ABSTRACT
Background
Tridaxprocumbens is an herbal plant that has been used for many centuries. It has shown promise in treating various medical ailments, including skin infections, burns, and wounds. The plant has several active ingredients, such as triterpenes, ursolic acid, quercetin, and tridaxin, all of which have potent anti-inflammatory, antibacterial, antioxidant, and wound-healing qualities.
Materials and Methods
Lipid-based nanocarriers called ethosomes have drawn much interest in studying six formulations with varying amounts of ethanol and lecithin created in the study. Numerous criteria, including globule size, shape, zeta potential, entrapment efficiency, and in vitro drug release, were evaluated in detail for the ethosomal formulations.
Results
The study’s findings showed that every formulation had spherical and unilamellar characteristics, with entrapment efficiencies ranging from 57 % to 75 %, globule sizes between 122.6 to 910.3 nm, and zeta potentials between -25.06 to -52 mV. The invitro release tests demonstrated the medication’s consistent, long-term release from ethosomal vesicles.
Conclusion
The results of further evaluation studies indicate that the F5 ethosomal formulation, which contains quercetin as an active ingredient, had the highest levels of entrapment efficiency. The release kinetics were consistent with a Fickian zero-order drug release and diffusion pattern, showing both penetration and prolonged drug action.
INTRODUCTION
Ethosomes, a lipid-based vesicular carrier system with particular properties, have been accepted as valuable tools in transdermal medication administration.1 The nanosized vesicles comprise phospholipids, ethanol, and water, and their distinctive composition facilitates the augmented penetration of bioactive chemicals over the skin. Ethosomes have demonstrated significant promise in enhancing the solubility,2 stability,3 and dermal permeation of pharmaceuticals4 and bioactive compounds.5 Formulating those-loaded extracts of Tridax procumbens entails the integration of the plant’s bioactive constituents into ethosomes, resulting in the development of an innovative delivery mechanism.6 This strategy aims to address the constraints commonly observed in the traditional administration of bioactive chemicals derived from Tridax procumbens, including inadequate solubility and restricted skin permeability.7 This study’s primary aim is optimizing the formulation to enhance the encapsulation efficiency of bioactive compounds derived from Tridax procumbens. Additionally, the research seeks to ensure the stability of these compounds and evaluate their release kinetics.
MATERIALS AND METHODS
Collection, Processing and Storage of Leaves
The methodology employed for collecting Tridax procumbens entailed systematically harvesting the plant within the premises of the Sri Balaji Vidyapeeth University campus, Puducherry, India. The complete plant underwent an initial cleaning process with thorough washing. Following this, the leaves were meticulously detached from the other parts of the plant to facilitate their preparation for the subsequent drying procedure. Subsequently, the leaves were exposed to natural sunshine for around five days until they achieved total desiccation. After the leaves had been sufficiently dried, they were subjected to additional processing. The substance underwent a meticulous grinding process with a grinder, resulting in a uniformly fine powder with a constant texture. In order to attain consistency, the powdered leaves underwent a process of size separation using a mesh with a designated number 60.8 The resultant homogeneous powder was afterwards appropriately preserved in a plastic receptacle.
Extraction of Crude Leaves of Tridax procumbens
The methodology employed in preparing the hydroalcoholic extract derived from Tridax procumbens leaves power encompassed a series of sequential procedures.9 The hydroalcoholic solvent was made by combining 150 mL of ethanol and 150 mL of distilled water in a 1:1 ratio. Subsequently, the powdered leaves and the solvent were combined in a proportion of 1:3, yielding a composite comprising 100 g of powdered material and 300 mL of solvent. In order to mitigate the risk of contamination, the conical flask was tightly sealed using aluminium foil and left undisturbed for a short duration. Subsequently, the hermetically sealed flask was positioned within an orbital shaker and exposed to a process of maceration lasting for five days, during which the shaker was set to operate at a speed ranging from 80 to 90 rpm. After the designated maceration duration, the substance was extracted from the shaker and filtrated using a muslin cloth to isolate the liquid filtrate from solid remnants. Following that, the filtrate was subjected to mild heating, utilizing a water bath, until it underwent solidification, forming a compact mass or residue. The residual substance was meticulously removed and afterwards preserved for subsequent examination. The hydroalcoholic extract of Tridax procumbens was synthesized to facilitate its utilization in various analyses.
Phytochemical Analysis
A qualitative phytochemical screening was conducted on the extract to ascertain the presence of specific chemical components.10 The screening process followed established protocols, as a series of tests were performed to identify the presence of the following compounds: Steroids, Tannin, Saponin, Anthocyanin, Coumarins, Emodins, Alkaloids, Proteins, Amino acids, Diterpenes, Phytosterol, Phenol, Phlobatannins, Leucoanthocyanin, Cardiac glycosides, and Flavonoids (Table 1).
Sl. No. | Experiment | Observation | Inference |
---|---|---|---|
1 | Steroid test | Upper red layer and yellow fluorescence formed. | Presence of steroids. |
3 | Saponin test | Formation of stable foam. | Presence of saponins. |
4 | Anthocyanin test | No blue violet is formed. | Absence of anthocyanins. |
5 | Coumarin test | Yellow colour formed. | Presence of coumarin. |
6 | Emodins test | No characteristic change Occurs. | Absence of emodins. |
7 | Alkaloid test Wagner test | Reddish brown ppt formed Yellow coloured ppt formed. | Presence of alkaloids Presence of alkaloids. |
8 | Protein test | No characteristic changes Occur. | Absence of protein. |
9 | Amino acid test | Blue colour formed. | Presence of amino acid. |
10 | Diterpenes test (Copper acetate test) | Emerald green colour formed. | Presence of diterpenes. |
11 | Phytosterol test | Green red colour formed. | Presence of phytosterol. |
12 | Phenol test | Bluish black colour formed. | Presence of phenol. |
13 | Glycoside test | Brown colour ring not Formed. | Absence of glycosides. |
14 | Flavonoid test | ||
15 | Alkaline reagent test | Intense yellow colour formed. | Presence of flavonoids. |
Quantification of Quercetin
UV spectroscopy, a method for assessing quercetin in the samples, is considered convenient and precise.11 In order to commence the analysis, a standard solution of quercetin is created by dissolving a predetermined quantity of quercetin in a suitable solvent, ethanol. This process yields a stock solution with a well-defined concentration, generally established at 1 mg/mL. In order to construct a calibration curve, a sequence of quercetin standard solutions with different concentrations is produced by diluting the stock solution using an identical solvent. The concentrations often employed for these standards typically span a range of 5-25 μg/mL. In order to perform sample analysis, 1 mg of sample was made into solution using 10 ml of ethanol. The spectrophotometer was subsequently prepared for measurement by adjusting the wavelength to the absorption peak of quercetin, which is commonly observed at approximately 369 nm. Before measurements were conducted, the spectrophotometer was calibrated with ethanol, which was utilized for both the standard and sample solutions. The absorbance of each standard solution and the sample were measured at the designated wavelength using cuvettes.
Preparation of Tridax Leaf Extract Loaded Ethosomes
A total of six distinct ethosomal formulations were generated by manipulating lecithin and ethanol concentrations and volumes while keeping the concentration and volume of Tridax extract, which contains the bioactive component quercetin (Table 2). The methodology employed for producing ethosomes was based on the procedure outlined by Fitrya et al. 2021.12 The first step involved the placement of lecithin into a beaker, followed by its dissolution in ethanol. The ethanolic solution was subsequently combined with ethanol containing the Tridax extract using a high-speed homogenizer at 6000 rpm.
Sl. No. | Formulation code | Lecithin (mg) | Ethanol (mL) | Tridax extract (mg) |
---|---|---|---|---|
1 | F1 | 100 | 40 | 400 |
2 | F2 | 200 | 40 | 400 |
3 | F3 | 300 | 40 | 400 |
4 | F4 | 400 | 40 | 400 |
5 | F5 | 200 | 60 | 400 |
6 | F6 | 400 | 60 | 400 |
In order to mitigate the evaporation of ethanol throughout the procedure, the beaker was securely sealed using either parafilm or aluminium foil. Colloidal ethosomal suspensions were prepared by gradually introducing distilled water into the mixture under constant stirring. The ultimate suspension was then kept at ambient temperature for 30 min while subjected to continuous agitation. The ethosomes generated during this procedure underwent a series of assessments to assess their attributes, encompassing morphology, globular dimensions, entrapment efficacy, zeta potential, and in vitro drug liberation, confirming their appropriateness for particular applications or investigations.
Evaluation of Tridax Extract Loaded Ethosomes
Assessment of Vesicle Shape
The shape was determined by employing an optical microscope13 connected to Dewinter microscopic software, namely the pro version 4.1. A minute quantity of autosomal material was deposited onto a pristine glass slide and subjected to examination using a 100x magnification lens.
Determination of Globule size and Zeta Potential
The globule size and charge of the ethosomes were assessed using laser droplet anemometry14 with the Zetasizer version 7.1. The ethosomes were diluted using distilled water, and afterwards, the resulting samples were introduced into an electrophoretic cell, wherein a potential of 150 mV was applied.
Investigation of in vitro drug release
The investigation involved the utilization of a modified Franz diffusion cell to conduct in vitro release tests on ethosomes loaded with Tridax leaf extract.15 The vesicle suspension was applied to a dialysis membrane, and the donor compartment was securely clamped over it using strings. Approximately 1.5 mL of the sample was extracted at predefined intervals spanning 24 hr. The receptor compartment was replenished with fresh medium following each withdrawal to maintain sink conditions. The concentration of the medication that was released was determined through the utilization of UV spectroscopy at a wavelength of 369 nm.
Analysis of release kinetics
Several kinetic models were utilized to examine the data on in vitro release kinetics.16 The zero-order rate equation is employed to characterize systems in which the rate of medication release remains constant regardless of its concentration. The initial-order equation characterizes the liberation process in systems where the release rate is contingent upon the concentration. Higuchi’s model, founded on the principles of Fickian diffusion, delineated the liberation of pharmaceutical substances from matrices that exhibit insolubility as a process contingent upon the square root of time.
RESULTS
The outcomes of a phytochemical examination of Tridax procumbens leaf extract are presented in Table 1. The hydro-alcoholic extract has been found to contain many active metabolites, such as steroids, tannins, saponins, coumarin, alkaloids, amino acids, diterpenes, phytosterol, phenol, and flavonoids. The extensive examination of phytochemicals included in this profile highlights their potential for diverse medical and therapeutic uses.
The research also reveals the notable absence of specific phyto-constituents, including anthocyanins, emodins, proteins, and glycosides, in the extract. Quercetin was estimated quantitatively and found to be 4.82% per 1 mg of Tridax procumbens Linn. ethanolic extract.
The morphological study of the ethosomal formulations was performed utilizing optical microscopy coupled with a digital camera. The shape of all six formulations was consistently observed to consist of spherical and unilamellar vesicles, as depicted in Figure 1. As quantified in nanometers (nm), the vesicles’ globular dimensions displayed variability across various formulations. Formulation 1 exhibited the highest globular size, measuring 910.3±12.8 nm, whilst Formulation 5 displayed the smallest globular size of 122.6±5.02 nm (Figure 2). Interestingly, there was no discernable trend in the globular size relating to the changes in lecithin and ethanol volume and concentration, contrary to several researchers claiming that vesicle size rises with greater lecithin concentrations.

Figure 1:
Optical microscopic image of Ethosome loaded Tridax extract.
The Zetasizer was utilized to evaluate the zeta potential of the formed ethosomes. The assessment of colloidal dispersion stability heavily relies on determining zeta potential. Colloidal globules generally exhibit stability when the zeta potential is within the ±20 to ±40 mV ranges. This phenomenon is mainly ascribed to the electrostatic repulsion between the particles. In the present investigation, it was observed that Formulation 5 displayed the most diminished zeta potential measurement of -25.02 mV (Figure 2), whereas Formulation 3 presented the highest value of -52.64 mV.

Figure 2:
Globule size (Upper) and Zeta potential (Lower) of Ethosome loaded Tridax extract.
The potential for quercetin entrapment was expected to increase as the amount of lecithin was augmented. Hydrophilic medications are generally encapsulated within the aqueous core located within the lipid layer, whereas lipophilic pharmaceuticals are sequestered within the lipid lamella. The encapsulation process is contingent upon the size of the enclosed aqueous core, whereas the entrapment of lipophilic medicines is predicated on the number of lipid bilayers involved.
Out of the several formulations that were examined, it was observed that Formulation 4 demonstrated the best entrapment efficiency, measuring at 75.3 ± 0.67%. This result suggests that Formulation 4 efficiently encapsulated quercetin. On the other hand, it is noteworthy that Formulation 6 exhibited the lowest entrapment effectiveness, as seen by the encapsulation of just 57.2 ± 1.82 % of quercetin. This finding implies a clear correlation between the rise in lecithin concentration and volume and the improvement in quercetin entrapment. Nevertheless, it is essential to highlight that an increase in the concentration and volume of ethanol resulted in a decrease in the trapping of quercetin. This finding suggests that ethanol concentration is a critical factor in regulating the entrapment efficiency of quercetin within ethosomes.
The evidence indicates that the augmentation of ethanol concentration results in an amplified absorption of drugs into the skin. Moreover, the drug diffusion process is enhanced when the size of the vesicle diminishes, mainly attributed to the greater surface area that becomes accessible for diffusion. Measurements were conducted at designated time intervals spanning 12 hr to evaluate the in vitro release of drugs from ethosomes. The experimental findings revealed that all formulations (F1-F6) demonstrated a prompt release of the medication, with a maximum release of 34% achieved within the initial 5 min period (Figure 3). The rapid initial release of the drug is ascribed to the presence of a compact lipid matrix, predominantly composed of the biodegradable lipid lecithin that encapsulates the active component. This finding suggests that all formulations can continuously release drugs over an extended period. Formulation 2 demonstrated the highest drug release rate at the 12 hr mark, achieving a percentage of 90.35 %. Conversely, Formulation 5 exhibited the lowest drug release rate, with a recorded value of 70.89 % at 12 hr (Figure 3). The pliable and malleable characteristics of ethosomes enable enhanced permeation into the underlying layers of the skin. Ethanol, due to its aliphatic chain structure, can disturb the stratum corneum by removing lipids. Including a lipid bilayer within those structures, which closely mimics the structural composition of the skin, serves to augment the process of transdermal drug diffusion. Lipids can permeate the skin via the follicular transport channel, facilitating diffusion, particularly when the lecithin content is elevated. Formulation F5 has notable characteristics in terms of its prolonged drug release compared to alternative formulations.
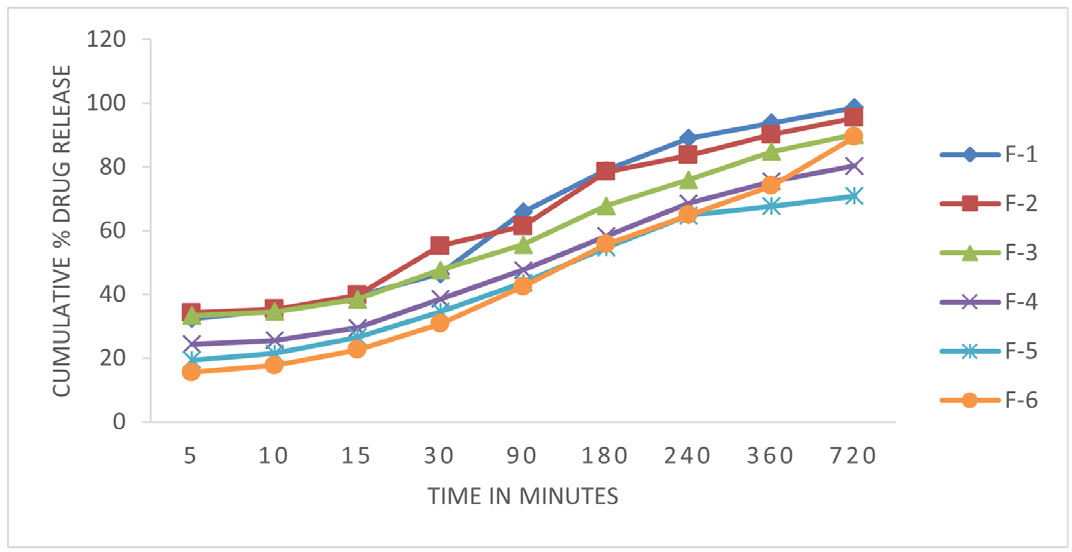
Figure 3:
In vitro release studies of Ethosome loaded Tridax extract.
The release data from in vitro experiments was analyzed utilizing a range of kinetic equations, including zero order, first order, Higuchi, and Korsmeyer-Peppas models. The release constant was derived by analyzing the slope of the relevant Figure 4, and the regression coefficient (r2) was computed. The zero-order equation was the most suitable model for describing the in vitro release kinetics of ethosomal preparations (formulations F1-F6).
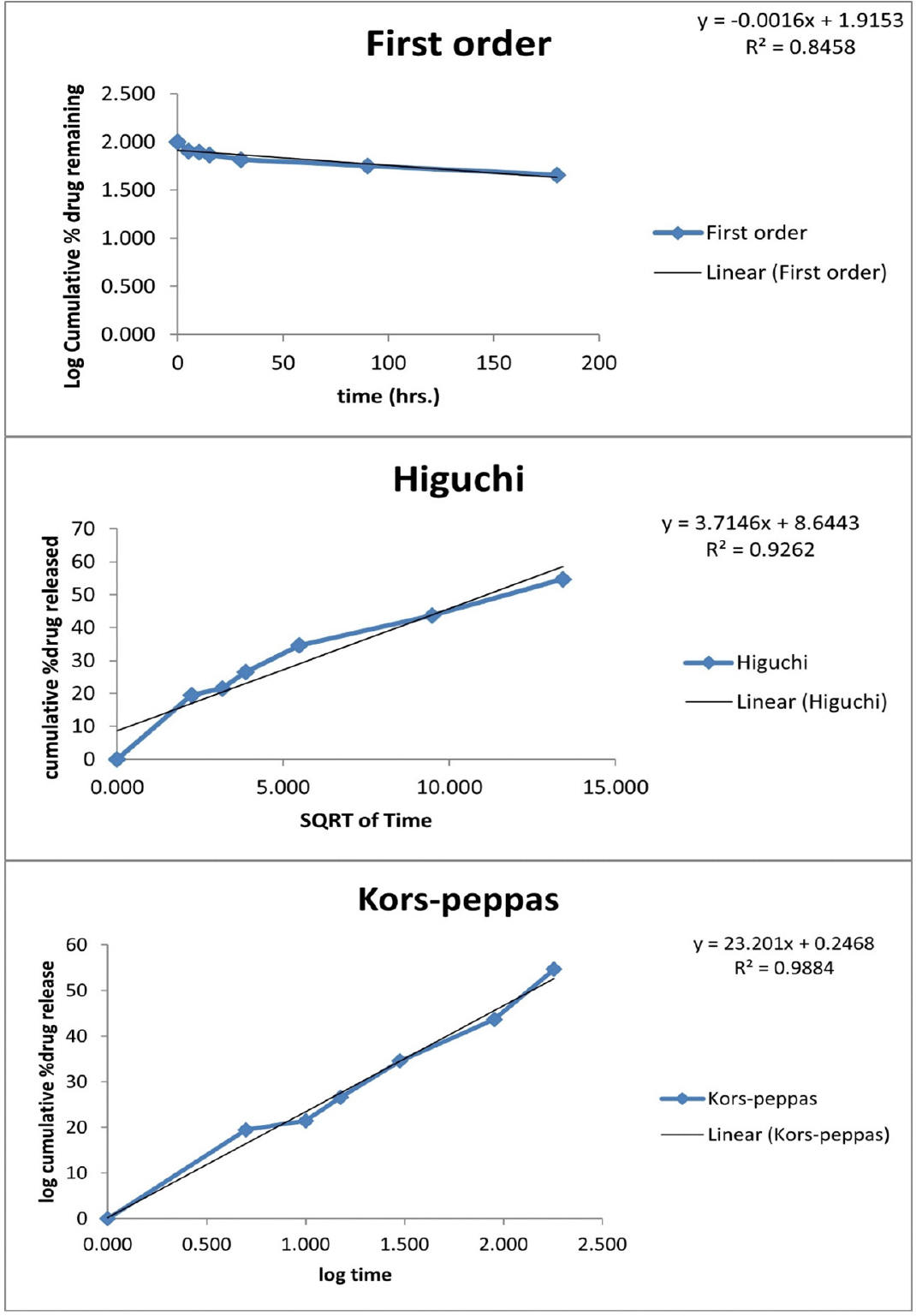
Figure 4:
Release kinetics of Ethosome loaded Tridax extract.
The plots demonstrated a robust linear relationship, as evidenced by the high coefficient of determination (r2) values ranging from 0.7172 to 0.766. Subsequently, the initial-order model exhibited coefficients of determination (r2) ranging from 0.7866 to 0.92, whereas the Higuchi equation demonstrated coefficients of determination (r2) ranging from 0.8459 to 0.8905. The results of this study suggest that the rate at which the drug is released is not significantly affected by the concentration of the drug. The value of the release exponent (n) was determined to be 0.59 (Figure 4), indicating the presence of anomalous diffusion. The research of release kinetics is of utmost importance in conducting comparative analysis, as it allows for examining release parameters about bioavailability. Additionally, it enables the investigation of the influence of formulation factors on the optimization and regulation of drug release from ethosomes. Additional examination of zero-order and first-order kinetic models demonstrated a linear correlation, with greater r2 values seen for the zero-order model, suggesting that ethosomes demonstrated drug release controlled by zero-order kinetics from their ethanolic carrier. According to Higuchi’s model, the drug release mechanism is characterized by diffusion. The Korsmeyer-Peppas model was utilized to investigate the drug release behaviour, and it was observed that the release mechanism exhibited Fickian anomalous diffusion characteristics. The ‘n’ values employed in the model ranged from 0.2 to 0.5.
DISCUSSION
Observing consistent morphology in the ethosomal vesicles across all formulations is a significant discovery. The findings suggest that alterations in the content and volume of lecithin and ethanol, essential constituents of ethosomal formulations, had negligible effects on the morphological properties of the vesicles.17 The results indicate a certain level of stability observed in the vesicle structure when varying amounts of lecithin and ethanol are examined. It is essential to acknowledge that our study did not detect any significant alterations in vesicle morphology about ethanol concentration. However, previous studies have suggested that ethanol can affect the thinning of the outer membrane of vesicles. This highlights the intricate nature of ethosomal formulations and emphasizes the necessity of doing comprehensive research to understand the various aspects that influence their properties.18 In general, the findings of our study indicate that ethosomal formulations exhibit a consistent spherical and unilamellar shape. These formulations can serve as a stable platform for drug delivery. Moreover, our results indicate that fluctuations in lecithin and ethanol concentrations have negligible effects on the structural integrity of these formulations.
Our formulations’ wide range of globular shapes underscores the intricate nature of ethosomal vesicles and their susceptibility to different formulation parameters. Although prior studies have suggested a positive correlation between lecithin concentrations and vesicle size, our results did not align with this pattern. This implies that the behaviour of ethosomal vesicles may be altered by factors other than lecithin concentration, such as ethanol.19 The lamellarity of ethosomal vesicles, which spans from the surface to the centre, is a crucial factor influencing their performance. Ethanol, a crucial constituent in ethosomal formulations, augments the solubility of both lipophilic and hydrophilic compounds.20 Higher entrapment effectiveness at higher ethanol concentrations may be attributed to the improved solubility.
The observed relationship between ethanol concentration and vesicle size deviates from the established concept of vesicle size behaviour. The decrease in size observed as ethanol concentration increases can be ascribed to either the solubilization of lipids in ethanol or the creation of a hydrocarbon phase with interpenetrating characteristics. This phenomenon is expected to result in a reduction in the size of vesicles. The zeta potential is a crucial parameter that acts as a fundamental indicator of the stability of colloidal systems.21 The observed phenomenon manifests the electrostatic forces operative among particles inside a dispersion. The formulation’s stability is attributed to preventing particle aggregation by electrostatic repulsion when the zeta potential falls within the ±20 to ±50 mV range. The trapping of active chemicals within ethosomes is subject to various conditions, such as the medication’s properties and the formulation’s composition. The entrapment of quercetin in the aqueous core of ethosomes was facilitated by its hydrophilic properties, whereas lipophilic medicines were retained by the lipid bilayers.22 This elucidates the correlation between the concentration of lecithin and the trapping of quercetin.
Notably, the augmentation of ethanol concentration and volume exhibited a decelerating impact on the trapping of quercetin. This implies that the presence of ethanol, a substance observed to impact the arrangement of lipid bilayers, can affect the process of encapsulating specific molecules. Hence, optimizing ethanol content is crucial in attaining the optimum entrapment efficiency in those formulations.
The research findings illustrate that the concentration of ethanol and the size of vesicles are significant factors influencing the diffusion of drugs into the skin. The rapid initial release of the drug is ascribed to the presence of a condensed lipid matrix. In contrast, utilizing a biodegradable lipid lecithin matrix accomplishes the sustained release of the drug. The flexible shape of ethosomes facilitates the enhanced deep penetration of drugs into the epidermis.23 The lipid structure of the skin is disrupted by ethanol, hence facilitating the diffusion of drugs. The improved diffusion of drugs can be attributed to the lipid bilayer in ethosomes, which closely resembles the skin’s structure, as well as the utilization of the follicular transport pathway. Formulation F5 demonstrates superior drug release characteristics compared to other formulations, indicating its potential as a viable choice for controlled drug delivery.
The study applied multiple kinetic models to explore the drug release mechanism and kinetics from ethosomes. A consistent drug release rate characterizes zero-order kinetics, whereas the first-order model exhibits a linear correlation, albeit with diminished control over the release process.24 Higuchi’s model proposed a release mechanism based on diffusion, while the Korsmeyer-Peppas model subsequently validated the occurrence of anomalous diffusion. The discoveries above are essential in enhancing medication release and gaining insights into the pharmacological characteristics of medicines encapsulated within ethosomes.
CONCLUSION
In conclusion, our year-long study developed and tested Tridax leaf extract-loaded ethosomal formulations. Tridax leaf extract-loaded ethosomes were prepared using heat homogenization with lecithin and ethanol, yielding six formulations with varied ratios. Globule size, shape, zeta potential, entrapment efficiency, and in vitro drug release were carefully investigated. In vitro release kinetics validated these formulations’ penetration and prolonged action using zero-order drug release and Fickian diffusion. These findings suggest that smaller vesicle-sized ethosomes could deliver Tridax leaf extract containing quercetin, especially in lipomas where drug delivery through phosphatidylcholine surfactants is needed by releasing the drug at various penetration pathways.
Cite this article
Vinayagam V, Palaniraja K, Ravi Y, Jayashankar A, Venugopal V. Innovative Formulation of Tridax procumbens Ethosomes: A Study on Properties and Drug Release Kinetics. J Young Pharm. 2024;16(1):17-23.
ACKNOWLEDGEMENT
This research work was supported by School of Pharmacy, Sri Balaji Vidyapeeth (Deemed to be University) Puducherry, India.
ABBREVIATIONS
UV | Ultra Violet |
---|---|
n | Release exponent |
R2 | Regression |
References
- Murthy . Transdermal drug delivery: approaches and significance. Res Rep Transdermal Drug Deliv. 2012:1 [CrossRef] | [Google Scholar]
- Ingole VV, Mhaske PC, Katade SR. Phytochemistry and pharmacological aspects of Tridax procumbens (L.): A systematic and comprehensive review. Phytomed Plus. 2022;2(1):100199 [CrossRef] | [Google Scholar]
- Kumar AE, Patra CN. Formulation Characterization Optimization of duloxetine loaded ethosome Patch for transdermal Application. Res J Pharm Technol. 2022:5565-70. [CrossRef] | [Google Scholar]
- Hannah L, Aguilar G, Blanchon D. Spatial distribution of the Mexican daisy, Erigeron karvinskianus, in New Zealand under climate change. Climate. 2019;7(2):24 [CrossRef] | [Google Scholar]
- Joel TJ, Shobini J. A plausible antibacterial green synthesized ag-NPs from Tridax procumbens leaf-flower extract. J Pure Appl Microbiol. 2018;12(4):2135-42. [CrossRef] | [Google Scholar]
- Raut S, Koli P, Desai H, Shelake S, Chougule N. An overview of ethosomes as novel vesicular carrier: its principle, preparation and applications. Int J Pharm Sci Rev Res. 2023;79(1) [CrossRef] | [Google Scholar]
- Natsheh H, Vettorato E, Touitou E. Ethosomes for dermal administration of natural active molecules. Curr Pharm Des. 2019;25(21):2338-48. [PubMed] | [CrossRef] | [Google Scholar]
- Majumdar TK, Vasudish CR, Premavalli KS, Bawa AS. Studies on processing and storage stability of Ashgourd-mint leaves juice. J Food Process Preserv. 2009;34:549-56. [CrossRef] | [Google Scholar]
- Devasvaran K, Alallam B, Lim V. Optimisation of the extraction of crude polysaccharides from Clinacanthus nutans leaves for antioxidant applications: content analysis, chemometrics and metabolomics analysis. Ind Crops Prod. 2023;202:117086 [CrossRef] | [Google Scholar]
- Nortjie E, Basitere M, Moyo D, Nyamukamba P. Extraction methods, quantitative and qualitative phytochemical screening of medicinal plants for antimicrobial textiles: a review. Plants (Basel). 2022;11(15):2011 [PubMed] | [CrossRef] | [Google Scholar]
- Shukla DPK. Simultaneous Quantification of quercetin and syringic acid in methanolic Extract of Leucas lavandulifolia by using Validated HPTLC Densitometric Method. SSRN Electron J. 2021 [CrossRef] | [Google Scholar]
- Fitrya F, Fithri NA, Amriani A, Haryati A, Wijaya DP. Preparation and characterization of petai pods extract (Parkia speciosa Hassk.) loaded ethosomes. Sci Technol Indonesia. 2021;6(1):19 [CrossRef] | [Google Scholar]
- Gutierrez-Medina B, Noemi C, Candia H, de Jesús M, Miranda S. Recovering cellular shape and size in the bright field optical microscope. Biophys J. 2017;112(3):581a [CrossRef] | [Google Scholar]
- IPF validates zeta potential analyser measurement results. Membr Technol. 2023;2023(2) [CrossRef] | [Google Scholar]
- Liu X, Liu H, Liu J, He Z, Ding C, Huang G, et al. Preparation of a ligustrazine ethosome patch and its evaluation in vitro and in vivo. Int J Nanomedicine. 2011;6:241-7. [PubMed] | [CrossRef] | [Google Scholar]
- Aulia S, Winarti L, Wicaksono Y. Meloxicam self-nanoemulsifying drug delivery system: formulation and release kinetics analysis. Int J Appl Pharm. 2021:188-93. [CrossRef] | [Google Scholar]
- Abdulbaqi IM, Darwis Y, Khan NA, Assi RA, Khan AA. Ethosomal nanocarriers: the impact of constituents and formulation techniques on ethosomal properties, in vivo studies, and clinical trials. Int J Nanomedicine. 2016;11:2279-304. [PubMed] | [CrossRef] | [Google Scholar]
- Sarhan WM, Sarhan O. Evaluation of ethosomal epigallocatechin-3-gallate gel formulations as an antioxidant and thermogenic agent. Int J Pharm Pharm Sci. 2020:122-30. [CrossRef] | [Google Scholar]
- NC R, Satveek M, HN M, Patil AB. A comparative study of terbinafine ethosomal formulations: A novel approach. J Health Allied Sci Nu. 2013;03:023-9. [CrossRef] | [Google Scholar]
- El-Shenawy AA, Abdelhafez WA, Ismail A, Kassem AA. Formulation and characterization of nanosized ethosomal formulations of antigout model drug (febuxostat) prepared by cold method: in vitro/ex vivo and in vivo assessment. AAPS PharmSciTech. 2019;21(1):31 [PubMed] | [CrossRef] | [Google Scholar]
- Jena J, Mahendrakar S, Sahu D, Sahu B, Patro N, Sahoo BM, et al. Nanovesicle ethosome: A potential carrier of microwave assisted polyherbal extracts as Cosmecueticals. Curr Nanomater. 2021;6(1):31-42. [CrossRef] | [Google Scholar]
- Nasri S, Ebrahimi-Hosseinzadeh B, Rahaie M, Hatamian-Zarmi A, Sahraeian R. Thymoquinone-loaded ethosome with breast cancer potential: optimization, in vitro and biological assessment. J Nanostructure Chem. 2020;10(1):19-31. [CrossRef] | [Google Scholar]
- Dhakar RC. Formulation development and evaluation of ethosome of stavudine. SSRN Electron J. 2021 [CrossRef] | [Google Scholar]
- Hao Y, Song H, Zhou Z, Chen X, Li H, Zhang Y, et al. Promotion or inhibition of extracellular vesicle release: emerging therapeutic opportunities. J Control Release. 2021;340:136-48. [PubMed] | [CrossRef] | [Google Scholar]