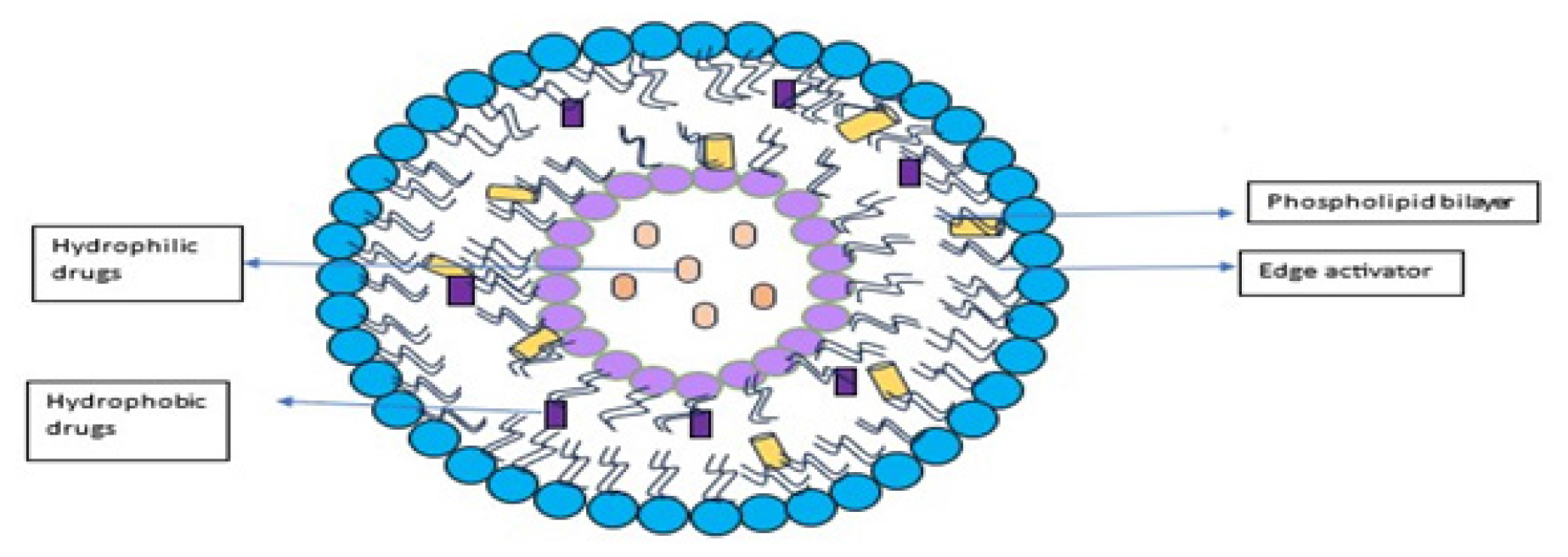
Figure 1:
Structure of transferosomes.
Penetrants may be able to pass through the epidermal barrier via one of the following routes: across the intact horny layer, through sweat glands, hair follicles, and sebaceous glands that are linked.29
STRUCTURE AND COMPOSITION
Structure
Phospholipids
Phospholipids are the principal constituents of the lipid bilayer. They are essential for the creation of vesicles and the preservation of structural integrity in transferosomes because they have amphiphilic characteristics, with hydrophilic heads and hydrophobic tails.31
Surfactants
Transferosome formulations frequently contain surfactants or edge activators to improve the lipid bilayer’s elasticity and flexibility. They enhance vesicle deformability by lowering cohesive forces between lipid molecules.32
Composition
In an aqueous media, phospholipids self-assemble into pliable lipid bilayers to produce vesicles that can be destabilised by edge activators or single-chain surfactants to increase fluidity and elasticity.33 Surfactant concentration and ratios in relation to phospholipids control the flexibility of vesicle membranes, lowering the possibility of vesicle rupture during skin application and permitting nonocclusive adhesion to the epidermal osmotic gradient. Variations in lipid types, vesicle properties, and surfactant composition have a significant impact on how well transferosomes enhance penetration. The components are mentioned in Table 1.
Components | Example | Uses |
---|---|---|
Phospholipids | Soya phosphatidyl choline, egg phosphatidyl choline, and dipalmitoyl phosphatidyl choline. | Vesicles forming complexes. |
Surfactants | Sodium cholate, sodium deoxycholate, Tween-80, and span-80. | For providing flexibility. |
Alcohol | Ethanol, methanol. | As a solvent. |
Buffering agent | Saline phosphate buffer (pH 6.4). | As a hydrating medium. |
Dye | Rhodamine 123, Nile red. | For Confocal Scanning Laser Microscopy (CSLM).34 |
Method of Preparations
Thin film hydration technique
The three-step thin film hydration process forms transferosomes. First, a thin film is created by mixing phospholipids, surfactant, and vesicle components in chloroform methanol; second, the solvent is removed using a rotary evaporator at a temperature above the lipid transition temperature35 and third, the thin film is hydrated with pH 6.5 buffer, spun, and expanded at room temperature. To achieve the desired sizes, the resultant vesicles are homogenised and manually extruded through polycarbonate membranes after undergoing freezing, sonication, or both.36
Sonication and vortexing
Critical techniques in transferosome-lipid-based vesicle synthesis, sonication and vortexing, use high-frequency sound waves and mechanical agitation, respectively, to generate micro-scale disruptions that support vesicle formation, improve stability for drug transport, and generate flexible transferosomes for efficient drug delivery through biological barriers such as the stratum corneum of the skin.
Modified handshaking method
Both the modified handshaking method and the rotary evaporation sonication method work on similar principles: an organic solvent, phospholipids, lipophilic medication, and an edge activator are dissolved to form a transparent solution in a round-bottom flask. The solvent is then removed by handshaking, and the solution is hydrated with a suitable buffer solution to form a film.37
Suspension homogenation method
Lipid-based vesicles called transferosomes are designed to improve the delivery of medication because of their remarkable deformability and drug-carrying capacity. To do this, lipid components are dispersed in an aqueous phase to generate a lipid suspension. Next, lipid aggregates are sized down via high-speed homogenization, which forms tiny vesicles. This method is beneficial for pharmaceutical and biological applications, especially in production scaling and maintaining consistent vesicle quality, since it provides exact control over vesicle size, ensuring homogeneity and stability.38?
Reverse-phase evaporation method
Lipid-based vesicles called transferosomes are employed in drug delivery systems. When lipids are dissolved in an organic solvent to form an organic phase, they are produced. The medicinal ingredient is added together with an aqueous phase to create a Water-in-Oil (W/O) emulsion. The organic solvent evaporates during the emulsification process, producing concentrated transferosomes that may include hydrophilic and hydrophobic medications inside the vesicles. This versatile technique can be applied to a variety of drug delivery circumstances, including transdermal distribution and targeted therapy.
Ethanol injection method
Transferosomes stabilised by ethanol are formed when a lipid solution dissolved in ethanol is delivered into an aqueous phase containing the drug, causing fast mixing. Medications that are susceptible to organic solvents are best suited for this approach. To form the organic and aqueous phases, which are both heated to 45–50°C, phospholipid, edge activator, and lipophilic drug are dissolved separately in ethanol, while water-soluble components are dissolved in phosphate buffer.
Centrifugation process
After dissolving the medication, phospholipids, and surfactants in alcohol, the solvent is extracted using rotary evaporation at low temperatures and low pressure. After that, hoover is used to get rid of any solvent residue. After obtaining the lipid layer, it is hydrated with the suitable buffer by centrifugation at 60 rpm for an hour at room temperature. This is followed by vesicle inflating for 2 hr and another room-temperature sonication cycle.
Freeze the process
After dissolving lipids and surfactants in an organic solvent, lipid bilayers are broken up by repeatedly freezing and thawing the mixture, which creates tiny multilamellar vesicles that have improved drug encapsulation properties.
High-pressure homogenization technique
A comprehensive and effective technique for generating transferosomes is high-pressure homogenization, which involves passing a lipid mixture including surfactants and phospholipids through a narrow-gap homogenization device several times. Lipid bilayers are broken apart by this mechanical shearing, resulting in uniformly sized, tiny transferosomes.39
CHARACTERIZATION
Vesicle size, zeta potential and morphology
Vesicle size determination is crucial for the synthesis of transferosomes, batch-to-batch comparison, and scale-up processes. The physical stability of the formulation is impacted by changes in vesicle size throughout storage. Vesicles smaller than 40 nm are more likely to proceed through fusion processes because of their high curvature, whereas larger transferosomes are pulled together by van der Waals interactions. Techniques like Photon Correlation Spectroscopy (PCS) and Dynamic Light Scattering (DLS) are used to quantify vesicle size. Zeta potential influences stability and may be measured with the use of electrophoretic mobility. Transferosome vesicles can be seen with phase contrast microscopy or Transmission Electron Microscopy (TEM).40
Transmission Electron Microscopy (TEM)
To view the morphology of transferosomes, TEM is used. Through TEM visualisation of the vesicles, scientists may evaluate their integrity, form, and structural attributes. With this method, the internal composition and surface morphology of transferosomes may be thoroughly examined, yielding important data for formulation optimisation and quality assurance.
Entrapment Efficiency Determination
An indirect method utilising the centrifuge technique is used to measure entrapment efficiency. Using an analysis of the released drug in the supernatant, this technique calculates the quantity of drug entrapped within transferosomes. Assessing the entrapment efficiency of transferosomes is essential for determining their drug-loading capacity and maximising the effectiveness of their drugdelivery.41
Zeta potential measurement
The electrophoretic mobility technique is used to detect zeta potential using devices such as the Malvern Zetasizer. This quantity represents the transferosomes’ surface charge, which affects their interactions and stability. Zeta potential tests shed light on transferosomes’ electrostatic characteristics, which helps with formulation optimisation and performance assessment.42
Stability Studies
In stability investigations, formulation integrity and performance consistency are ensured by monitoring changes in transferosomes over time. Researchers may assess transferosomes physical and chemical stability which is essential for preserving product quality and efficacy by keeping them under particular circumstances and examining their features after a predetermined amount of time.43
APPLICATIONS
Transferosomes are incredibly flexible for delivering a variety of therapeutic materials, such as proteins, peptides, and small molecules, and are particularly effective at encapsulating both hydrophilic and lipophilic medicines.44
Transferosomes improve the delivery of topical drugs by penetrating the stratum corneum, the skin’s topmost layer. This is especially useful for delivering drugs that are difficult to cross the epidermal barrier, such as corticosteroids and some antibacterial agents.45
For drugs that require extended-release profiles or have restricted bioavailability, transdermal patches containing transferosomes provide a handy and non-invasive way for regulated and sustained drug release through the skin. 46
Enclosing poorly water-soluble pharmaceuticals within lipid bilayers, transferosomes improve treatment outcomes by overcoming low solubility difficulties and increasing the oral bioavailability of particular medications.47
Transferosomes have the ability to increase antigen uptake by antigen-presenting cells, which has important ramifications for vaccine delivery and makes them an essential tool in vaccine development since they may stimulate a more robust immune response.48
Transferosomes have the potential to optimise drug delivery to the eye and boost therapeutic efficacy in ophthalmic applications such as eye drops or gels.49
CONCLUSION
Transferosomes, which have better penetration, deformability, and versatility in encasing different molecules, represent a substantial development in transdermal drug delivery. Flexibility, integrity, and stability are dependent on the critical component of the formulation in particular, the ratio of the edge activator to phospholipids. When this field of study develops, transferosomes, with their capacity to carry a variety of chemicals, hold great promise for improving the viability and efficacy of transdermal drug delivery.
Cite this article:
Haranath C. Exploring Transferosomes: A Comprehensive Review of Novel Strategies and Applications in Drug Delivery. J Young Pharm. 2024;16(3):410-5.
ACKNOWLEDGEMENT
Authors are thankful to the RIPER for providing support
ABBREVIATIONS
CSLM | Confocal scanning laser microscopy |
---|---|
DLS | Dynamic light scattering |
PCS | Photon correlation spectroscopy |
TEM | Transmission electron microscopy |
References
- Chaurasiya P, Ganju E, Upmanyu N, Ray SK, Jain P. Transferosomes: a novel technique for transdermal drug delivery. J Drug Deliv Ther. 2019;9(1):279-85. [CrossRef] | [Google Scholar]
- Opatha SA, Titapiwatanakun V, Chutoprapat R. Transferosomes: A promising nanoencapsulation technique for transdermal drug delivery. Pharmaceutics. 2020;12(9):855 [PubMed] | [CrossRef] | [Google Scholar]
- Jeong WY, Kwon M, Choi HE, Kim KS. Recent advances in transdermal drug delivery systems: a review. Biomater Res. 2021;25:1 [PubMed] | [CrossRef] | [Google Scholar]
- Sudhakar K, Fuloria S, Subramaniyan V, Sathasivam KV, Azad AK, Swain SS, et al. Ultraflexible liposome nanocargo as a dermal and transdermal drug delivery system. Nanomaterials (Basel). 2021;11(10):2557 [PubMed] | [CrossRef] | [Google Scholar]
- Fernández-García R, Lalatsa A, Statts L, Bolás-Fernández F, Ballesteros MP, Serrano DR, et al. Transferosomes as nanocarriers for drugs across the skin: quality by design from lab to industrial scale. Int J Pharm. 2020;573:118817 [PubMed] | [CrossRef] | [Google Scholar]
- Kotouček J, Hubatka F, Mašek J, Kulich P, Velínská K, Bezdeková J, et al. Preparation of nanoliposomes by microfluidic mixing in herring-bone channel and the role of membrane fluidity in liposomes formation. Sci Rep. 2020;10(1):5595 [PubMed] | [CrossRef] | [Google Scholar]
- Akram MW, Jamshaid H, Rehman FU, Zaeem M, Khan Jz, Zeb A, et al. Transferosomes revolutionary nanosystem for efficient transdermal drug delivery. AAPS PharmSciTech. 2022;23:1-18. [PubMed] | [CrossRef] | [Google Scholar]
- Maji R, Omolo CA, Jaglal Y, Singh S, Devnarain N, Mocktar C, et al. A transferosome-loaded bigel for enhanced transdermal delivery and antibacterial activity of vancomycin hydrochloride. Int J Pharm. 2021;607:120990 [PubMed] | [CrossRef] | [Google Scholar]
- Antimisiaris SG, Marazioti A, Kannavou M, Natsaridis E, Gkartziou F, Kogkos G, et al. Overcoming barriers by local drug delivery with liposomes. Adv Drug Deliv Rev. 2021;174:53-86. [PubMed] | [CrossRef] | [Google Scholar]
- Myneni GS, Radha G, Soujanya G. Novel vesicular drug delivery systems: a review. J Pharm Res. 2021;11:1650-64. [PubMed] | [CrossRef] | [Google Scholar]
- Gupta R, Kumar A. Transfersomes: the ultra-deformable carrier system for non-invasive delivery of drug. Curr Drug Deliv. 2021;18(4):408-20. [PubMed] | [CrossRef] | [Google Scholar]
- Verma S, Goand UK, Husain A, Katekar RA, Garg R, Gayen JR, et al. Challenges of peptide and protein drug delivery by oral route: current strategies to improve the bioavailability. Drug Dev Res. 2021;82(7):927-44. [PubMed] | [CrossRef] | [Google Scholar]
- Patil P, Datir S, Saudagar R. A review on topical gels as drug delivery system. J Drug Deliv Ther. 2019;9(3):989-94. [PubMed] | [CrossRef] | [Google Scholar]
- Kanwar N, Sinha VR. In situ forming depot as sustained-release drug delivery systems. Crit Rev Ther Drug Carrier Syst. 2019;36(2):93-136. [PubMed] | [CrossRef] | [Google Scholar]
- Tiwari G, Tiwari R, Singh R, Rai AK. Ultra-deformable liposomes as flexible nanovesicular carrier to penetrate versatile drugs transdermally. NANOASIA. 2020;10(1):12-20. [CrossRef] | [Google Scholar]
- Dymek M, Sikora E. Liposomes as biocompatible and smart delivery systems–The current state. Adv Colloid Interface Sci. 2022;309:102757 [PubMed] | [CrossRef] | [Google Scholar]
- Peng S, Zou L, Zhou W, Liu W, Liu C, McClements DJ, et al. Encapsulation of lipophilic polyphenols into nanoliposomes using pH-driven method: advantages and disadvantages. J Agric Food Chem. 2019;67(26):7506-11. [PubMed] | [CrossRef] | [Google Scholar]
- Brayden DJ, Hill TA, Fairlie DP, Maher S, Mrsny RJ. Systemic delivery of peptides by the oral route: formulation and medicinal chemistry approaches. Adv Drug Deliv Rev. 2020;157:2-36. [PubMed] | [CrossRef] | [Google Scholar]
- Agrawal MB, Patel MM. Optimization and evaluation of quetiapine-loaded transdermal drug delivery system for the treatment of schizophrenia. Drug Dev Ind Pharm. 2020;46(11):1819-31. [PubMed] | [CrossRef] | [Google Scholar]
- Dan N, Samanta K, Almoazen H. An update on pharmaceutical strategies for oral delivery of therapeutic peptides and proteins in adults and pediatrics. Children (Basel). 2020;7(12):307 [PubMed] | [CrossRef] | [Google Scholar]
- Parmar J, Chaudhary Z, Upadhyay S, Upadhyay U. Dosage forms in novel drug delivery system. National Journal of Pharmaceutical Sciences. 2022;2(2):177-90. [PubMed] | [CrossRef] | [Google Scholar]
- Mirtaleb MS, Shahraky MK, Ekrami E, Mirtaleb A. Advances in biological nano phospholipid vesicles for transdermal delivery: a review on applications. J Drug Deliv Sci Technol. 2021;61:102331 [CrossRef] | [Google Scholar]
- Lokhande S, Jadhao M. Transferosomes: A Novel Approach to Drug Delivery. International Journal in Pharmaceutical Sciences. 2023;1(9):279-87. [CrossRef] | [Google Scholar]
- Apolinário AC, Hauschke L, Nunes JR, Lopes LB. Lipid nanovesicles for biomedical applications: ’What is in a name’?. Prog Lipid Res. 2021;82:101096 [PubMed] | [CrossRef] | [Google Scholar]
- Farooque F, Wasi M, Mughees MM. Liposomes as drug delivery system: an updated review. J Drug Deliv Ther. 2021;11(5-S):149-58. [CrossRef] | [Google Scholar]
- Siva Rama Krishnan S. Transdermal delivery of febuxostat using elastic liposomes formulation and characterisation. 2019 [CrossRef] | [Google Scholar]
- Phatale V, Vaiphei KK, Jha S, Patil D, Agrawal M, Alexander A, et al. Overcoming skin barriers through advanced transdermal drug delivery approaches. J Control Release. 2022;351:361-80. [PubMed] | [CrossRef] | [Google Scholar]
- Haranath C, Poojitha N, Ahad HA, Yarra S, Eranti B. Recent advances in lipid based nanovesicles for transdermal drug delivery. J Med Pharm Allied Sci. 2022;11(6):5217-20. [PubMed] | [CrossRef] | [Google Scholar]
- Woo WM. Skin structure and biology. Imaging Technol Transdermal Deliv Skin Disord. 2019;2(3):1-14. [PubMed] | [CrossRef] | [Google Scholar]
- Sapkota R, Dash AK. Liposomes and transferosomes: A breakthrough in topical and transdermal delivery. Ther Deliv. 2021;12(2):145-58. [PubMed] | [CrossRef] | [Google Scholar]
- Van Tran V, Moon JY, Lee YC. Liposomes for delivery of antioxidants in cosmeceuticals: challenges and development strategies. J Control Release. 2019;300:114-40. [PubMed] | [CrossRef] | [Google Scholar]
- Sharma U, Verma P, Jain NK. A review on novel vesicular drug delivery system: transferosomes. Int J Pharm Life Sci. 2020;11(7):321-8. [PubMed] | [CrossRef] | [Google Scholar]
- Chen S, Hanning S, Falconer J, Locke M, Wen J. Recent advances in non-ionic surfactant vesicles (niosomes): fabrication, characterization, pharmaceutical and cosmetic applications. Eur J Pharm Biopharm. 2019;144:18-39. [PubMed] | [CrossRef] | [Google Scholar]
- Nayak D, Tippavajhala VK. A comprehensive review on preparation, evaluation and applications of deformable liposomes. Iran J Pharm Res. 2021;20(1):186-205. [PubMed] | [CrossRef] | [Google Scholar]
- Kauslya A, Borawake PD, Shinde JV, Chavan RS. Niosomes: a novel carrier drug delivery system. J Drug Deliv Ther. 2021;11(1):162-70. [CrossRef] | [Google Scholar]
- Kodi SR, Reddy MS. Transferosomes: A novel topical approach. J Drug Deliv Ther. 2023;13(2):126-31. [CrossRef] | [Google Scholar]
- Verma A, Jain A, Tiwari A, Saraf S, Panda PK, Agrawal GP, et al. Folate conjugated double liposomes bearing prednisolone and methotrexate for targeting rheumatoid arthritis. Pharm Res. 2019;36(8):123 [PubMed] | [CrossRef] | [Google Scholar]
- Chinthaginjala H, Abdul H, Reddy AP, Kodi K, Manchikanti SP, Pasam D, et al. Nanosuspension as promising and potential drug delivery: a review. Int J Life Sci Pharm Res. 2020;11(1):59-66. [CrossRef] | [Google Scholar]
- Sahu AN, Mohapatra D. Nanovesicular transferosomes for the topical delivery of plant bioactives. Nanomedicine (Lond). 2021;16(28):2491-5. [PubMed] | [CrossRef] | [Google Scholar]
- Gayathri H, Sangeetha S. Design and development of tofacitinib citrate loaded transferosomal gel for skin cancer by box-Behnken design-doe approach. Int J Health Sci. 2022;6:3119-40. [CrossRef] | [Google Scholar]
- Poddar S, Sharmeen S, Hage DS. Entrapment of proteins within columns for high performance Affinity Chromatography. Affinity Chromatography: methods and protocols. 2022:205-27. [CrossRef] | [Google Scholar]
- Joseph TM, Luke PM. Transferosomes: novel delivery system for increasing Theskin permeation of drugs. Int J Med Pharm Sci. 2020;10(2):1-8. [CrossRef] | [Google Scholar]
- Elmowafy M. Skin penetration/permeation success determinants of nanocarriers: pursuit of a perfect formulation. Colloids Surf B Biointerfaces. 2021;203:111748 [PubMed] | [CrossRef] | [Google Scholar]
- Chacko IA, Ghate VM, Dsouza L, Lewis SA. Lipid vesicles: A versatile drug delivery platform for dermal and transdermal applications. Colloids Surf B Biointerfaces. 2020;195:111262 [PubMed] | [CrossRef] | [Google Scholar]
- Souto EB, Dias-Ferreira J, Oliveira J, Sanchez-Lopez E, Lopez-Machado A, Espina M, et al. Trends in atopic dermatitis-from standard pharmacotherapy to novel drug delivery systems. Int J Mol Sci. 2019;20(22):5653-9. [PubMed] | [CrossRef] | [Google Scholar]
- Pardeshi SR, More MP, Kulkarni AD, Pardeshi CV, Patil PB, Patil AS, et al. Current perspectives in nanomedicine delivery for targeted ocular therapeutics. Bull Mater Sci. 2023;46(1):35-9. [CrossRef] | [Google Scholar]
- Anita C, Munira M, Mural Q, Shaily L. Topical nanocarriers for management of rheumatoid arthritis: a review. Biomed Pharmacother. 2021;141:111880 [PubMed] | [CrossRef] | [Google Scholar]
- Karunakaran B, Gupta R, Patel P, Salave S, Sharma A, Desai D, et al. Emerging trends in lipid-based vaccine delivery: A special focus on developmental strategies, fabrication methods, and applications. Vaccines. 2023;11(3):661-7. [PubMed] | [CrossRef] | [Google Scholar]
- Witika BA, Mweetwa LL, Tshiamo KO, Edler K, Matafwali SK, Ntemi PV, et al. Vesicular drug delivery for the treatment of topical disorders: current and future perspectives. J Pharm Pharmacol. 2021;73(11):1427-41. [PubMed] | [CrossRef] | [Google Scholar]