ABSTRACT
Cannabis sativa, commonly known as marijuana, has been historically used for medicinal and recreational purposes. It has been employed in the treatment of neurological conditions. Cannabidiol (CBD), the active component of cannabis, has shown therapeutic effects and has been studied extensively for its potential benefits in various neurological disorders using preclinical models. The objective of this review is to consolidate current research on CBD and its association with Apo-lipoprotein (ApoE) and other targets related to neurodegenerative diseases. A comprehensive search of the PubMed Scopus and google scholar databases was conducted using keywords such as CBD, Microglia activation, astrocytes, ApoE, mammalian target of Rapamycin and wingless-related integration site expression. The available evidence suggests that CBD does not significantly affect the endocannabinoid system, except in vitro at high concentrations, thereby generating considerable interest in its therapeutic potential. However, the current physiological targets for CBD are challenging to exploit for neurological treatment, leading to uncertain clinical findings. In certain cases, there is minimal or no correlation between the disease and the identified targets. This review examines the classic receptors, neurotransmitters and pathways associated with both ApoE and CBD. Additionally, several interconnected targets of CBD have been discovered that exhibit a relationship with a specific ApoE, rather than merely triggering its action. Various molecular targets of CBD have been identified for specific neurodegenerative diseases, playing a central role in the ApoE system.
INTRODUCTION
Cannabis, a plant indigenous to the tropical and subtropical regions of India and naturally occurring in the Himalayan mountain area, has a rich history of human use for diverse purposes such as fiber, food, medicine and social and religious practices.1 Notably, the Atharvaveda’s recognition of Cannabis (Soma) as one of the five sacred plants renowned for its potential in alleviating various neurological disorders adds cultural and traditional significance to its historical use in medicinal practices. Within cannabis, CBD emerges as a pivotal constituent classified as a cannabinoid. The primary mode of action for cannabinoids involves the activation of Cannabinoid receptor type 1 (CB1) or Cannabinoid receptor type 2 (CB2), both of which function as G-Protein Coupled Receptors (GPCRs). CB1 receptors predominantly localize to neurons, exhibiting comparatively lower to moderate expression in peripheral tissues. Conversely, CB2 receptors primarily reside in immune cells, displaying a significantly reduced and restricted distribution within the Central Nervous System (CNS).2 An intriguing aspect of CBD is its lack of significant affinity for either CB1 or CB2 receptors, ensuring its safety from psychoactive effects.3 By not binding strongly to these receptors, CBD presents unique therapeutic potential without inducing the characteristic psychoactive response associated with cannabinoids.
In 1992, the groundbreaking discovery of the first Endocannabinoid, Anandamide (AEA), was made by William Devane and colleagues through its extraction from the pig brain.4 The endocannabinoid system comprises endogenous CB receptor agonists, CB receptors and proteins involved in the metabolism and regulation of endocannabinoids. Endocannabinoids belong to the class of lipid mediators and are derived from long-chain polyunsaturated fatty acids. The initial endocannabinoid identified, Arachidonoyl Ethanolamide (AEA), was initially detected in a pig brain in 1992 and later named anandamide, derived from the Sanskrit word “ananda,” meaning inner bliss in Indian.5 Subsequently, in 1994, the second endocannabinoid, 2-arachidonoylglycerol (2-AG), was discovered and extracted from the intestines of dogs and rats.
In the pathogenesis of Alzheimer’s Disease (AD), beta-Amyloid (Aβ) plays a crucial role in the disease’s pathophysiology, leading to chronic inflammation and impacting astrocytes and microglial cells.6 In vivo data has shown that CBD exhibits neuroprotective effects against hippocampal and cortical neurodegeneration, possesses anti-inflammatory and antioxidant properties,7 reduces tau hyperphosphorylation,8 and regulates the migration of microglial cells.9 CBD has been observed to enhance the immune system’s response and activate the autophagy pathway in APP/PS1 mice, potentially attenuating the degenerative processes in AD.10 Furthermore, CBD therapy has demonstrated improvements in various disease-relevant TAU58/2 transgenic mice, suggesting its potential in treating tauopathy-related behavioral dysfunctions.11
Astrocytes, the brain’s secondary regulating cells, play diverse roles in maintaining brain homeostasis, facilitating neurogenesis, establishing the microarchitecture of gray matter and providing protective functions through adaptive astrogliosis systems.12 Astroglial cells actively participate in the pathogenesis of various neurological diseases, including AD, amyotrophic lateral sclerosis, Parkinson’s disease and multiple dementias.13 In AD, astrocyte-associated Aβ plaques preserve microvessels and cells, which are crucial structures for brain tissue regulation through hypertrophic processes.14 During Aβ aggregation, neuronal involvement in calcium signaling impairs synaptic plasticity, inhibits astrocytic calcium signals and gliotransmitter release and disrupts intercellular communication.15 Consequently, various brain functions are affected, including blood flow, the Blood-Brain Barrier (BBB), synaptic activity, neurotrophin production and clearance of dead cells, as well as the balance of extracellular ions, fluid and neurotransmitters. This leads to synapse loss, neural network dysfunction and impaired neuronal signaling. The impact of astrocytes on early cognitive decline has been extensively discussed as a significant component of AD pathology.16
The isoforms of ApoE, particularly ApoE4, modulate neuroinflammation, cerebral lipid homeostasis, BBB integrity and regional blood flow in specific brain regions. In the context of pharmacologically induced endocannabinoid tone, it becomes crucial to determine whether specific ApoE alleles confer vulnerability or risk for adverse tissue events, even at commonly established safe doses of the FAAH inhibitor. This raises the question of whether assessing ApoE status is necessary for future therapeutic studies. Notably, the ApoE4 allele induces isoform-specific signaling events that significantly impact the proteolysis of the Amyloid Precursor Protein (APP), caspase activation, kinase activity and Sirtuin 1 activation.17
In this review, we aim to delve into the neurochemical, structural and functional aspects that shed light on the intricate mechanisms involved in microglial, astrocyte and synaptic plasticity in relation to the ApoE-Aβ (Apolipoprotein E-Amyloid beta) interaction. Furthermore, we explored the factors that direct the focus of CBD through various pathways to mitigate the risk of AD.
MATERIALS AND METHODS
Methods
This review article adopts a comprehensive approach by compiling the most recent and relevant information on the potential therapeutic targets of CBD for the management of neurodegenerative diseases. The search strategy employed a thorough literature search conducted on the PubMed, Scopus and Google Scholar databases. The search included a combination of keywords such as CBD, microglia activation, astrocytes, ApoE, mammalian Target of Rapamycin (mTOR) and Wingless-related integration site (Wnt) expression. These keywords were selected based on their relevance to the molecular targets associated with CBD and neurodegenerative diseases. The inclusion of these specific keywords ensures a comprehensive review of the current findings and molecular mechanisms related to the potential therapeutic effects of CBD.
Concept
Considering the pivotal role of ApoE dysfunction in the development of AD, along with the demonstrated effects of CBD in reducing microglial aggregation, modulating the Triggering Receptor Expressed on Myeloid cell 2 (TREM2) and Interleukin-33 (IL-33) and influencing the Transient Receptor Potential Vanilloid-1 (TRPV-1), Brain-Derived Neurotrophic Factor (BDNF), ATP-binding cassette transporter and mTOR, CBD emerges as a promising therapeutic agent for AD by exerting its influence on multiple pathways.
Role of Cannabidiol and Microglia
Microglia play a crucial role in surveilling the surrounding environment through their extensively branched processes, known as ramified primary microglia. They assess the synaptic activity and evaluate the surrounding milieu through intracellular signal transduction. In response to stimuli, microglia promptly react and enhance their functional capabilities in affected regions.18 The cell surface of microglia is equipped with several transporters, channels and receptors for neurotransmitters, cytokines and chemokines.19 Extensive research efforts have been devoted to unravel the precise involvement of microglia in the etiology of AD. In the AD brain, microglia encapsulate Amyloid-beta (Aβ) plaques and their altered morphology suggests a collective response to pathological challenges.20 Microglia contribute to the clearance of apoptotic cells and the elimination and maintenance of synapses for proper neural circuitry.21 Notably, cannabinoids have demonstrated neuroprotective properties by preventing microglial activation induced by Aβ and reducing tau hyperphosphorylation in both in vitro and in vivo studies.
Microglial activation is a prominent characteristic of brain pathology. Chronic activation of microglia leads to neuronal damage through the release of potentially neurotoxic substances, including proinflammatory cytokines, reactive oxygen species, Nicotinamide Adenine Dinucleotide Phosphate (NADPH) oxidase and Nitric Oxide (NO), which are activated by hypoxia,22 LPS,23 and amyloid beta.24 Aggregation of Amyloid beta (Aβ) and/ or tau fibrils stimulates Toll-Like Receptors (TLRs), members of the inflammasome family expressed in neurons, astrocytes and microglia. TLR2, 4 and 9 are specifically implicated in the pathology of AD (Kuwar et al., 2019). The correlation between TRPV1, PPARs, TLRs and microglia reflects the intricate balance between sensory perception, inflammation and immune responses in the CNS, as illustrated in Figure 1. CBD has been shown to possess anti-inflammatory potential by inhibiting the release of proinflammatory cytokines (TNF-α and IL-1β) and suppressing the stimulated NF-κB response, a crucial regulator of cellular behaviors including inflammatory responses and cellular growth.25 CBD has also demonstrated the ability to reduce microglial aggregation and migration, decrease Nitric Oxide (NO) production and modulate neuropathic pain in rodent models.26
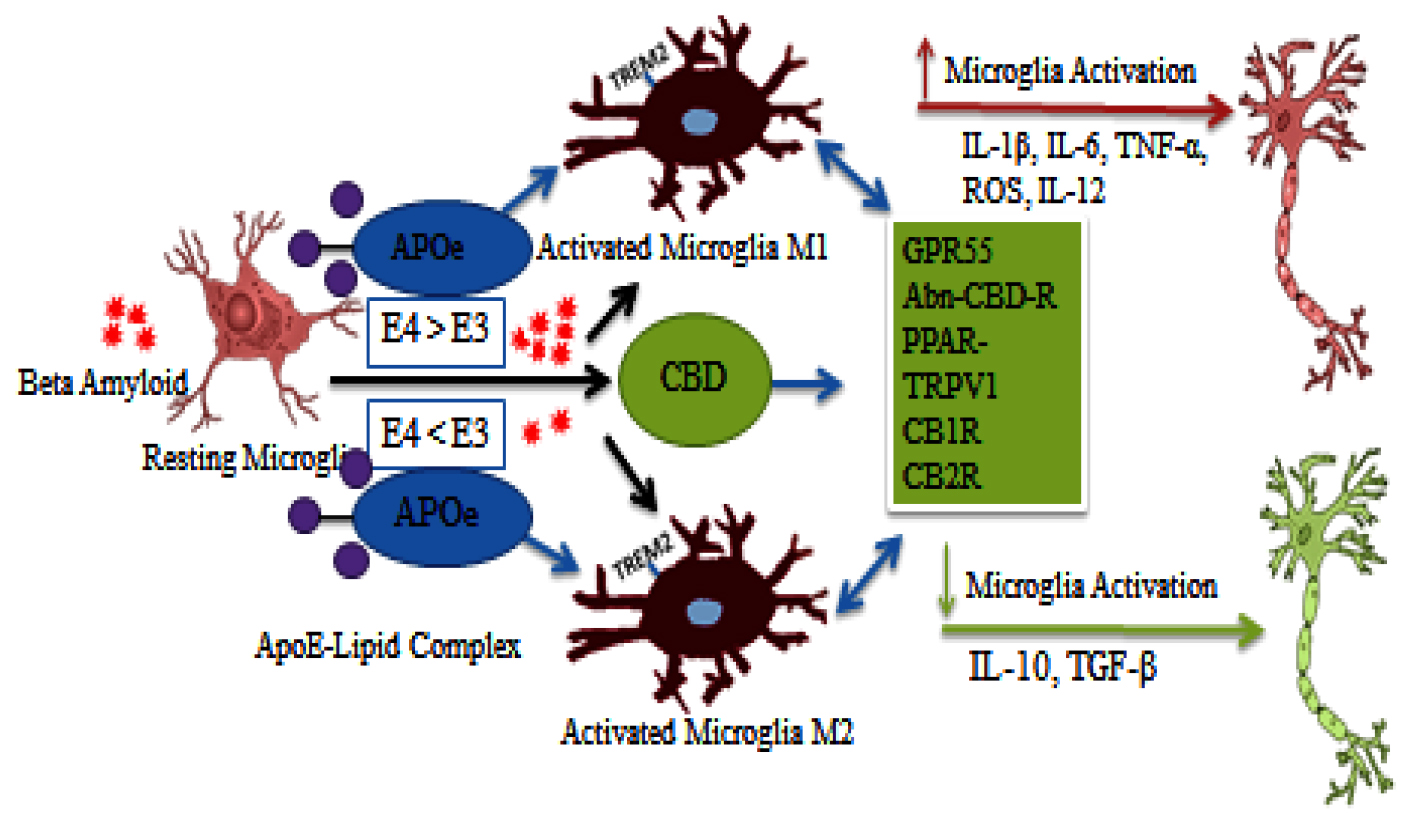
Figure 1:
Detailed depiction highlighting the significance of Aβ-induced aggregation, clearance pathways across the BBB, the impact of different ApoE alleles and the role of CBD in understanding the neurodegenerative processes. In the presence of an Aβ nucleus, ApoE is anticipated to counteract its amyloidogenic properties, thereby potentially impeding Aβ fibril formation. Furthermore, under specific conditions, ApoE and Aβ can form large aggregates. Newly synthesized Aβ fibrils can attach to pre-existing aggregates, leading to the formation of even larger aggregations, ultimately culminating in the development of amyloid plaques in the brain. It is noteworthy that ApoE4 exhibits a comparatively weaker affinity for Aβ when compared to ApoE3, highlighting the differential interactions of ApoE alleles with Aβ. Further investigation is warranted to elucidate the precise mechanisms underlying CBD’s involvement in these intricate processes and its potential therapeutic implications for neurodegenerative disorders.
In vitro studies using microglial cell lines have revealed that CBD can alter zinc homeostasis, oxidative stress and glutathione levels in unstimulated BV-2 cells. Moreover, CBD exerts its anti-inflammatory effects on microglia through the inhibition of NADPH oxidase synthesis and its intrinsic antioxidative activity. NADPH oxidase, responsible for the production of Reactive Oxygen Species (ROS), plays a significant role in mitigating adverse aspects of neurodegenerative diseases.27 CBD’s ability to attenuate microglial cell activation is also associated with mechanisms involving FAAH inhibition,28 PPAR-γ,29 and 5-HT1a receptors 30,31 in innate cells and lymphocytes.32
CBD, on the other hand, has a central role in regulating the TRPV1, PPAR and 5-HT1A receptors and acts as an antagonist for the GPR55 receptor. Its antioxidant and anti-inflammatory properties are partially mediated by its activities on TRPV1 and PPAR. PPARγ inhibits the production of inflammatory cytokines, such as TNF, IL-1 and IL-6, as well as the production of nitric oxide. Additionally, abnormal CBD receptor (Abn-CBD-Rs) has a neuroprotective effect through PPARα. CBD also increases cell viability by inhibiting ROS, nitrite production, lipid peroxidation and the expression of the protein inducible Nitric Oxide Synthase (iNOS).
One important component involved in the recognition and clearance of Aβ and extracellular amyloidogenic waste by microglia is the Triggering Receptor Expressed on Myeloid cells 2 (TREM2).33 TREM2 is a sensor for amyloid and is responsible for the recognition and digestion of Aβ, facilitated by ApoE.34 The recognition of Aβ and subsequent clearance by microglia is influenced by various extracellular TREM2 ligands, such as phospholipids, glycolipids, Lipoproteins (LDL and HDL) and apoptotic functions. Studies have shown that TREM2-deficient microglia exhibit decreased in vitro absorption of Aβ lipoprotein structures35 and increased evidence of Aβ internalization in vivo.36 Figure 2 illustrates VOSviewer analysis of 90 PubMed articles on ‘CBD-APOe-Microglia’, showing a significant cluster (Cluster 3, Item 33) emphasizing interconnectedness of CBD, APOe and microglial research.
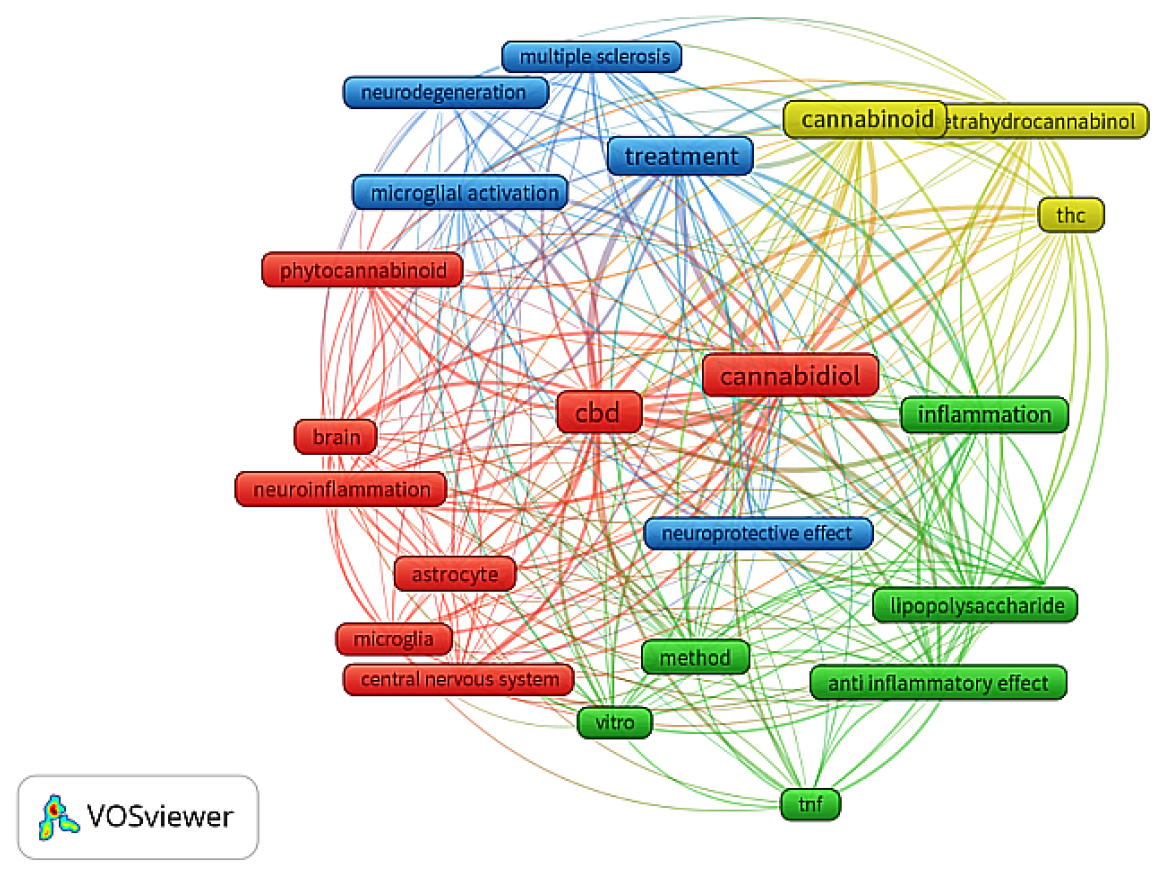
Figure 2:
The VOSviewer analysis of 90 PubMed articles related to ‘CBD-APOe-Microglia’ revealed a significant cluster (Cluster 3, Item 33) with 474 links and a total link strength of 1589. This cluster likely emphasizes the interconnectedness of research on CBD, APOe and microglial cells. The keywords associated with the search, such as neuroinflammation, neuroprotective effect and microglial activation, suggest a focus on CBD’s potential role in addressing neurodegenerative conditions like multiple sclerosis. The high link strength indicates a robust association between these key concepts, highlighting the importance of understanding the impact of CBD treatment on microglia and its potential neuroprotective effects. The presence of keywords like astrocyte and lipopolysaccharides suggests a broader exploration of CBD’s influence on the neuroinflammatory environment.
A recent study by Cao et al. (2020) investigated the impact of CBD on the TREM2 and the ApoE pathways in microglial phagocytosis. The study demonstrated that CBD treatment upregulated the expression of both TREM2 and ApoE in microglia, leading to enhanced clearance of extracellular and intracellular debris. This effect was mediated through the cannabinoid receptor 2 (CB2R) and involved the activation of the PI3K/AKT pathway.37 CBD has also been shown to regulate TREM2 and IL-33, which are associated with improved cognitive function. The TREM2-ApoE system plays a crucial role in the microglial clearance of various extracellular and intracellular debris, thereby potentially reducing neuronal damage in the neurodegenerative environment. Activation of TREM2 leads to suppression of the homeostatic microglial phenotype and initiation of neurodegenerative pathways.38
The immunomodulatory effects of CBD, including its ability to decrease inflammatory cytokine production and preserve microglial cells, might contribute to its observed effects on the TREM2-ApoE pathway. Activation of the TREM2-ApoE pathway has been associated with impairment of microglial regulation of brain immune function.
Role of Cannabidiol and Astrocyte
Astrocytes play vital roles in neuronal development, signaling and neurotransmitter regulation, including glutamate and ions such as potassium and calcium, throughout the healthy brain. They safeguard neurons, regulate cerebral blood flow and metabolism and facilitate neurotransmission, contributing to the central nervous system’s functions. Astrocytes are also involved in transporting cholesterol through ApoE-containing HDL-like particles, supporting the synaptic formation. One of the potential functions of ApoE receptor signaling in astrocytes is the modulation of synaptic pruning, a regular process that adjusts the number of neurons, synapses and axons in the central nervous system. Moreover, astrocytes contribute to brain plasticity by protecting glutamatergic receptors.39 Notably, astrocytes express high levels of low-density Lipoprotein Receptor (LRP1), which is crucial for the phagocytosis of damaged myelin and regulates multiple signaling pathways, potentially influencing Aβ endocytosis. In AD, the expression of LRP1 in glial cells may be upregulated due to neuroinflammation or suppressed in neurons because of postsynaptic damage. Studies have demonstrated that LRP1 antagonists effectively inhibit Aβ clearance via LRP1, highlighting the receptor’s significant role in AD development.40
CBD exhibits anti-inflammatory properties by inhibiting astrocyte activation and reducing the release of pro-inflammatory cytokines. Additionally, CBD stimulates the release of neurotrophic factors from astrocytes, promoting neuronal survival and synaptic plasticity. Neuroinflammation, associated with aging, is characterized by increased glycogen synthase kinase 3 beta (GSK-3β) activity and decreased activity of protein kinase B (Akt) and Wingless-related integration site (Wnt) pathways in the hippocampus of aged rats. The dysregulation of the Wnt/β-catenin/PI3K/Akt pathway leads to mitochondrial oxidative stress and the accumulation of Aβ protein inhibits the Wnt pathway. Enhancing Wnt signaling represents a promising therapeutic strategy for AD. Studies have shown that CBD reduces GSK-3 activity, promotes Wnt/LRP expression and subsequently mitigates oxidative stress in mitochondria and the inflammatory cascade in the brain.41
Neurodegenerative diseases often involve increased reactivity of Glial Fibrillary Acidic Protein (GFAP) and expression of ApoE in astrocytes located at brain synapses, believed to play a role in synaptogenesis through cholesterol release. In Parkinson’s disease and dementia, Koob et al. observed a reduction in nuclear and membrane-bound ApoE expression, leading to its redistribution to the cytoplasm, as well as decreased cholesterol levels in the astrocyte cell membrane. Advanced astrocyte function is marked by increased cell proliferation (cell hypertrophy) and activation of interstitial astrocyte filaments such as GFAP and vimentin. Elevated GFAP immunoreactivity in the hippocampal region of the Cornu Ammonis (CA1) following brain hypoxia correlates with the severity and progression of neuronal necrosis.42 CBD administration at a dose of 30 mg/kg for one week reduced the elevated production of GFAP in ischemia-treated mice.
While CB receptors have not been consistently detected in astrocytes, emerging evidence suggests the expression of CB1 and CB2 receptors in these cells, playing a crucial role in synaptic communication and inflammatory responses.43 CBD modulates several targets, including peroxisome proliferator-activated receptor gamma (PPAR-γ) (O’Sullivan, 2016), serotonin 5-H1A receptor,44 adenosine transporter A2A and Transient Receptor Potential vanilloid 1 (TRPV1).45 Furthermore, CBD demonstrates potent regulation of oxidative stress by scavenging superoxide radicals and inhibiting lipid peroxidation.46 In Figure 3, the VOSviewer analysis of PubMed articles on “CBD-APOe-astrocytes” (153 documents) focuses on Item22, Cluster3, Links178, revealing a network with a link strength of 1197. This network likely encompasses various aspects related to CBD, APOe and astrocytes, indicating a multifaceted connection. Keywords include tau protein, taupathy, astrocytic plaque, parkinsonism, age, dementia, globus pallidus, neuronal loss, hippocampus, neurodegeneration and neuroprotective effects, suggesting a comprehensive exploration of CBD’s potential impact on neurodegenerative conditions
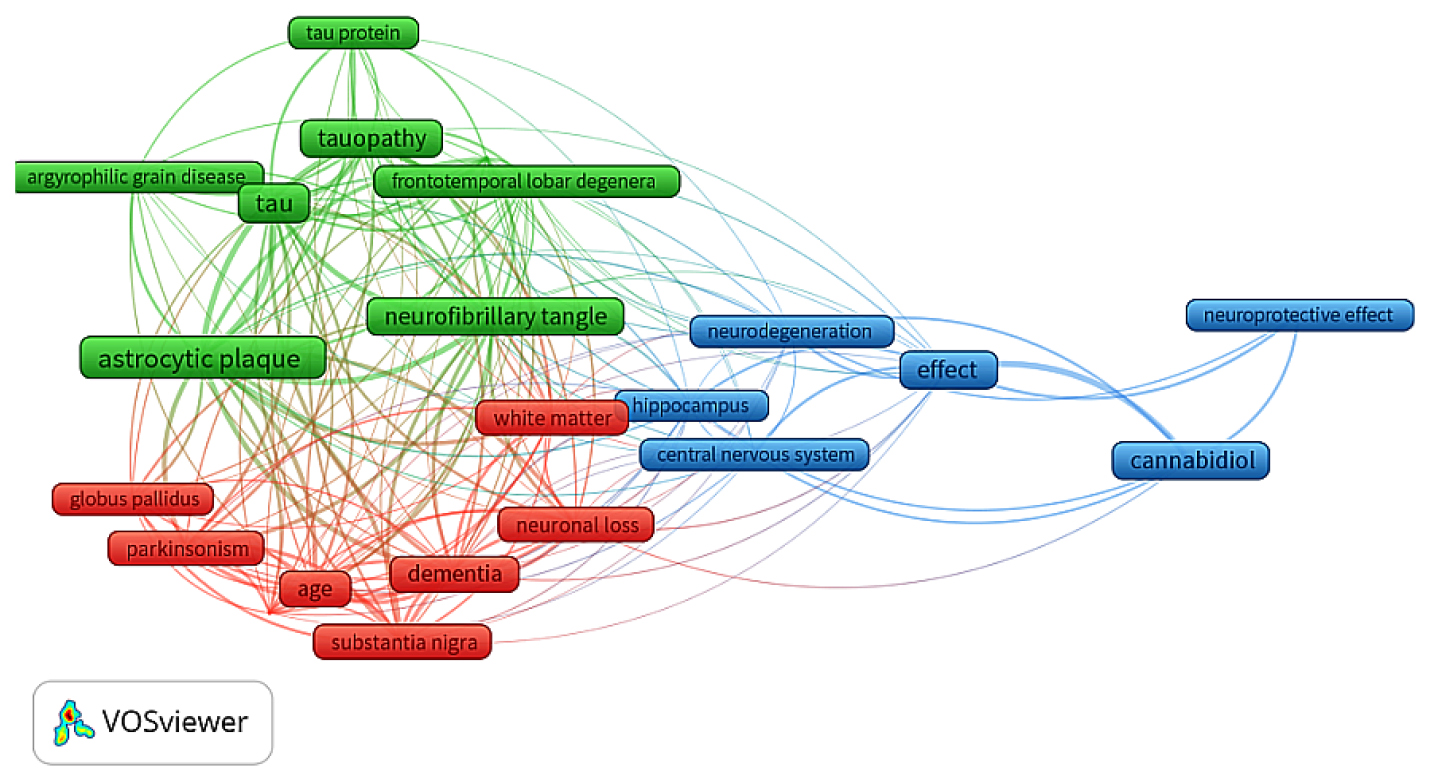
Figure 3:
In the VOSviewer analysis of the PubMed articles related to ‘‘CBD-APOe-astrocytes’ (153 total documents), the focus on Item22, Cluster3, with Links178 reveals a substantial network with a total link strength of 1197. This network likely encompasses various aspects related to CBD, APOe and astrocytes, suggesting a multifaceted connection between these elements. The keywords associated with the articles include tau protein, taupathy, astrocytic plaque, parkinsonism, age, dementia, globus pallidus, neuronal loss, hippocampus, neurodegeneration and neuroprotective effects. The intricate interplay of these terms suggests a comprehensive exploration of CBD’s potential impact on neurodegenerative conditions, including aspects of tau pathology, astrocytic involvement and protective effects. This analysis underscores the complex relationships within the literature, providing valuable insights into the intersection of CBD and neurodegenerative processes.
Role of Cannabidiol and Brain-Derived Neurotrophic Factor (BDNF)
Synaptic plasticity, a crucial process in the brain associated with learning and memory, involves modifications in synaptic strength through both pre- and postsynaptic mechanisms. Studies in mice have demonstrated that a single administration of CBD can rapidly and persistently induce antidepressant effects, potentially mediated by alterations in synaptic protein function. Acute CBD treatment has been shown to elevate Brain-Derived Neurotrophic Factor (BDNF) levels in the prefrontal medial cortex and hippocampus, suggesting CBD’s involvement in activating the BDNF signaling pathway to promote robust and enduring antidepressant effects. These effects appear to be associated with rapid changes in synaptic plasticity within the medial Prefrontal Cortex (mPFC) and can be blocked by the mTOR inhibitor.47 While CBD has limited impact on excitatory neurotransmitters, it does exhibit inhibitory effects on two forms of endogenous cannabinoid-mediated retrograde synaptic plasticity: depolarization-induced excitation suppression and metabotropic excitation suppression. The increased expression of BDNF induced by CBD may contribute to its neuroprotective effects. BDNF has been shown to have anti-apoptotic, anti-inflammatory and antioxidant properties, which can help protect neurons from damage and promote their survival. Additionally, BDNF is known to facilitate synaptic plasticity by promoting the formation and strengthening of synapses, which are crucial for learning and memory processes.48
Furthermore, CBD shows promise for treating of painful diabetic neuropathy, with its effectiveness potentially mediated by the activation of the serotonergic system. The involvement of ApoE2 receptors in the clearance of harmful reactive Aβ from synapses and the role of ApoE in Aβ metabolism in AD have been suggested, although the specific mechanisms and contribution of ApoE to non-Aβ-mediated processes in AD pathogenesis remain unclear.49 In Figure 4, the VOSviewer analysis of 48 PubMed articles related to ‘CBD-APOe-BDNF’ reveals a distinct cluster (Cluster 1) with 20 items linked by 147 connections, emphasizing significant associations between CBD, APOe and BDNF in neurogenesis and neuroinflammation
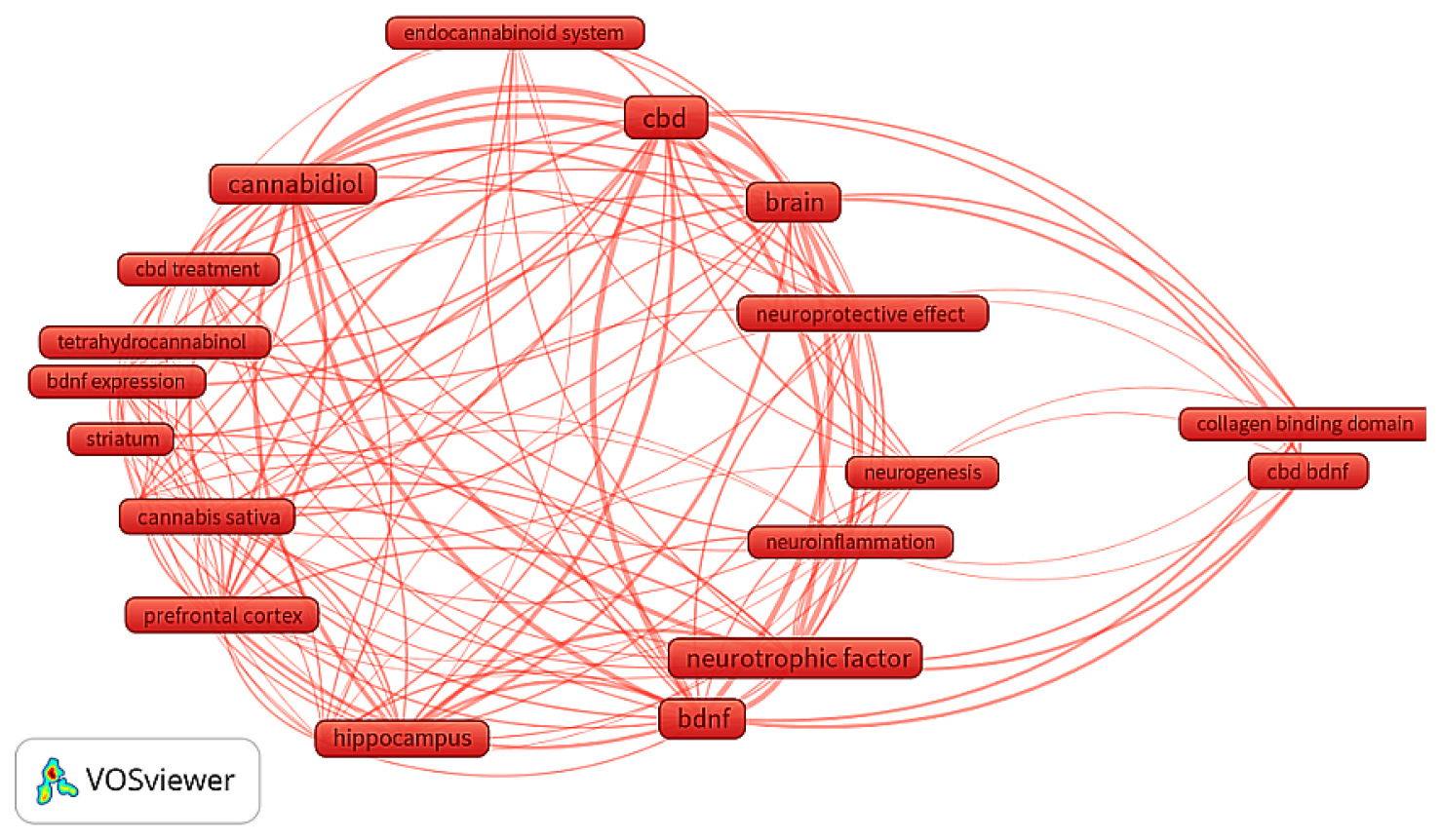
Figure 4:
In the VOSviewer analysis of 48 PubMed articles related to ‘CBD-APOe-BDNF’ with keywords including CBD, brain, neurotropic factor, hippocampus, prefrontal cortex, cannabis sativa, striatum, BDNF expression, neurogenesis, neuroinflammation and collagen binding domain, a distinct cluster (Cluster 1) comprising 20 items was identified. This cluster is linked by 147 connections with a total link strength of 794. The presence of CBD, APOe and BDNF in the cluster suggests a significant association between cannabidiol and brain-derived neurotrophic factor in the context of neurogenesis, neuroinflammation and related processes. The comprehensive analysis underscores the intricate relationship between CBD, BDNF and various brain regions, shedding light on potential therapeutic implications for conditions involving the hippocampus, prefrontal cortex and striatum.
Reelin, a crucial protein involved in neuronal migration and synaptic plasticity, exerts its effects primarily through the ApoE2 and VLDLR receptors. Studies on mice lacking reelin, VLDLR and ApoE2 have revealed hyperphosphorylation of the microtubule-stabilizing tau protein, indicating the regulatory role of reelin in microtubule function in neurons via the VLDLR and ApoE2 receptors. Dysregulation of reelin has been implicated in the development of AD pathology and may contribute to impairments in synaptic plasticity and memory. This is supported by findings of reduced Reelin levels in the hippocampus of AD patients.
Role of Cannabidiol and Apo-lipoprotein-E
The most common mutations observed in AD involve APP, Presenilin 1, Presenilin 2 and ApoE-4. Although the exact role of ApoE in the pathophysiology of AD is not fully understood, it is believed to be involved in Aβ deposition or clearance through direct protein-to-protein interaction. ApoE primarily modulates microglial activity to control neurodegeneration and has a minor role in regulating pathological tau and insoluble tau formation.50 The accumulation of amyloid pathology in the brain is influenced by ApoE, which can trigger both Aβ clearance and accumulation. The formation of ApoE-mediated plaques can lead to the aggregation of ApoE and therapeutic antibodies targeting ApoE aggregates are designed to reduce Aβ pathology, presenting a potential pathway for AD treatment.51
Studies have demonstrated that different isoforms of ApoE exhibit varying levels of affinity for the spontaneous accumulation of Aβ. Specifically, ApoE4 carriers have a higher frequency of Aβ deposition in senile plaques compared to non-carriers and ApoE4 promotes Aβ deposition in the brains of aged individuals with normal cognitive function.52 Additionally, a strong relationship has been observed between lower levels of ApoE in Cerebrospinal Fluid (CSF) and higher brain Aβ deposition, with decreased concentrations of ApoE in CSF being associated with increased Aβ accumulation.53
ApoE primarily binds to LDLR, with ApoE3 and ApoE4 exhibiting a 50-fold higher affinity for LDLR compared to ApoE2. It has been established that the presence of the ApoE4 variant, which carries an ApoE lipid/cholesterol transporter, confers a greater genetic risk for AD, while the ApoE2 variant has a preventive effect. In vitro studies have shown that incubation of ApoE with Aβ oligomers significantly affects its lipid binding ability, indicating that Aβ oligomers impact the physiological activity of ApoE.54 Dysfunctional exosome-mediated Aβ clearance has been proposed as a potential cause for elevated Aβ levels in the brain. Age-dependent reductions in exosome levels associated with ApoE4 have been observed in postmortem human brains and ApoE4 mouse brains with benign neuropathology.55 Figure 5 illustrates the complex interaction among Aβ aggregation, clearance mechanisms, cholesterol metabolism and cognition, emphasizing ApoE’s mediator role. Cholesterol enters neurons via HDL facilitated by ApoE. Key receptors, including LRP1, LDLR and HSPG, regulate Aβ clearance, modulated by ApoE. Deficiency of LDLR leads to ApoE accumulation, while its overexpression reduces ApoE levels, crucial for ApoE catabolism in the brain. CBD also affects Aβ clearance, cholesterol-related gene expression and ApoE interaction, potentially aiding in AD therapy.
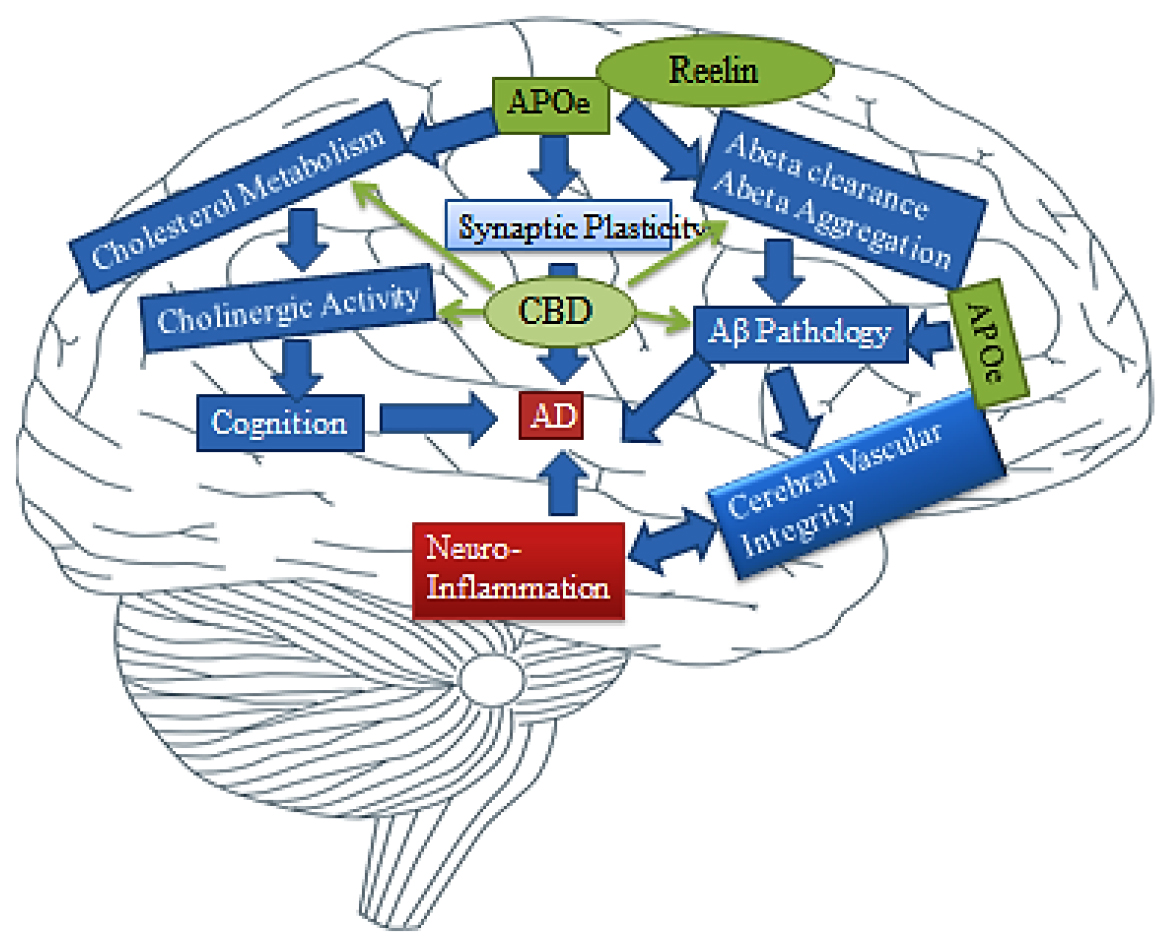
Figure 5:
Schematic representation illustrating the intricate interplay between Aβ-induced aggregation, clearance mechanisms, cholesterol metabolism and their impact on cognition, with a specific focus on the mediator role of ApoE. Cholesterol enters neurons through the action of HDL secreted by astrocytes, facilitated by the presence of ApoE. Crucial cell surface receptors including low-density Lipoprotein Receptor-Related Protein 1 (LRP1), Low-Density Lipoprotein receptor (LDLR) and heparan sulfate proteoglycan (HSPG) play pivotal roles in binding ApoE, Aβ and ApoE/Aβ complexes and are involved in major cellular pathways responsible for Aβ clearance. These receptors, directly or indirectly, regulate the clearance of Aβ, which is modulated by ApoE. Notably, deficiency of LDLR leads to the accumulation of ApoE in the brain, while the overexpression of LDLR reduces ApoE levels, highlighting the significance of LDLR in ApoE catabolism within the brain. Additionally, CBD has been shown to modulate Aβ clearance, influence cholesterol-related gene expression and interact with ApoE, potentially contributing to its therapeutic effects in AD.
Regarding amyloid pathology, ApoE2 and, to a lesser extent, ApoE3 exhibit hyperlipidation, leading to reduced Aβ aggregation and faster Aβ clearance from the brain (Safieh et al., 2019). Although ApoE is implicated in both vascular and parenchymal Aβ pathology, it appears to play a primary role in common carotid artery pathology. Previous studies have demonstrated that ApoE4/Aβ complexes are less stable than ApoE2 and ApoE3 complexes.56 Interestingly, the levels of ApoE/Aβ complexes are less stable in ApoE4 carriers and AD brains, resulting in higher concentrations of Aβ in the brain and CSF.
In the AD brain, there is a negative correlation between microRNA (miRNA) expression and ApoE, indicating the crucial role of miRNA in inhibiting Aβ clearance by suppressing ApoE expression.57 miRNAs have emerged as potential biomarkers for various diseases, including brain damage, neurodegeneration and mental illnesses. They play a critical role in multiple aspects of brain function, such as neurogenesis, neuronal development,58 and cellular processes involved in enhancing synaptic function.59
Research has demonstrated that under oxidative stress conditions, miRNA expression is reduced, potentially leading to decreased Aβ uptake and enhanced Aβ clearance during neuronal aging. Recent studies have shown that CBD modulates miRNA expression and targets specific miRNAs known to be affected by CBD. miR-33, abundantly expressed in different brain regions, has been found to increase Aβ synthesis in neurons by promoting APP cleavage when overexpressed. By controlling ABCA1 and Aβ levels in the brain, miR-33 overexpression reduces cellular cholesterol efflux and elevates extracellular Aβ levels, promoting Aβ production and impairing Aβ clearance.60 Conversely, the depletion of miR-33 enhances ApoE lipidation, facilitating extracellular Aβ breakdown and leading to a significant reduction in brain Aβ levels. These findings suggest that miR-33, through its modulation of ApoE and Aβ metabolism, could be a potential therapeutic target for AD.61
Furthermore, previous studies have demonstrated the essential role of the ATP-binding cassette transporter (ABCA1) in the active clearance of Aβ by neuronal and glial cells, with ApoE lipidation by ABCA1 playing a critical role in Aβ clearance.62 ABCA1 acts as a major regulator of cellular cholesterol and phospholipid homeostasis, making it a potential target for therapeutic interventions in AD. The impact of GPR55 on the expression of major cholesterol efflux transporters, including ABCA1, was also investigated. Activation of GPR55 led to a significant downregulation of the ABCA1 transporter, which was effectively prevented by CBD. Studies using mouse models of AD have demonstrated that increased expression of ABCA1 results in enhanced lipidation of ApoE and decreased accumulation of Aβ in the brain. Conversely, inhibition of ABCA1 in cultured neurons leads to reduced lipidation of ApoE and increased Aβ accumulation. These findings suggest that lipidated ApoE may be more efficient in clearing Aβ from the brain than non-lipidated ApoE. Dysfunction of ABCA1 may contribute to Aβ accumulation in the brain, particularly in individuals carrying the ApoE4 allele.62 ABCA1 is crucial for the active clearance of Aβ by neurons and glial cells and ApoE lipidation by ABCA1 plays a vital role in preventing AD.
In Figure 6, VOSviewer analysis of 20 PubMed articles related to ‘CBD-APOe’ reveals key themes: CBD, APOe, corticobasal generation, AD, brain, dementia, age and multiple system atrophy. Cluster 4, with 99 links and total link strength of 399, underscores interconnectedness, highlighting pivotal roles of ‘CBD’ and ‘APOe’. ApoE plays a crucial role in neurodegeneration by modulating microglial activity and regulating the accumulation and clearance of amyloid pathology in the brain. ApoE4 carriers have an increased susceptibility to Aβ deposition, while ApoE2 and ApoE3 exhibit preventive properties. miRNA has been identified as a significant regulator of Aβ clearance by negatively modulating ApoE expression. CBD has shown the ability to modulate the miRNA expression, suggesting its potential as a therapeutic target for AD. miR-33 has emerged as a potential therapeutic target for AD due to its modulation of ApoE and Aβ metabolism.
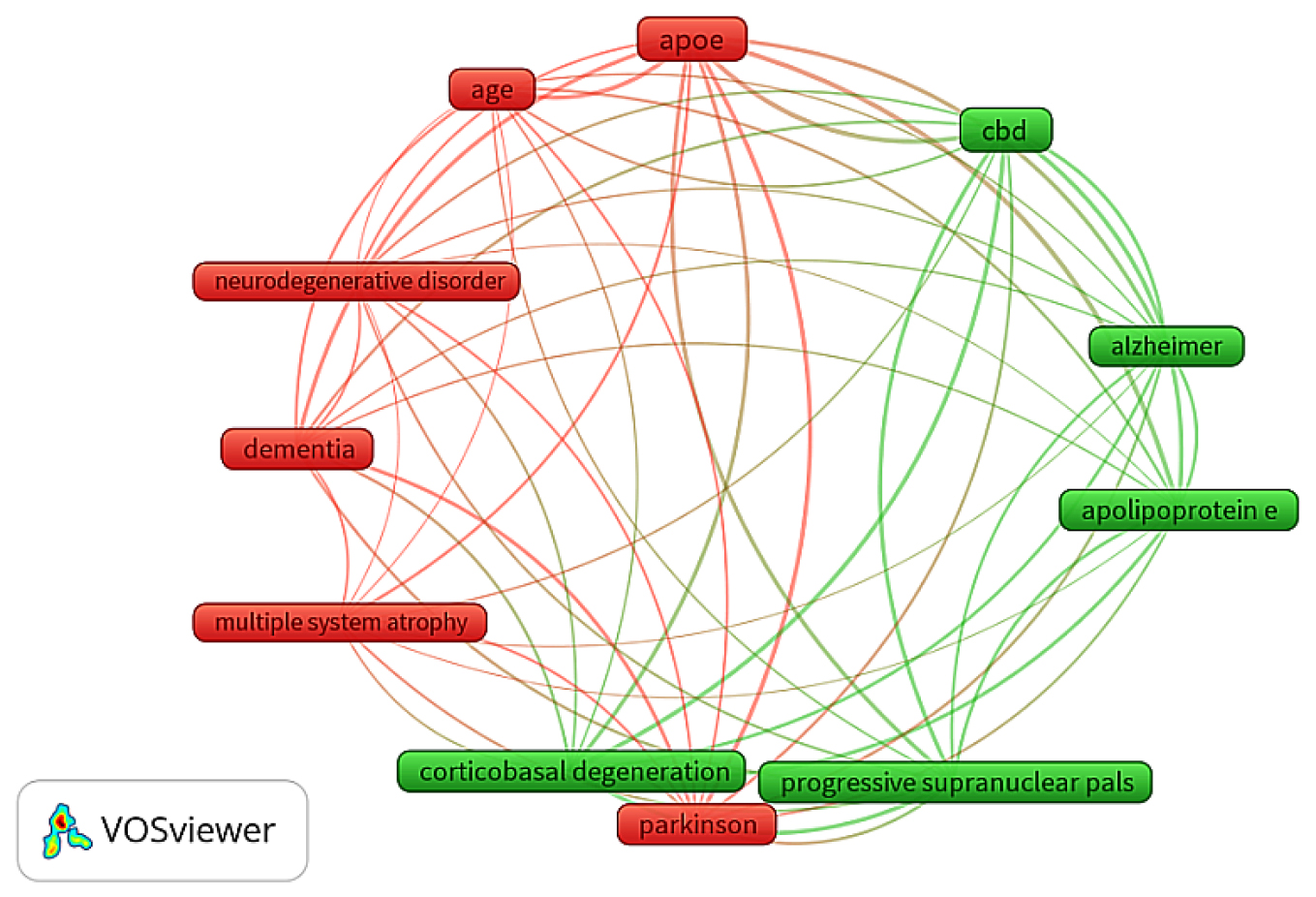
Figure 6:
In VOSviewer analysis of 20 PubMed articles related to ‘CBD-APOe’ search, the focus was on key themes such as CBD, APOe, corticobasal generation, AD, brain, dementia, age and multiple system atrophy. The analysis identified a distinct cluster (Cluster 4) with 99 links and total link strength of 399, suggesting a strong interconnection between articles within this cluster. The keywords ‘CBD’ and ‘APOe’ likely played pivotal roles in establishing these connections. The results underscore the relevance and interconnected nature of research on CBD and APOe in the context of corticobasal generation, AD, brain health, dementia, age-related considerations and multiple system atrophy, providing insights into the intricate relationships within this review domain.
Role of Cannabidiol and mammalian target of Rapamycin (mTOR)
Autophagy plays a crucial role in the metabolism of Aβ and tau pathology in AD. Dysregulated autophagy has been implicated in the pathogenesis of AD and targeting autophagy regulation could offer a novel therapeutic approach. In the early stages of AD, the presence of Aβ and tau can induce autophagy through both mTOR-dependent and mTOR-independent pathways to facilitate their clearance.63 In Figure 7, the VOSviewer analysis of 48 PubMed articles on ‘CBD-APOe-mTOR’ illustrates significant clusters (Item15, Cluster2) with 73 links and a total link strength of 167. It focuses on CBD, APOe, mTOR, etc., indicating complex interrelationships. The mTOR pathways, which control various cellular processes such as cell growth, ribosome biosynthesis, translational regulation and autophagy, are implicated in AD.64
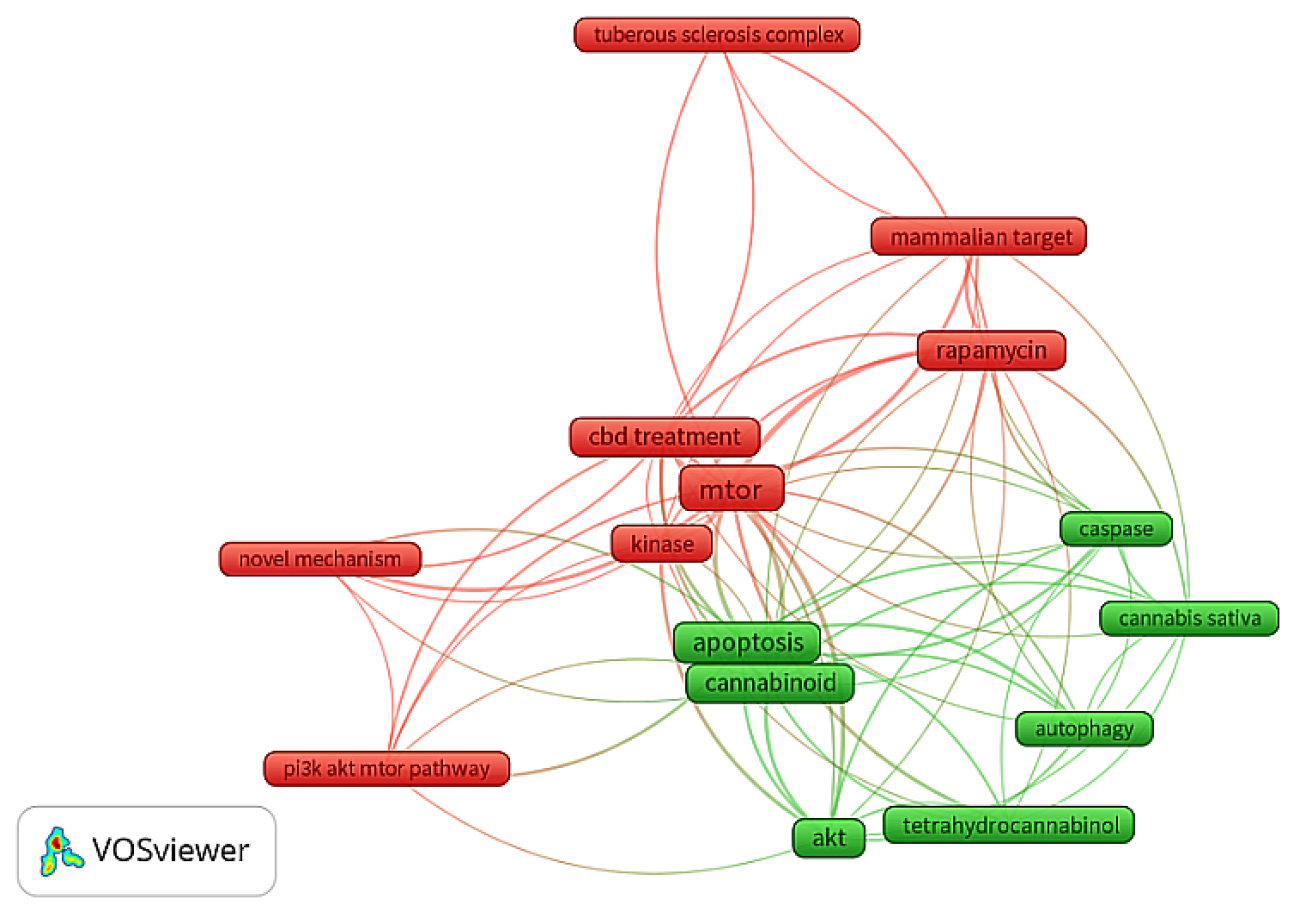
Figure 7:
The VOSviewer analysis of 48 PubMed articles with the search term ‘CBD-APOe-mTOR’ revealed a significant cluster marked as Item15, Cluster2, comprising 73 links with a total link strength of 167. The study focuses on key elements like CBD, APOe, mTOR, tuberous sclerosis complex, mammalian Target of Rapamycin (mTOR), caspase, cannabis sativa, autophagy, Akt, PI3K-Akt-mTOR pathway, novel mechanisms, kinase and CBD treatment. The clustering and link strength indicate a substantial network of interconnected concepts, suggesting an intricate relationship between CBD, APOe and mTOR pathways in the context of tuberous sclerosis complex, autophagy and novel mechanisms. This comprehensive analysis provides valuable insights into the multifaceted interactions within this research domain, potentially uncovering new avenues for understanding CBD’s impact on mTOR-related pathways.
Activation of mTOR has been shown to modulate the expression and secretion of ApoE in astrocytes, indicating a potential regulatory role of mTOR in ApoE metabolism. Particularly, the ApoE4 isoform, a major genetic risk factor for late-onset AD, has been implicated in dysregulating mTOR signaling. Studies have demonstrated increased mTOR activity in ApoE4-expressing cells compared with cells expressing the more common ApoE3 isoform.65 The dysregulation of mTOR signaling in the presence of ApoE4 may have detrimental effects on various cellular processes implicated in AD pathogenesis. Aberrant activation of mTOR has been associated with increased production and accumulation of Aβ peptides, a hallmark of AD.66 Moreover, mTOR dysregulation can impair autophagy, a crucial cellular process for the clearance of misfolded proteins, including Aβ and tau, which are key players in AD pathology.67 Figure 8 illustrates molecular interactions and pathways in Aβ protein accumulation in AD brains and CBD’s effects. Aβ accumulation increases ROS, decreases PI3K/Akt and reduces Wnt signaling. CBD counters this by enhancing Wnt/β-catenin, PI3K/Akt and inhibiting GSK-3β
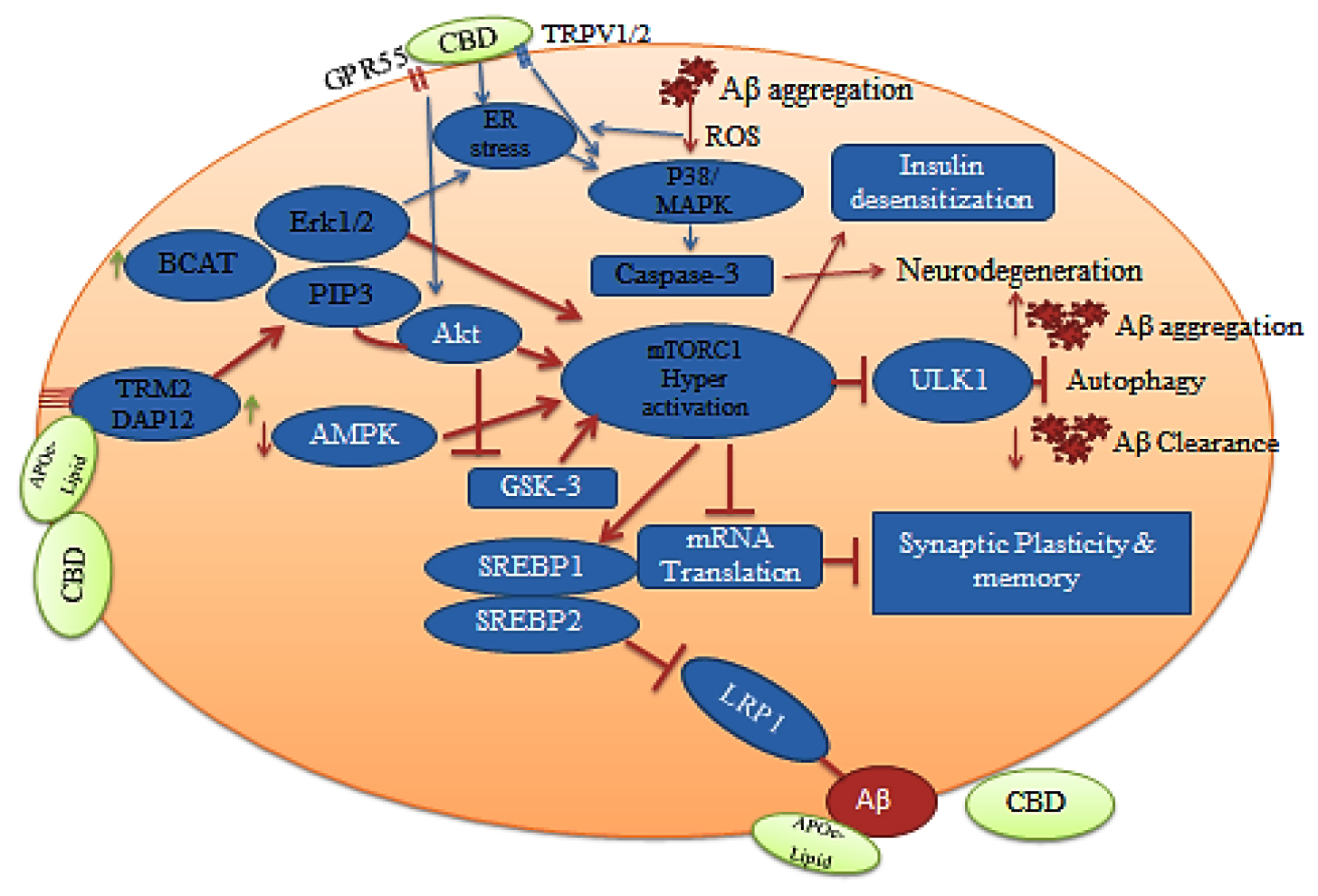
Figure 8:
Schematic representation of the molecular interactions and pathways involved in the accumulation of Aβ protein in the AD brain and the effects of CBD on key signaling pathways and processes. The accumulation of Aβ protein leads to increased ROS levels and a decrease in the activity of Phosphoinositide 3-Kinase (PI3K) and Akt. Additionally, Aβ accumulation results in a reduction in Wnt signaling and degradation of β-catenin. The down-regulation of β-catenin expression further decreases PI3K/Akt signaling. CBD acts by reducing Glycogen Synthase Kinase-3β (GSK-3β) activity, which leads to increased Wnt/β-catenin and PI3K/ Akt pathway activity, as well as a reduction in oxidative stress in AD. The activation of Peroxisome Proliferator-Activated Receptor-gamma (PPAR-gamma) by CBD contributes to its effects. CBD also stimulates the ubiquitination of APP and inhibits the accumulation of Aβ, ApoE4 and TREM2, which have distinct roles in AD pathogenesis. Lipidated ApoE binds to Aβ, facilitating its internalization or transport to different cell types. TREM2 expressed in microglia mediates the clearance of Aβ driven by ApoE. Furthermore, Aβ-induced microglial activation serves as a mediator in the interaction between ApoE and CBD.
Furthermore, targeting the mTOR pathway has been proposed as a potential therapeutic strategy for AD. Inhibition of mTOR has been shown to reduce Aβ levels, enhance autophagy and improve cognitive function in animal models of AD.68 Importantly, recent evidence suggests that mTOR inhibition may specifically benefit individuals carrying the ApoE4 allele, potentially mitigating the negative effects associated with this risk factor.
Notably, CBD has been found to have a significant impact on mTOR signaling, particularly in the dopaminergic activity within the neural mesolimbic pathway mediated by the 5-HT1A serotonin receptor. CBD has demonstrated the ability to modulate rpS6, a marker for monitoring neuronal activity, by activating mTOR and reducing glutamate levels in the brain. Therefore, CBD may modulate crucial pathways for neuronal survival, including autophagy and other cell survival regulatory pathways. Additionally, CBD has shown the potential to alleviate cell viability impairment induced by the neurotoxin MPP+ in SH-SY5Y cells by modulating the activity of Bax and caspase 3, key regulators of cell death and apoptosis.69 Its defensive actions are mediated by the stimulation of the ERK and Akt/mTOR pathways, which play crucial roles in cell survival, differentiation, proliferation and metabolism.70 The activation of CBD-induced ERK is regulated through its association with CB2 and TRPV1. Moreover, CBD has been found to modulate the c-Jun-N-terminal Kinase (JNK) pathway, a component of the MAPK signaling pathway involved in regulating cellular processes like proliferation and apoptosis. By downregulating the PI3K-Akt prosurvival cascade, CBD influences the balance between cell death and survival.71 Additionally, cannabinoids, including CBD, modulate lipid and glucose metabolism as well as inflammatory processes through the endocannabinoid AEA, leading to neuroadaptive changes that improve depressive-like responses by acting as CB1 receptor agonists.72
CONCLUSION AND FUTURE PERSPECTIVES
Despite the availability of scattered data on cannabis bioactives like CBD, numerous studies have proposed several potential molecular targets that underlie the therapeutic effects of CBD, including CB1, CB2, GPR55, 5-HT1A, TRPV1, A2A, PPARs and opioid receptors. While the initial belief that CBD solely acts via the endocannabinoid system has been mostly rejected, the existing research has not adequately explored the direct correlation between ApoE as a target and CBD-based therapeutics. Our systematic review highlights the limited research available to support the connection between ApoE and CBD-mediated therapeutic functions. Further analysis of existing literature and new research is necessary to definitively establish CBD’s active targets.
Determining the specific molecular targets responsible for CBD’s neurodegenerative effects presents a challenge. Nonetheless, our systematic review has identified several targets based on published in vitro data, preclinical investigations and clinical signals. To validate these targets, focused studies are crucial in establishing direct links between ApoE and the effects of CBD. These studies will contribute to a deeper understanding of the therapeutic potential of CBD in the context of ApoE-related conditions.
ACKNOWLEDGEMENT
The authors would like to express their gratitude to the Institute of Pharmacy, Nirma University, for providing financial support (MRP Project-NU/DRI/MinResPrj/APl/2019-20) to conduct this research and prepare the manuscript. The authors also acknowledge the Institute of Pharmacy for providing the necessary resources and technical assistance to carry out the research work, which is a part of the Doctor of Philosophy (Ph.D.) research program to be submitted to Nirma University.
ABBREVIATIONS
CBD | Cannabidiol |
---|---|
ApoE | Apolipoprotein E |
GPCR | G-protein coupled receptors Introduction |
CB | Cannabinoid receptor |
AD | Alzheimer’s disease |
Aβ | Beta amyloid |
BDNF | Brain derived neurotrophic factor |
mTOR | mammalian target of rapamycin |
ApoE-Aβ | Apolipoprotein E-Amyloid beta |
NADPH | Nicotinamide adenine dinucleotide phosphate |
TLR | Toll-like receptors |
PPAR-γ | Peroxisome proliferators-activated receptor γ |
TREM2 | Triggering receptor expressed on myeloid cells 2 |
LRP1 | Low density lipoprotein receptor |
GSK-3 | Glycogen synthase kinase 3 beta |
Akt | Protein kinase B |
Wnt | Wingless-related integration site |
TRPV1 | Transient receptor potential vanilloid 1 |
miRNA | microRNA |
JNK | c-Jun N-terminal kinase |
2-AG | 2-arachidonoylglycerol |
mPFC | medial prefrontal cortex |
References
- Kuddus M, Ginawi IAM, Al-Hazimi A. Cannabis sativa: An ancient wild edible plant of India. Emirates J Food Agric. 2013;25(10):736-45. [CrossRef] | [Google Scholar]
- Pertwee RG. The diverse CB 1 and CB 2 receptor pharmacology of three plant cannabinoids: Δ 9-tetrahydrocannabinol, cannabidiol and Δ 9-tetrahydrocannabivarin. Br J Pharmacol. 2008;153(2):199-215. [CrossRef] | [Google Scholar]
- Gobira PH, Vilela LR, Gonçalves BDC. Cannabidiol, a Cannabis sativa constituent, inhibits cocaine-induced seizures in mice: Possible role of the mTOR pathway and reduction in glutamate release. Neurotoxicology. 2015;50:116-21. [CrossRef] | [Google Scholar]
- Pertwee RG. Pharmacological actions of cannabinoids. Handb Exp Pharmacol. 2005;168:1-51. [CrossRef] | [Google Scholar]
- Mechoulam R, Ben-Shabat S, Hanus L. Identification of an endogenous 2-monoglyceride, present in canine gut, that binds to cannabinoid receptors. Biochem Pharmacol. 1995;50(1):83-90. [CrossRef] | [Google Scholar]
- Arcuri C, Mecca C, Bianchi R, Giambanco I, Donato R. The pathophysiological role of microglia in dynamic surveillance, phagocytosis and structural remodeling of the developing CNS. Front Mol Neurosci. 2017:10 [CrossRef] | [Google Scholar]
- Aso E, Ferrer I. CB2 Cannabinoid Receptor As Potential Target against Alzheimer’s Disease. Front Neurosci. 2016:10 [CrossRef] | [Google Scholar]
- Van Der Stelt M, Mazzola C, Esposito G. Endocannabinoids and β-amyloid-induced neurotoxicity in vivo: Effect of pharmacological elevation of endocannabinoid levels. Cell Mol Life Sci. 2006;63(12):1410-24. [CrossRef] | [Google Scholar]
- Martín-Moreno AM, Reigada D, Ramírez BG. Cannabidiol and other cannabinoids reduce microglial activation in vitro and in vivo: Relevance to alzheimer’s disease. Mol Pharmacol. 2011;79(6):964-73. [CrossRef] | [Google Scholar]
- Hao Fengjin, YF. Cannabidiol (CBD) enhanced the hippocampal immune response and autophagy of APP/PS1 Alzheimer’s mice uncovered by RNA-seq. Life Sci. 2021;264(3):113812 [CrossRef] | [Google Scholar]
- Kreilaus Fabian, Przybyla Magdalena, Ittner Lars, TK. Cannabidiol (CBD) treatment improves spatial memory in 14-month-old female TAU58/2 transgenic mice. Behav Brain Res. 2022;425(4):113812 [CrossRef] | [Google Scholar]
- Gómez-Gónzalez B, Cabañas-Morales AM, Hurtado-Alvarado G, Velázquez-Moctezuma J. Neuronal-glial-endothelial interactions regulate central nervous system homeostasis. Endothelial Cell Plasticity in the Normal and Injured Central Nervous System. ;2015:36-75. [CrossRef] | [Google Scholar]
- Verkhratsky A, Olabarria M, Noristani HN, Yeh CY, Rodriguez JJ. Astrocytes in Alzheimer’s Disease. Neurotherapeutics. 2010;7(4):399-412. [CrossRef] | [Google Scholar]
- Cullen KM. Perivascular astrocytes within Alzheimer’s disease plaques. Neuroreport. 1997;8(8):1961-6. [CrossRef] | [Google Scholar]
- Volterra A, Meldolesi J. Astrocytes, from brain glue to communication elements: The revolution continues. Nat Rev Neurosci. 2005;6(8):626-40. [CrossRef] | [Google Scholar]
- Dallérac G, Rouach N. Astrocytes as new targets to improve cognitive functions. Prog Neurobiol. 2016;144:48-67. [CrossRef] | [Google Scholar]
- Hunsberger HC, Pinky PD, Smith W, Suppiramaniam V, Reed MN. The role of APOE4 in Alzheimer’s disease: strategies for future therapeutic interventions. Neuronal Signal. 2019;3(2):1-15. [CrossRef] | [Google Scholar]
- Bernier LP, Bohlen CJ, York EM. Nanoscale Surveillance of the Brain by Microglia via cAMP-Regulated Filopodia. Cell Rep. 2019;27(10):2895-908. [CrossRef] | [Google Scholar]
- Helmut K, Hanisch UK, Noda M, Verkhratsky A. Physiology of microglia. Physiol Rev. 2011;91(2):461-553. [CrossRef] | [Google Scholar]
- Bouvier DS, Murai KK. Synergistic actions of microglia and astrocytes in the progression of Alzheimer’s disease. J Alzheimer’s Dis. 2015;45(4):1001-14. [CrossRef] | [Google Scholar]
- Luo H, Rossi E, Saubamea B. Cannabidiol Increases Proliferation, Migration, Tubulogenesis and Integrity of Human Brain Endothelial Cells through TRPV2 Activation. Mol Pharm. 2019;16(3):1312-26. [CrossRef] | [Google Scholar]
- Yang JJ, Wang SJ, Gao XL. Toll-like receptor 4 (TLR-4) pathway promotes pulmonary inflammation in chronic intermittent hypoxia-induced obstructive sleep apnea. Med Sci Monit. 2018;24:7152-61. [CrossRef] | [Google Scholar]
- Zhao J, Bi W, Xiao S. Neuroinflammation induced by lipopolysaccharide causes cognitive impairment in mice. Sci Rep. 2019;9(1) [CrossRef] | [Google Scholar]
- Caldeira C, Cunha C, Vaz AR. Key aging-associated alterations in primary microglia response to beta-amyloid stimulation. Front Aging Neurosci. 2017;9:277 [CrossRef] | [Google Scholar]
- Tsuda M. Microglia in the spinal cord and neuropathic pain. J Diabetes Investig. 2016;7(1):17-26. [CrossRef] | [Google Scholar]
- Inoue K, Tsuda M. Microglia in neuropathic pain: Cellular and molecular mechanisms and therapeutic potential. Nat Rev Neurosci. 2018;19(3):138-52. [CrossRef] | [Google Scholar]
- dos-Santos-Pereira M, Guimarães FS, Del-Bel E, Raisman-Vozari R, Michel PP. Cannabidiol prevents LPS-induced microglial inflammation by inhibiting ROS/NF-κB-dependent signaling and glucose consumption. Glia. 2020;68(3):561-73. [CrossRef] | [Google Scholar]
- Syu Ren, Zzhen Wang, Zhang Y, Nhong Chen. Potential application of endocannabinoid system agents in neuropsychiatric and neurodegenerative diseases-focusing on FAAH/MAGL inhibitors. Acta Pharmacol Sin. 2020;41(10):1263-1271. [CrossRef] | [Google Scholar]
- Hind WH, England TJ, O’Sullivan SE. Cannabidiol protects an in vitro model of the blood-brain barrier from oxygen-glucose deprivation via PPARγ and 5-HT1A receptors. Br J Pharmacol. 2016;173(5):815-25. [CrossRef] | [Google Scholar]
- Pazos MR, Mohammed N, Lafuente H. Mechanisms of cannabidiol neuroprotection in hypoxic-ischemic newborn pigs: Role of 5HT1A and CB2 receptors. Neuropharmacology. 2013;71:282-91. [CrossRef] | [Google Scholar]
- Fernández-Ruiz J, Sagredo O, Pazos MR. Cannabidiol for neurodegenerative disorders: Important new clinical applications for this phytocannabinoid. Br J Clin Pharmacol. 2013;75(2):323-33. [CrossRef] | [Google Scholar]
- Nichols JM, Kaplan BLF. Immune Responses Regulated by Cannabidiol. Cannabis Cannabinoid Res. 2020;5(1):12-31. [CrossRef] | [Google Scholar]
- Krasemann S, Madore C, Cialic R. The TREM2-APOE Pathway Drives the Transcriptional Phenotype of Dysfunctional Microglia in Neurodegenerative Diseases. Immunity. 2017;47(3):566-81. [CrossRef] | [Google Scholar]
- Singh AK, Mishra G, Maurya A. Role of TREM2 in Alzheimer’s Disease and its Consequences on β-Amyloid, Tau and Neurofibrillary Tangles. Curr Alzheimer Res. 2019;16(13):1216-29. [CrossRef] | [Google Scholar]
- Ulland TK, Song WM, Huang SCC. TREM2 Maintains Microglial Metabolic Fitness in Alzheimer’s Disease. Cell. 2017;170(4):649-63. [CrossRef] | [Google Scholar]
- Wang JZ, Xia YY, Grundke-Iqbal I, Iqbal K. Abnormal hyperphosphorylation of tau: Sites, regulation and molecular mechanism of neurofibrillary degeneration. Adv Alzheimer’s Dis. 2012;3:123-39. [CrossRef] | [Google Scholar]
- Joly-Amado A, Kulkarni N, Nash KR. Reelin Signaling in Neurodevelopmental Disorders and Neurodegenerative Diseases. Brain Sci. 2023;13(10) [CrossRef] | [Google Scholar]
- Yeh FL, Wang Y, Tom I, Gonzalez LC, Sheng M. TREM2 Binds to Apolipoproteins, Including APOE and CLU/APOJ and Thereby Facilitates Uptake of Amyloid-Beta by Microglia. Neuron. 2016;91(2):328-40. [CrossRef] | [Google Scholar]
- Dzamba D, Harantova L, Butenko O, anderova M. Glial Cells – The Key Elements of Alzheimers Disease. Curr Alzheimer Res. 2016;13(8):894-911. [CrossRef] | [Google Scholar]
- Liu CC, Hu J, Zhao N. Astrocytic LRP1 mediates brain Aβ clearance and impacts amyloid deposition. J Neurosci. 2017;37(15):4023-4031. [CrossRef] | [Google Scholar]
- Esposito G, De Filippis D, Carnuccio R, Izzo AA, Iuvone T. The marijuana component cannabidiol inhibits β-amyloid-induced tau protein hyperphosphorylation through Wnt/β-catenin pathway rescue in PC12 cells. J Mol Med. 2006;84(3):253-58. [CrossRef] | [Google Scholar]
- Wilhelmsson U, Li L, Pekna M. Absence of glial fibrillary acidic protein and vimentin prevents hypertrophy of astrocytic processes and improves post-traumatic regeneration. J Neurosci. 2004;24(21):5016-21. [CrossRef] | [Google Scholar]
- Metna-Laurent M, Marsicano G. Rising stars: Modulation of brain functions by astroglial type-1 cannabinoid receptors. Glia. 2015;63(3):353-364. [CrossRef] | [Google Scholar]
- De Gregorio D, McLaughlin RJ, Posa L. Cannabidiol modulates serotonergic transmission and reverses both allodynia and anxiety-like behavior in a model of neuropathic pain. Pain. 2019;160(1):136-50. [CrossRef] | [Google Scholar]
- Gray RA, Stott CG, Jones NA. Anticonvulsive Properties of Cannabidiol in a Model of Generalized Seizure Are Transient Receptor Potential Vanilloid 1 Dependent. Cannabis Cannabinoid Res. 2020;5(2):145-9. [CrossRef] | [Google Scholar]
- Atalay S, Jarocka-karpowicz I, Skrzydlewskas E. Antioxidative and anti-inflammatory properties of cannabidiol. Antioxidants. 2020;9(1) [CrossRef] | [Google Scholar]
- García-Gutiérrez MS, Navarrete F, Gasparyan A, Austrich-Olivares A, Sala F, Manzanares J, et al. Cannabidiol: A potential new alternative for the treatment of anxiety, depression and psychotic disorders. Biomolecules. 2020;10(11):1-34. [CrossRef] | [Google Scholar]
- Campos AC, Fogaça MV, Scarante FF. Plastic and neuroprotective mechanisms involved in the therapeutic effects of cannabidiol in psychiatric disorders. Front Pharmacol. 2017;8:269 [CrossRef] | [Google Scholar]
- Badhe RV, Chejara DR, Kumar P, Choonara YE, Pillay V. Neurodegenerative disease conditions and genomic treatment for better health. Genomics-Driven Healthcare: Trends in Disease Prevention and Treatment. 2018:281-310. [CrossRef] | [Google Scholar]
- Safieh M, Korczyn AD, Michaelson DM. ApoE4: an emerging therapeutic target for Alzheimer’s disease. BMC Med. 2019;17(1) [CrossRef] | [Google Scholar]
- Liao F, Li A, Xiong M. Targeting of nonlipidated, aggregated apoE with antibodies inhibits amyloid accumulation. Am Soc Clin Investig. 2018;128.5:2144-55. [CrossRef] | [Google Scholar]
- Caselli RJ, Dueck AC, Osborne D. Longitudinal modeling of age-related memory decline and the APOE ε4 effect. N Engl J Med. 2009;361(3):255-63. [CrossRef] | [Google Scholar]
- Sheline YI, Morris JC, Snyder AZ. APOE4 allele disrupts resting state fMRI connectivity in the absence of amyloid plaques or decreased CSF Aβ42. J Neurosci. 2010;30(50):17035-40. [CrossRef] | [Google Scholar]
- Mahley RW, Rall SC. A POLIPOPROTEIN E: Far More Than a Lipid Transport Protein. Annu Rev Genomics Hum Genet. 2000;1(1):507-37. [CrossRef] | [Google Scholar]
- Theendakara V, Patent A, Peters Libeu CA, Philpot B, Flores S, Descamps O, Poksay KS, Zhang Q, Cailing G, Hart M, John V, et al. Neuroprotective Sirtuin ratio reversed by ApoE4. Proc Natl Acad Sci U S A. 2013;110(45):18303-8. [CrossRef] | [Google Scholar]
- Tai LM, Mehra S, Shete V. Soluble apoE/Aβ complex: Mechanism and therapeutic target for APOE4-induced AD risk. Mol Neurodegener. 2014;9(1):2 [CrossRef] | [Google Scholar]
- Wang M, Qin L, Tang B. MicroRNAs in Alzheimer’s disease. Front Genet. 2019:10 [CrossRef] | [Google Scholar]
- Cochella L, Hobert O. Current Topics in Developmental Biology. 2012;99:115-43. [CrossRef] | [Google Scholar]
- Goldie BJ, Cairns MJ. Post-transcriptional trafficking and regulation of neuronal gene expression. Mol Neurobiol. 2012;45(1):99-108. [CrossRef] | [Google Scholar]
- Kim J, Yoon H, Horie T. Microrna-33 regulates apoe lipidation and amyloid-β metabolism in the brain. J Neurosci. 2015;35(44):14717-26. [CrossRef] | [Google Scholar]
- Williams T, Borchelt DR, Chakrabarty P. Therapeutic approaches targeting Apolipoprotein e function in Alzheimer’s disease. Mol Neurodegener. 2020;15(1):1-19. [CrossRef] | [Google Scholar]
- Elali A, Rivest S. The role of ABCB1 and ABCA1 in beta-amyloid clearance at the neurovascular unit in Alzheimer’s disease. Front Physiol. 2013 [CrossRef] | [Google Scholar]
- Kuang H, Tan CY, Tian HZ. Exploring the bi-directional relationship between autophagy and Alzheimer’s disease. CNS Neurosci Ther. 2019 [CrossRef] | [Google Scholar]
- Laplante M, Sabatini DM. MTOR signaling in growth control and disease. Cell. 2012;149(2):274-93. [CrossRef] | [Google Scholar]
- Zhao Y, Zhang Y, Zhang J, Zhang X, Yang G. Molecular Mechanism of Autophagy: Its Role in the Therapy of Alzheimer’s Disease. Curr Neuropharmacol. 2020;18(8):720-39. [CrossRef] | [Google Scholar]
- Raulin AC, Doss SV, Trottier ZA, Ikezu TC, Bu G, Liu CC, et al. ApoE in Alzheimer’s disease: pathophysiology and therapeutic strategies. Mol Neurodegener. 2022;17(1):1-26. [CrossRef] | [Google Scholar]
- Spilman P, Podlutskaya N, Hart MJ. Inhibition of mTOR by rapamycin abolishes cognitive deficits and reduces amyloid-β levels in a mouse model of alzheimer’s disease. PLoS One. 2010;5(4) [CrossRef] | [Google Scholar]
- Mueed Z, Tandon P, Maurya SK, Deval R, Kamal MA, Poddar NK, et al. Tau and mTOR: The hotspots for multifarious diseases in Alzheimer’s development. Front Neurosci. 2019:13 [CrossRef] | [Google Scholar]
- Jovanovic-Tucovic M, Harhaji-Trajkovic L, Dulovic M. AMP-activated protein kinase inhibits MPP+-induced oxidative stress and apoptotic death of SH-SY5Y cells through sequential stimulation of Akt and autophagy. Eur J Pharmacol. 2019:863 [CrossRef] | [Google Scholar]
- Giacoppo S, Pollastro F, Grassi G, Bramanti P, Mazzon E. Target regulation of PI3K/Akt/mTOR pathway by cannabidiol in treatment of experimental multiple sclerosis. Fitoterapia. 2017;116:77-84. [CrossRef] | [Google Scholar]
- Ivanov VN, Grabham PW, Wu CC, Hei TK. Inhibition of autophagic flux differently modulates cannabidiol-induced death in 2D and 3D glioblastoma cell cultures. Sci Rep. 2020;10(1):1-17. [CrossRef] | [Google Scholar]
- Zou S, Kumar U. Cannabinoid receptors and the endocannabinoid system: Signaling and function in the central nervous system. Int J Mol Sci. 2018;19(3) [CrossRef] | [Google Scholar]