ABSTRACT
Background: The main goal of any drug delivery system is to achieve the desired concentration of the drug in blood or tissue, which is therapeutically effective and nontoxic for a prolonged period. Sitagliptin is a dipeptidyl peptidase 4 enzyme inhibitor used in the treatment of non-insulin-dependent diabetes mellitus. The purpose of this study was to design a flexible dosage form that controls the release and provides therapeutic effects with minimum side effects. Materials and Methods: Various batches of pellets were made using the extrusion spheronization technique for obtaining the batch that resulted in a sustained release pattern for Sitagliptin. Results: The pellets showed excellent flow properties due to sphericity thereby influencing the dosage production rate, the small particle that could easily disperse and help in avoiding the dose dumping. The pellets showed 89.10 % release in 12 hr. Conclusion: The study concludes the development of sustained-release pellets by selective excipients with better drug release minimizing the problems.
INTRODUCTION
The oral route of drug administration is often regarded as the most desirable and patient-friendly method of drug administration. The research in orally active candidates is focused to achieve repeatable and effective plasma concentrations in vivo during drug development.1 Many substances are either ineffectively or incompletely absorbed after oral administration (i.e., bioavailability is a problem), or the needed dose frequency is too short to allow once- or twice-daily administration (i.e., pharmacokinetic half-life is a problem).2 During a discovery programme, lead optimization usually overcomes such flaws; but, in many circumstances, it is not possible to locate a viable clinical candidate with the required “perfect” physicochemical and/or pharmacokinetic qualities.3 Controlled release drug delivery has recently become the industry standard in modern pharmaceutical design, and extensive research has been carried out to improve medicinal product effectiveness, reliability, and safety.4 In recent years, there has been an increased effort to develop prolonged release dosage forms, as well as extended release dosage forms, in conjunction with growth and innovation in the field of pharmaceutical technology.5 The prolonged release dose has the advantage of reducing dosing frequency while improving therapeutic efficacy and lowering the occurrence of side effects.6
One of the main goals of dosage form design is to optimise medication distribution in order to achieve some control over the therapeutic impact in the face of unknown variations in the in vivo environment where drug release occurs. Managing drug action through formulation also entails controlling bioavailability to limit drug absorption rates, which is normally performed by optimising drug availability, i.e., striving to achieve a maximal rate and amount of drug absorption.7
Extrusion and spheronization and other methods like fluid bed granulation, centrifugal granulation are generally prefered for pellet preparation. Since 1970, extrusion spheronization has been one of the most prevalent procedures for preparing pellets for the acquisition of modified release systems in the pharmaceutical sector.8 Extrusion spheronization pellets provide multiple benefits, including high sphericity, dense structure, less hygroscopic product, limited particle size distribution, and a plane and even surface.9,10
The number of persons diagnosed with diabetes has risen dramatically during the last two decades all across the world.11 According to the World Health Organization (WHO), there were 135 million diabetes in 1995, with that Figure expected to rise to 300 million by 2025.12 The drug sitagliptin, which is used to decrease blood sugar levels, was chosen for the development of sustained release matrix pellets.13
Ingredients | Values for 0 Level |
---|---|
Sitagliptin | 2.5 g |
Xanthan Gum | 4.0 g |
MCC | 12.5 g |
Lacose Monohydrate | 3.5 g |
Povidone | 1.6 g |
Triethyl Citrate (TEC) | 0.4 g |
Granulating vehicle | 8 mL |
Spheronization Speed | 1000 |
Spheronization time | 10 min |
In this study, we attempt to prepare uncoated matrix pellets using non-expensive polymers. This attempt was made also to achieve the sustained release from developed product and investigate the influence of process parameters.
MATERIALS AND METHODS
Materials
Sitagliptin was obtained as a gift sample from Glenmark Nasik. Xanthan Gum, Avicel pH101, Lactose monohydrate, were procured from Research-Lab Fine Chem Industries, Mumbai. Povidone (Kolidon® K30, BASF) was employed as a secondary binder. A hydroalcoholic solution using 95 % ethanol (50 % V/V) was used a s a granulating vehicle.
Methods
Differential scanning calorimetry (DSC) analysis
With a DSC 204A/G phoenix® instrument (Mettler Toledo DSC 822e, Switzerland) and STARe software version 7.01, the samples were placed in the aluminium cell and heated at a rate of 10°C/ min from 100 to 300°C in an inert atmosphere (Mettler Toledo, Switzerland). Temperatures of peak transition start for sitagliptin, excipients, and physical mixture were measured and compared. All of the tests were carried out in a nitrogen-rich environment.14
Infrared spectroscopic (IR) analysis
The interaction between Sitagliptin and excipients was verified using Fourier transform infrared spectrophotometry (FTIR Spectrometer, BRUKER IFS-55, Switzerland). The KBr technique was used to acquire the IR spectra of Sitagliptin, excipients, and a physical mixture of Sitagliptin and excipients. Between 500 and 4000 cm-1 of spectra was captured.15
Formulation and development of extended-release matrix pellets
Extrusion spheronization was used to make the prolonged release matrix pellets, with over 80% yield, particle size NMT 1.25 mm, and around 90% release at pH 7.2 within 12 hr as essential product quality parameters.16 The drug-containing materials were precisely weighed as specified in the recipe (Table 1) and combined in a high-shear mixer. By gradually introducing the granulating vehicle in small quantities, the powder mix was transformed to a moist mass. Through a perforation of 1mm diameter, the wet mass was extruded to create cylindrical extrudates. The feeder rotated at 45 rpm, while the agitator rotated at 35 rpm. In an Umang spheronizer, the extrudate was spheronized in 25 g loads at 1000 rpm. To make spherical solid units, the extrudates were split into parts. The pellets were dried on trays in a monolayer for 20 min at room temperature (21 ± 2°C).17
Optimization of sustained release pellets
Variable responses from a three-factor Box-Behnken (BBD) design were used to optimise the formulation. The research examined the spheronization time (Min), spheronization speed, and water content18 added (mL) at three different levels i.e., -1 (low), 0 (intermediate) and +1 (high), equidistant on both the sides from middle value. The tests were done three times to ensure that the design was accurate to at least 95%.19 The dependent parameters considered for the study were % friability and % release of the drug. The quadratic models revealed extremely significant model terms (p<0.001).20 The variables of all 13 formulations are represented in Table 2.
Pellets characterization
Size distribution
Sieve analysis was used for studying size distribution of the formed pellets. The pellets (20 g) were placed on a set of sieves (2000, 1200, 1000, 800, and 500 μm). The oscillations on the devices were continued for 20 min and thereafter the material retained on each sieve was collected and weighed. The pellets retained on 800 and passed through 1000 μm were used in further studies.21
Loss on Drying
One (1.0) g of pellets were accurately weighed and the powder was kept in crucible at 105°C for 3 hr and the moisture content was calculated.22
Friability of the pellets
The friability of the pellets was determined using Roche frriabiliator. Approx 10 g pellets subjected to 25 rpm/min for total 4 min as described by official publication for oral solid. The collected pellets were dedusted and weighed to obtained the value of fines generated in the process. The percentage friability was calculated using the formula given in the literature.23
Disintegration test of pellets
The disintegration apparatus modified with a 100 μm mesh net at the base of the tubes was used for evaluation of pellets using deionized water as disintegration media at 37°C. The apparatus was operated until no obvious particles were left on the sieve in each disintegration basket.24
Factor 1 | Factor 2 | Factor 3 | Response 1 | Response 2 | |
---|---|---|---|---|---|
Run | A: Water Content | B: Spheronization Speed | C: Spheronization Time | Friability | Release |
mL | rpm | Min | % | % | |
10 | -1 | -1 | 0 | 0.65 | 89.5 |
5 | 0 | -1 | 1 | 0.42 | 88.5 |
6 | 0 | -1 | -1 | 0.48 | 90.2 |
12 | 1 | -1 | 0 | 0.36 | 82.2 |
8 | -1 | 0 | 1 | 0.72 | 84.4 |
9 | -1 | 0 | -1 | 0.69 | 93.7 |
7 | 0 | 0 | 0 | 0.48 | 82.5 |
1 | 1 | 0 | -1 | 0.55 | 82.4 |
3 | 1 | 0 | 1 | 0.48 | 79.9 |
11 | -1 | 1 | 0 | 0.73 | 88.4 |
2 | 0 | 1 | -1 | 0.64 | 79.2 |
13 | 0 | 1 | 1 | 0.71 | 77.3 |
4 | 1 | 1 | 0 | 0.69 | 80.6 |
Scanning electron microscopy (SEM)
A scanning electron microscope (Jeol) operating at 15 kV was used to examine the pellets’ interior and exterior morphologies. Pellets were sputter coated with metal for around 1 min and then mounted on aluminium studs as entire pellets or after being split in half. Magnification was set to provide a panoramic view of the entire pellet, as well as an imprint of the external and internal structure.25
Dissolution Studies
The dissolution tests were performed on correctly weighed samples (n = 6) containing 50 mg of Sitagliptin in automated dissolution testing equipment (Electrolab) utilising USP apparatus I at 100 rpm in phosphate buffer solution of pH 7.2 at 37°C.26 A multi-cell transport spectrophotometer was used to analyse the samples obtained from the vessels at 267nm (Shimadzu, Japan).
RESULTS
According to preliminary API and excipient compatibility studies, the medication interacts with the chosen excipient almost never. The DSC investigations verified that there is no interaction between the active ingredient and excipient and that the active ingredient melts at 215°C. The FTIR analyses also revealed the excipients’ distinctive peaks and the drug’s fingerprint.
Only Avicel pH 101 has the highest particle size at low density, according pre-formulation study. As a result, grinding and sieving were used to measure the particle size of the Avicel pH 101.
The model was significant and displayed a value of less than 0.005 in the DOE optimization trial. The dependent variable-based optimal model provided design flexibility to create the formulation with the required properties. Pred R-Squared and Adj R-Squared did not differ by more than 0.2. The expected values for friability and drug release as determined by checkpoint analysis are displayed in Table 3. A LOD of less than 2% was applied to each formulation that was created. Consequently, it has a smaller effect on product development.
A high yield percentage with an appropriate bead size of 0.8 to 1 mm is required for the extrusion spheronization process employing a die plate with a diameter of 1 mm to be developed successfully. The manufactured pellets had a Gaussian distribution with a mean particle size and area of 0.93 mm and 0.93 mm, respectively.
When evaluated in phosphate buffer pH 7.4 as the medium for the dissolution investigation, drug release was successful. Even after six hours, the pellets were intact and continued to release the medicine gradually via the diffusion mechanism.
DISCUSSION
The combination of xanthan gum and MCC creates an ideal matrix for achieving a drug’s sustained release profile. The Selected polymers from the previous research not revealed any interactions. Despite the low density of Avicel pH 101, combining it with other excipients resulted in dense low-friability pellets appropriate for tablet compression.26 The low friability and dense pellets offer uniformity in drug content thereby decreasing the dose variability. The dense pellets also offer ease in the scale up operations on the high-speed machines. The main goal of the optimization was to reduce medication loss due to friability while increasing release. The difference between “Pred R-Squared” and “Adj R-Squared” was less than 0.2, indicating that they are in reasonable agreement, with a value of larger than 4 indicating adequate precision.23 Formulation was found to be improved after a checkpoint study (Table 3). Based on the interaction of Factor X1 with Factor X2 and X1 with X3, the contour plots propose the design space. There was no interaction between X2 and X3.
Response | Adequate Precision | R 2 | Adjusted R2 | Predicted R2 | SD | %CV | p-Value | |
---|---|---|---|---|---|---|---|---|
Y1 (% Friability) | 10.51 | 0.79 | 0.72 | 0.59 | 0.06 | 11.51 | 0.0020 | |
Y2 (% Release) | 8.60 | 0.74 | 0.66 | 0.46 | 2.92 | 3.46 | 0.0048 | |
Factor | Optimised Level | |||||||
X1 (Water Level) | 0.183 | |||||||
X2 (Spheronization speed) | – 1 | |||||||
X3 (Spheronization time) | – 1 | |||||||
Response | Expected | Observed | ||||||
Y1 (% friability) | 0.46 | 0.44 | ||||||
Y2 (% Release) | 88.86 | 89.10 |
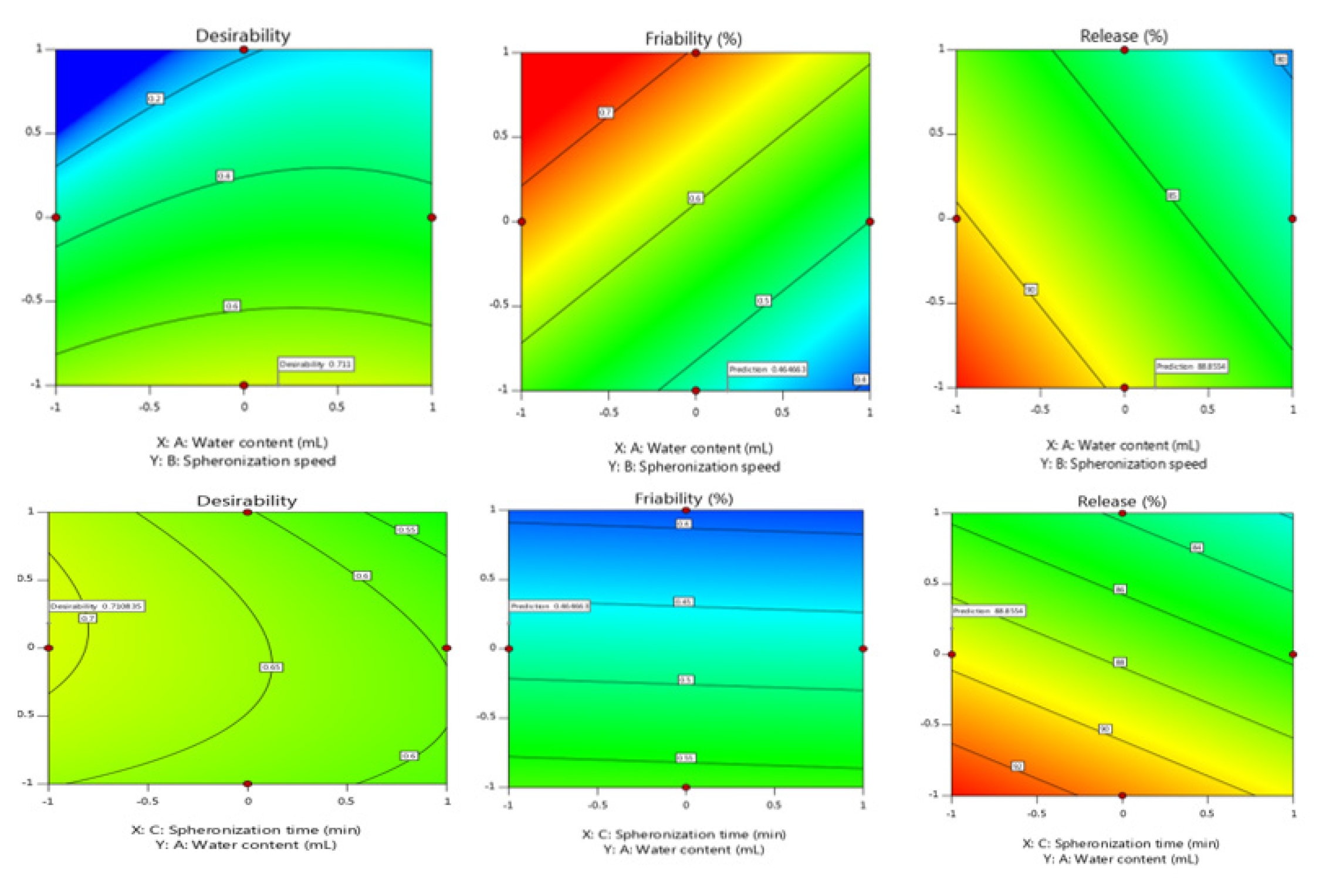
Figure 1.
Contour plot for predicting influence of independent parameters on Dependent parameters.
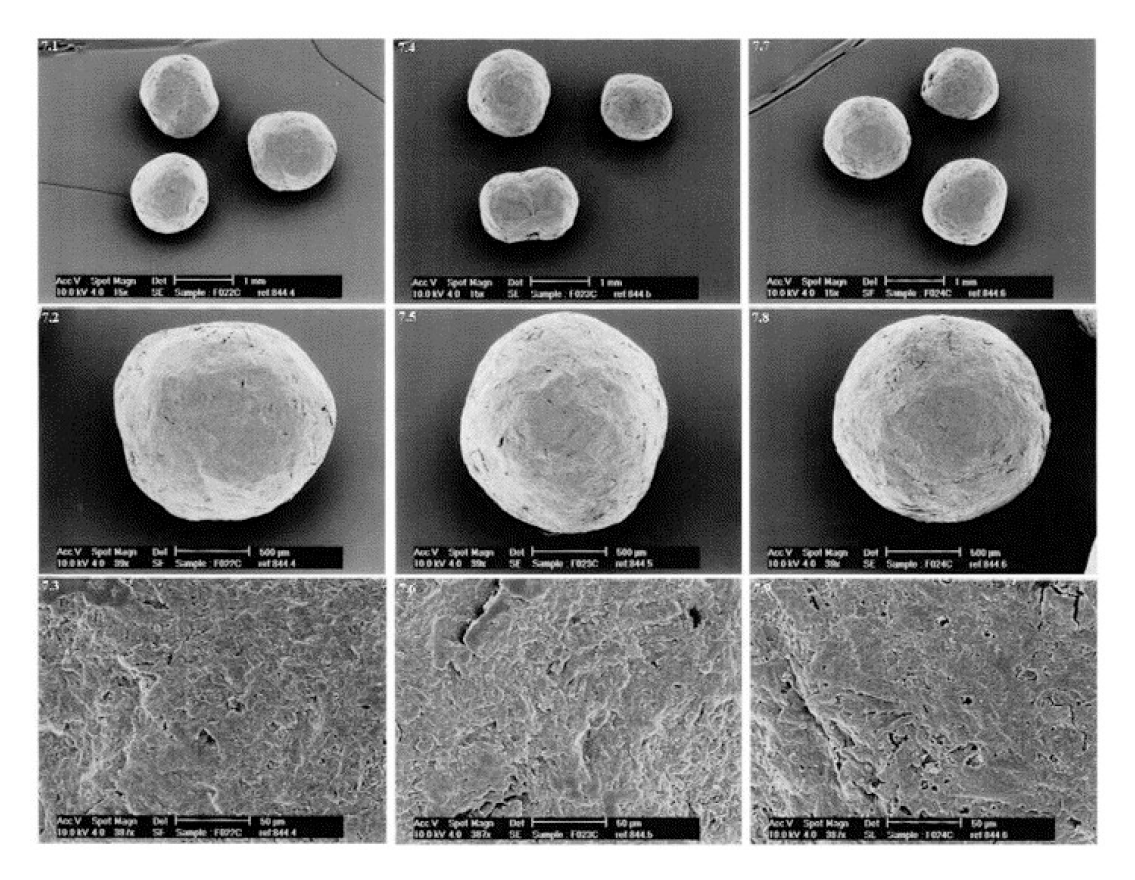
Figure 2.
SEM images of the optimised Pellets of Sitagliptin.
(Figure 1). Increased xantham gum and water concentrations resulted in bigger particles but lower total yield. Because an optimal moiture content aids effective pellet compression in the presence of additional excipients, the drying duration was optimised to leave a moiture content of 5-8%. The longer the drying time, the more friable the pellets become, making the compression process more complicated and challenging.27
Because the spheronization speed has a significant impact on the morphology of the pellets, it was set to 900 rpm. The initial faster spheronization speed aids in getting enough water to the perimeter, resulting in more spherical pellets.28,29 Triethyl citrate was used to assist plasticize the generated pellets during the initial spheronization process and then keep their shape after drying.30,31 All of the recipes produced pellets that were spherical and homogeneous in shape. The scanning electron micrograph of optimised pellets is shown in Figure 2.
The model drug was quickly released when pellets were exposed to dissolving testing (phosphate buffer pH 7.4 as the medium). The pellets did not appear to enlarge or grow in size during the trial. The fact that the pellets did not disintegrate during dissolving was the most intriguing finding.32 Pellets, on the other hand, were found to be intact after dissolving tests (720 min); the structure’s integrity was visually retained. It is clear that increasing the amount of TEC in the plasticizer (from 10% to 30%) greatly increased film elongation and reduced stress at break point.
Lactose monohydrate in the formulation provided consistency to wet masses for extrusion-spheronization and also conserved the structure of the pellets by counteracting the hydrophilic polymer MCC and xanthan gum’s excessive cohesive and adhesive forces. MCC appeared to swell quickly in the addition of xanthan gum and lactose monohydrate, presumably to assure the creation of a gel-like structure that would allow the model medicine to be released continuously.33
CONCLUSION
The advancement of drug delivery has witnessed a dramatic shift from traditional to new drug delivery systems that fit specific needs, but it has also rendered drug delivery pricey due to the usage of expensive excipients. This study was effective in establishing a long-acting, easily scalable medication delivery system for the treatment of chronic illnesses while minimizing side effects.
Cite this article
Gurjar PN, Bhosale AV. Impact of Selective Polymer on Optimization of Sustained Release Matrix Pellets of Sitagliptin. J Young Pharm. 2023;15(2):308-13.
CONFLICT OF INTEREST
The authors declare that there is no conflict of interest.
References
- Ankit B, Rathore R, Tanwar Y, Gupta S, Bhaduka G. Oral sustained release dosage form: An opportunity to prolong the release of drug. Int J Advanced Res Pharm and Bio Sci. 2013;3(1):7-14. [Google Scholar]
- Joshi A, Dashora K. A review-modified release drug delivery system and its significance. Current Research in Biological and Pharmaceutical Sciences. 2014;3(5):1-6. [Google Scholar]
- Miethke M, Pieroni M, Weber T, Bronstrup M, Hammann P, Halby L, et al. Towards the sustainable discovery and development of new antibiotics. Nature Reviews Chemistry. 2021;5(10):726-49. [Google Scholar]
- Patel H, Panchal DR, Patel U, Brahmbhatt T, Suthar M. Matrix type drug delivery system: A review. J Pharm Sci Biosci Res. 2011;1(3):143-51. [Google Scholar]
- Mandhar P, Joshi G. Development of sustained release drug delivery system: A review. Asian Pac J Health Sci. 2015;2(1):179-85. [Google Scholar]
- Leppik IE, Hovinga CA. Extended-release antiepileptic drugs: A comparison of pharmacokinetic parameters relative to original immediate-release formulations. Epilepsia. 2013;54(1):28-35. [Google Scholar]
- Sharma D, Dev D, Prasad D, Hans M. Sustained release drug delivery system with the role of natural polymers: A review. Journal of Drug Delivery and Therapeutics. 2019;9(3-s):913-23. [Google Scholar]
- Jadhav N, Irny P, Mokashi A, Souche P, Paradkar A. Pelletization by extrusion spheronization technique: An excipient review. Drug Delivery Letters. 2012;2(2):132-45. [Google Scholar]
- Manoharan K, Bhaskaran NA, Kumar L. Pellets and techniques of pelletization. Research Journal of Pharmacy and Technology. 2019;12(12):6157-64. [Google Scholar]
- Agrawal S, Joshi K, Gaud R. Formulation development of multi unit particulate system (MUPS) for anti-diabetic drugs. Asian Journal of Pharmaceutical Sciences. 2016;1(11):77-8. [Google Scholar]
- Kallikazaros IE. Diabetes mellitus: A sweet-and-sour disease. Hellenic J Cardiol. 2013;54(2):153-4. [Google Scholar]
- Vlad I, Popa AR. Epidemiology of diabetes mellitus: A current review. Romanian Journal of Diabetes Nutrition and Metabolic Diseases. 2012;19(4):433-40. [Google Scholar]
- Kelleher JF, Madi AM, Gilvary GC, Tian Y, Li S, Almajaan A, et al. Metformin hydrochloride and sitagliptin phosphate fixed-dose combination product prepared using melt granulation continuous processing technology. Aaps Pharmscitech. 2020;21(1):1-14. [Google Scholar]
- Rosasco MA, Bonafede SL, Faudone SN, Segall AI. Compatibility study of tobramycin and pharmaceutical excipients using differential scanning calorimetry, FTIR, DRX, and HPLC. Journal of Thermal Analysis and Calorimetry. 2018;134(3):1929-41. [Google Scholar]
- Mallah MA, Sherazi STH, Bhanger MI, Mahesar SA, Bajeer MA. A rapid Fourier- transform infrared (FTIR) spectroscopic method for direct quantification of paracetamol content in solid pharmaceutical formulations. Spectrochimica Acta Part A: Molecular and Biomolecular Spectroscopy. 2015;141:64-70. [Google Scholar]
- Evers M, Weis D, Antonyuk S, Thommes M. Scale-up of the rounding process in pelletization by extrusion-spheronization. Pharm Dev Technol. 2019;24(8):1014-20. [Google Scholar]
- Barbosa EJ, Ferraz HG. Gellan gum and polyvinylpyrrolidone (PVP) as binding agents in extrusion/spheronization pellet formulations. Acta Pharm. 2019;69(1):99-109. [Google Scholar]
- Agrawal S, Fernandes J, Shaikh F, Patel V. Quality aspects in the development of pelletized dosage forms. Heliyon. 2022:e08956 [Google Scholar]
- Wang J, Kan S, Chen T, Liu J. Application of quality by design (QbD) to formulation and processing of naproxen pellets by extrusion-spheronization. Pharmaceutical development and technology. 2015;20(2):246-56. [Google Scholar]
- H Aodah A, H Fayed M, Alalaiwe A, B Alsulays B, F Aldawsari M, Khafagy ES, et al. Design, optimization, and correlation of in vitro/in vivo disintegration of novel fast orally disintegrating tablet of high dose metformin hydrochloride using moisture activated dry granulation process and quality by design approach. Pharmaceutics. 2020;12(7):598 [Google Scholar]
- Korakianiti ES, Rekkas DM, Dallas PP, Choulis NH. Optimization of the pelletization process in a fluid-bed rotor granulator using experimental design. Aaps Pharmscitech. 2000;1(4):71-5. [Google Scholar]
- Young CR, Crowley M, Dietzsch C, McGinity JW. Physicochemical properties of film-coated melt-extruded pellets. Journal of microencapsulation. 2007;24(1):57-71. [Google Scholar]
- Deshkar S, Pore A. Formulation and Optimization of Immediate Release Pellets of Antiplatelet Drugs Using Design of Experimentation. J Drug Delivery Sci Technol. 2017;7:255-68. [Google Scholar]
- Pezzini BR, Beringhs AO, Ferraz HG, Silva MAS, Stulzer HK, Sonaglio D, et al. Liquisolid Pellets and Liqui-Pellets Are Not Different. AAPS Pharm Sci Tech. 2020;21(2):72 [Google Scholar]
- Haaser M, Karrout Y, Velghe C, Cuppok Y, Gordon K, Pepper M, et al. Application of terahertz pulsed imaging to analyse film coating characteristics of sustained-release coated pellets. International journal of pharmaceutics. 2013;457(2):521-6. [Google Scholar]
- Khalid GM, Usman AG. Application of data-intelligence algorithms for modeling the compaction performance of new pharmaceutical excipients. Future Journal of Pharmaceutical Sciences. 2021;7(1):1-11. [Google Scholar]
- Gohel MC, Parikh RK, Nagori SA, Jena DG. Fabrication of modified release tablet formulation of metoprolol succinate using hydroxypropyl methylcellulose and xanthan gum. Aaps pharmscitech. 2009;10(1):62-8. [Google Scholar]
- Patel S, Kaushal AM, Bansal AK. Compression physics in the formulation development of tablets. Critical Reviews™ in Therapeutic Drug Carrier Systems. 2006;23(1) [Google Scholar]
- Khatri P, Desai D, Shelke N, Minko T. Role of plasticizer in membrane coated extended release oral drug delivery system. Journal of Drug Delivery Science and Technology. 2018;44:231-43. [Google Scholar]
- Al-Hashimi N, Begg N, Alany RG, Hassanin H, Elshaer A. Oral Modified Release Multiple-Unit Particulate Systems: Compressed Pellets, Microparticles and Nanoparticles. Pharmaceutics. 2018;10(4):176 [Google Scholar]
- Cortes H, Caballero-Florán IH, Mendoza-Muńoz N, Escutia-Guadarrama L, Figueroa-González G, Reyes-Hernández OD, et al. Xanthan gum in drug release. Cellular and Molecular Biology. 2020;66(4):199-207. [Google Scholar]
- Arshad MS, Zafar S, Yousef B, Alyassin Y, Ali R, AlAsiri A, et al. A review of emerging technologies enabling improved solid oral dosage form manufacturing and processing. Advanced Drug Delivery Reviews. 2021:113840 [Google Scholar]